Nanoemulgels (NEGs) are novel drug delivery systems that aim to improve the solubility and permeability of drugs across the biological membrane. The nano-sized droplets in the nanoemulsion enhance the permeation of the formulation, along with surfactants and co-surfactants that act as permeation enhancers and can further improve permeability. The hydrogel component of NEG helps to increase the viscosity and spreadability of the formulation, making it ideal for topical application. Moreover, oils that have anti-inflammatory properties, such as eucalyptus oil, emu oil and clove oil, are used as oil phases in the preparation of the nanoemulsion, which shows a synergistic effect with active moiety and enhances its overall therapeutic profile. This leads to the creation of hydrophobic drugs that possess enhanced pharmacokinetic and pharmacodynamic properties, and simultaneously avoid systemic side effects in individuals with external inflammatory disorders. The nanoemulsion’s effective spreadability, ease of application, non-invasive administration, and subsequent ability to achieve patient compliance make it more suitable for topical application in the combat of many inflammatory disorders, such as dermatitis, psoriasis, rheumatoid arthritis, osteoarthritis and so on. Although the large-scale practical application of NEG is limited due to problems regarding its scalability and thermodynamic instability, which arise from the use of high-energy approaches during the production of the nanoemulsion, these can be resolved by the advancement of an alternative nanoemulsification technique.
1. Nanoemulgel, a Potential Carrier System
Nanoemulgels (NEGs) are composed of nanosized emulsions (either oil/water or water/oil) that are integrated into a gel-based system. NEGs convert nanoemulsions into a more stable, non-greasy, and thicker system using a gelling agent, utilized in the preparation of the gel base
[1]. Individually, both the components have their shortcomings; for instance, NEs have low viscosity and spreadability, inadequate skin retention and are non-scalable
[2][3], while gels are not able to incorporate hydrophobic molecule effectively
[4]. The drawbacks of both these systems can be resolved using a novel approach involving NEGs. With these, the lipid-soluble drugs are solubilized in the nanoemulsion’s oil phase, which is then combined with the gel to formulate an NEG. This allows the lipophilic drug to be incorporated into a hydrogel, while increasing the nanoemulsion’s viscosity at the same time
[5]. The nanoemulsion component of the NEG safeguards the drug from hydrolysis and enzymatic degradation. Meanwhile, the gel component stabilizes the system’s thermodynamics by lowering the surface and interfacial tension, and increasing the viscosity and spreadability
[6]. Lipophilic molecules are easily formulated into NEs, which can increase the permeability of drugs so that they can cross the layers of skin; this is because the distribution of nanoemulsion droplets covers a large surface area on the skin. As a result, remarkable enhancements have been made to the pharmacokinetic and pharmacodynamic aspects of lipophilic drugs
[7]. Drawbacks related to traditional topical formulations, such as powders that show hygroscopicity, creams that show instabilities, such as phase inversion or breaking, in their formulations, components in ointments that go rancid, lotions that are sticky in nature, etc., lead to patient incompliance. There are no such drawbacks observed with NEG formulations
[7][8].
In recent years, the development of nanoemulgels has emerged due to their numerous advantages; for example, they allow site specificity, continuous delivery, the release of drugs via a two-step mechanism, first from the nanoemulsion and then from the gel, and a reduction in the dose and dosing frequency. As a result, the total effectiveness of drug administration via the topical route is improved. NEGs do not suffer from drug-leaching or degradation problems regarding niosomes and liposomes, which also leads to their greater drug-loading efficiency. NEGs have the ability to control the release of a drug for an extended period of time, which is desirable for compounds with a short half-life. They create a greater concentration gradient and improve skin permeation due to their superior capacity to adhere to the skin and their greater drug solubilizing ability. Moreover, their noninvasive administration, bypassing gastrointestinal (GI) side effects, makes their application easy and awards them excellent safety and therapeutic profiles, which ultimately leads to better patient compliance
[7].
NEGs, used as a carrier system, are employed for the treatment of a number of inflammatory skin conditions, including acne fungal infections, pimples and psoriasis, along with osteoarthritis and rheumatoid arthritis-related inflammation. The literature reports that, as well as their topical use, nanoemulgels can be used for local and systemic diseases/disorders such as alopecia, periodontitis, and Parkinson’s if administered via ocular, dental, vaginal, and nose-to-brain routes
[9].
2. Nanoemulgels in Topical Delivery for Anti-Inflammatory Drugs
Anti-inflammatory drugs showcase their activity at different stages of the inflammatory cascade (
Figure 1). Glucocorticoids inhibit the phospholipase enzyme that catalyzes the formation of Arachidonic acid, which is the precursor of inflammatory mediators such as prostaglandins and leukotrienes
[10][11]. Non-steroidal anti-inflammatory drugs (NSAIDs) primarily inhibit cyclooxygenase 1 (COX1) and cyclooxygenase 2 (COX2), which, in turn, restrict the formation of prostaglandins, which are responsible for inflammatory reactions
[12]. Meanwhile, many natural anti-inflammatory moieties such as curcumin and quercetin have been reported to have both COX and lipoxygenase (LOX)-inhibiting properties
[13][14]. Topical delivery is an excellent approach for the management of external inflammatory conditions, because by opting for this route, we can not only bypass the first-pass metabolism, but also the gastrointestinal (GI) barriers; these are the gastric emptying time and the intestinal transit time, the enzymes present and pH changes
[15][16]. Topical NSAID application has the benefit of improving the distribution of local drugs to the damaged tissues, while minimizing the risk of systemic side effects, such GI bleeding and peptic ulcers
[17]. Additionally, oils that possess anti-inflammatory properties can be meticulously chosen as the oil phase for the formulation; this can synergistically enhance the desired outcome of the formulation.
The nanosized globules of the chosen NE enhance the potency of the NEG by improving its permeability and diffusibility through the use of appropriate permeation enhancers
[6]. Moreover, a high concentration of water in the gel can sufficiently hydrate the stratum corneum (SC) and cause its tight architecture to leak, allowing the active ingredients to readily penetrate the skin
[18]. Before reaching the skin’s surface, the drug in the NE first traverses from the internal phase (Nanoemulsion) to the external phase (gel), and thus serves as a drug reservoir for topical delivery. When applied topically, oily globules first get liberated from the hydrogel, then permeate deep into the SC of the skin, where they deliver the drug moiety
[19].
Figure 1. Delivery of anti-inflammatory drug along with its mechanism of action.
Mechanistic Approach of Nanoemulgel Delivery via Topical Route
The stratum corneum is regarded as the most effective barrier for regulating the entry and permeation of topically applied drugs. Tight junctions act as a secondary barrier when the subcutaneous (SC) layer is disturbed due to skin diseases or permeation enhancers
[20][21]. The permeation of the drug molecule takes place via three routes, namely. paracellular, transcellular and transappendageal routes (
Figure 2). The primary pathway for permeation through skin is the paracellular route, which involves the drug traversing via the lipid milieu in among the corneocytes. To pass through this pathway, small lipophilic molecules (molecular mass < 500 Dalton) can penetrate the tight lipid junctions between the cells in a circuitous manner
[22]. The transcellular pathway enables the direct passage of the molecule through the SC into the inner layers of the epidermis and possibly to the dermis at the bottom. A molecule moving through the transcellular pathway needs to partition into and diffuse via corneocytes for up to 20 lipid lamellae, which separate each of these cells in order to pass across corneocytes. Thus, to pass through the transcellular pathway, the drug or the carrier should have both hydrophilic and hydrophobic properties. The transcellular route inhibits the permeability of highly lipophilic molecules while allowing tiny hydrophilic or modestly lipophilic compounds (log p between 1–3) to permeate across the epidermis
[22][23]. The transappendageal route involves transporting compounds along hair follicles and their accompanying sebaceous glands, as well as sweat glands. It is comprehensively understood that the appendages’ (hair follicles and associated glands) contribution to epidermal permeation is often minimal because they only make up a small portion of the skin (e.g., only around 0.1% of the forearm skin) for permeation
[24]. NEGs enhance the permeation of the drug as it can utilize all three pathways to transport the drug through the epidermis. The formulation consists of oils, surfactants alone or in combination with the cosurfactant, which acts as an inherent permeation enhancer and gelling agent which aids in increasing the permeability by enhancing the formulation’s adhesion to the skin.
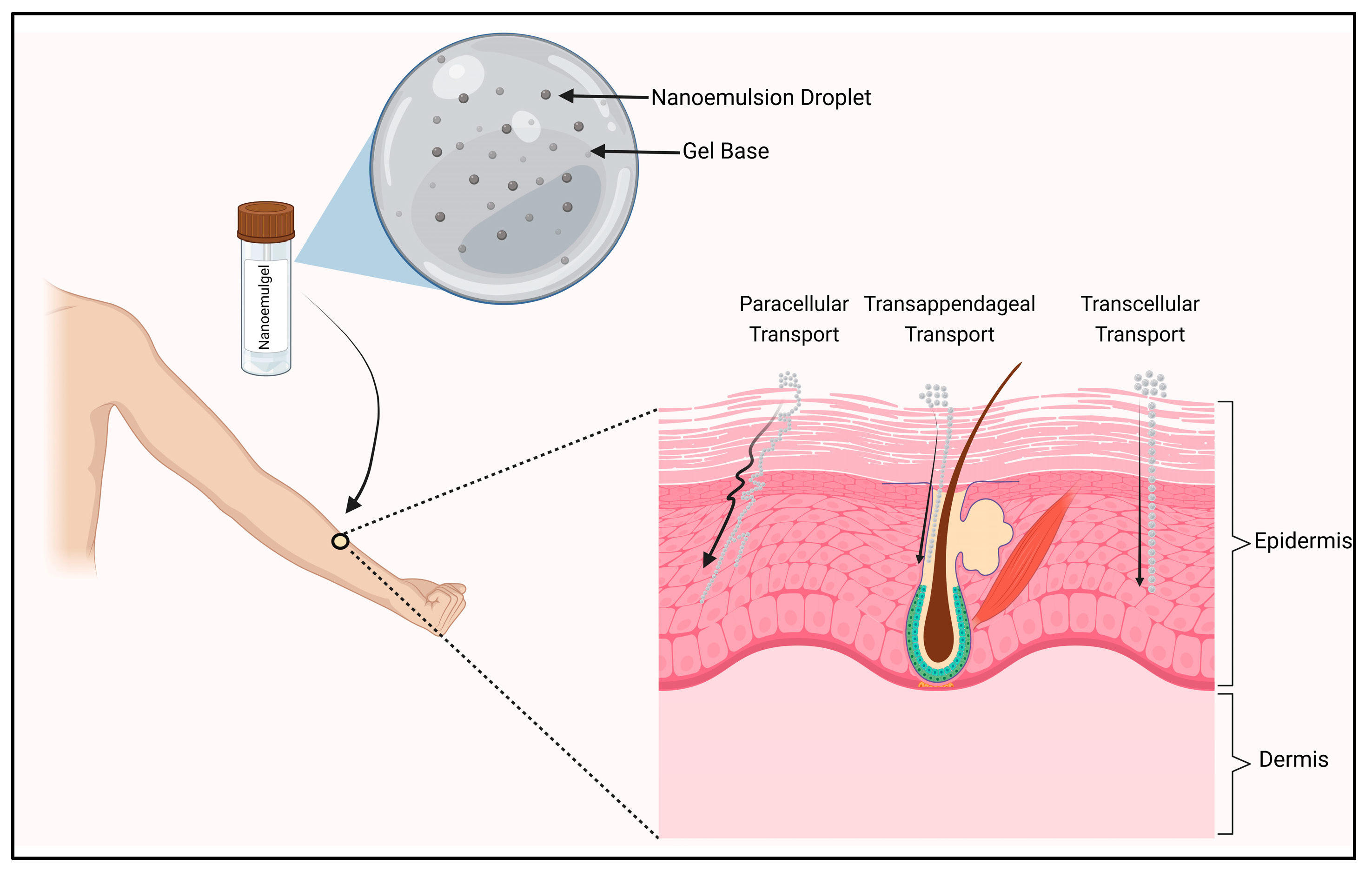
Figure 2. Mechanistic representation of nanoemulgel delivery via skin.
3. Components of Nanoemulgel
Nanoemulgels are basically a two-component system, i.e., NEs are incorporated into the gel base. Nanoemulsions can be a nanosized emulsion of the o/w or w/o type, made up of a blend of oil and water with the help of a suitable surfactant and co-surfactant. The gel base simply consists of polymers with the ability to swell when they encounter liquid by absorption
[1][25]. An overview of the essential components of an NEG is given below (
Figure 3):
Figure 3. Concept diagram for the building blocks required to design an NEG.
3.1. Aqueous Solvents
Aqueous solvents constitute the aqueous phase of the nanoemulsion and the major portion of the gel part of the NEG. The most common aqueous solvents in use are water and alcohol
[7].
3.2. Oil Phase
They serve as a medium for dissolving or dispersing numerous constituents of the formulation. Mostly, lipids of either natural or synthetic origin are used as the oil phase in medicinal and cosmetic applications, except if the oil itself is an active agent. Lipids can range in consistency from liquids to high-molecular-weight solids. A stable emulsion is formed when an oil has a high level of hydrophobicity and shows better emulsification properties, and can simultaneously reduce the solubility of the lipophilic molecules. Therefore, selecting an oil is a crucial preliminary step in the production of an NEG as a novel nano-drug delivery system. The suitable oil should be chosen depending on the formulation specifications for permeability, solubility, stability, and viscosity. Generally, oils in which drugs are more soluble and having good permeation-enhancing properties must be selected. Mineral oils are typically employed for the administration of lipophilic drugs in topical emulsions due to their occlusive properties, which help to stop transepidermal water loss (TEWL) from the skin
[26][27].
3.3. Surfactants
Surfactants that are helpful in the emulsification process, thus named emulsifiers, can be added to stabilize the oil and water phases together
[28]. They work at the interface by lowering the tension between the two phases, which is important for the development of dispersion, and they also aid in reaching the distribution equilibrium at the interface, resulting in the increased thermodynamic and kinetic stability of the emulsion
[9][29]. As a result, they have good solubility in both water and oil. “The portion in which the surfactant is more soluble produces the external phase of the emulsion”, states the Bancroft rule
[30]. For instance, sorbitan fatty acid esters that are oil-soluble favor the production of w/o emulsion, while tweens that are water-soluble facilitate the production of the o/w type of emulsion
[1][31].
3.4. Cosurfactants
Cosurfactants are added to assist surfactants when obtaining nanoemulsion systems at low surfactant concentrations. The cosurfactant and surfactant can cooperate to aid in the emulsification process by disturbing the interfacial layer and facilitating the solubilization of oil
[32]. The co-surfactant and the concentration to be used have to be decided meticulously because they may impact the surfactant’s ability to emulsify the system. Cosurfactants and surfactants whose values are close in the HLB scale cannot produce a stable emulsion in the same way that those produced by non-ionic surfactants with distinct HLB values can. This can be explained, as the higher HLB value surfactants may dissolve in the aqueous phase, while lower HLB value surfactants may dissolve in the non-aqueous phase, allowing for a more effective alliance between the surfactant and the cosurfactant mixture
[33].
3.5. Gelling Agents
Gelling agents are introduced into the formulation to transform the nanoemulsion formulation’s liquid state to gel and address the issues of low viscosity, limited spreadability, and poor retention
[34]. A gelling ingredient, when dispersed in liquid (mostly aqueous phase), results in a weakly cohesive gelling matrix that stabilizes the formulation and promotes the optimum percutaneous drug delivery when utilized in an externally applied formulation
[35]. The gelling agent’s thixotropic property thickens the formulation without changing its volume, aiding in the transition of the liquid to gel. Some gelling agents work by dissolving in the liquid phase and producing a colloidal mixture that may cross-polymerize to construct a minimally cohesive internal structure. Other gelling agents work by virtue of their thixotropic property, i.e., their time-dependent shear-thinning ability, which causes discrete particles to adhere or interlock to withstand strain. Gelling agents have a crucial role in determining the number of parameters, which can include bioadhesion, consistency, drug-release kinetics, extrudability, homogeneity, rheological properties, swelling index, and the spreadability of nanoemulgels. Sodium Carboxy methylcellulose (NaCMC), Carbomers and Hydroxypropyl methylcellulose (HPMC) are the most commonly used gelling agents. Plant-derived polysaccharides that act as stimuli-responsive gelling agents can be used for preparing a gel base that allows the delivery of drugs in a more targeted manner
[36][37]. These kinds of polymers are able to interchange their phases, alter their rigidness, and control and sustain their drug delivery. They can be made to release the drug only if specific conditions (stimuli) are met; this includes in a ROS (Reactive Oxygen Species)-rich environment during inflammation and tissue injury
[38], when there is a lower extracellular pH in inflamed tissue or around a tumorous microenvironment
[39], or when temperature is increased, which acts as an indicator of the inflammatory phase in wound progression
[37][40].
4. Toxicity Concerns
Nanoemulgels contain components such as surfactants and cosurfactants which, are known to cause skin irritation and deterioration, cytotoxicity, and allergic reactions in some patients. Anionic surfactants, such as sodium dodecyl sulfate (SDS), primarily target the SC layer of skin, causing damage to the bilipid layer, the denaturation of protein and skin dehydration, which may lead to dermatitis in sensitive skin. Cationic surfactants cause more severe cytotoxic effects and apoptosis in normal and cancerous cells. Conversely, non-ionic surfactants are relatively less toxic and irritative to skin
[41][42]. Hence, non-ionic surfactants are preferred over ionic surfactants for topical formulations. Cosurfactants such as propylene glycol, when used in an unreasonably high amount, can cause hyperosmolarity, cardiac arrhythmia, lactic acidosis and toxicity of the central nervous system. Fligner et al. reported cardiorespiratory arrest due to a high propylene glycol concentration (1059 mg/dL) in an 8-year-old child during topical silver sulfadiazine therapy
[43]. To minimize these types of toxic effects, these kinds of components should be used in a minimum quantity. When used in the proper amount, numerous researchers have reported that the nanoemulgels did not cause skin irritation or toxicity. Abdallah et al. conducted a skin irritation study by spreading a brucine-loaded nanoemulgel over the shaved skin of rat and covered it with gauze. The rats did not show any signs of oedema or erythema, even after 24 h of application. Similarly, Bhattacharya and Prajapati conducted an acute skin irritation study of a Celecoxib-loaded nanoemulgel on rabbits and reported a 1.6 times lower skin irritation score compared to that of a standard irritant after 7 days of application.
Moreover, the risk of experiencing systemic side effects caused by topically applied nanoemulgels cannot be ignored due to the possibility of the formulation reaching the blood stream by virtue of its enhanced permeation and nano size range, although extensive research needs to be conducted to reach conclusive findings regarding nanoemulgel toxicity. Nano formulations such as polymeric nanoparticles are associated with oxidative stress and cytotoxicity, lipid-based nanoparticles have shown hypersensitivity and cardiopulmonary distress, while tiny rare-earth fluoride nanoparticles can encourage tumor growth via electrical dipole interactions, which are inversely proportion to the size
[44][45].