Cistus ladanifer (rockrose) is a widespread shrub species in the Mediterranean region well known due to its production of labdanum gum, especially in the hot season. Its leaves and branches can be subjected to different extraction and distillation processes to produce various types of extracts. The natural extracts of C. ladanifer have several applications, especially in the perfumery and cosmetics sector. C. ladanifer extracts, in addition to presenting interesting odoriferous properties, are also known for their bioactive properties, such as antioxidant and antimicrobial. The use of this species in animal feed or phytostabilisation of mining areas has also been successfully applied. Furthermore, the lignin and polysaccharides that are the major fractions from Cistus residues can be relevant sources of high-value products in a biorefinery framework.
1. Introduction
Cistus ladanifer L. (crimson-spot rockrose) is a wild perennial shrub species of the
Cistaceae family and the
Cistus genus that is mainly distributed in Mediterranean countries, such as France, Greece, Spain, Portugal, Morocco, Algeria, and Cyprus
[1][2][3][4][5][6].
The
C. ladanifer species includes three subspecies:
ladanifer (…),
africanus (Dans), and
sulcatus (Demoly). The subsp.
ladanifer is mainly distributed in the Iberian Peninsula, France, and northern Africa; the subsp.
sulcatus is endemic to southwestern Portugal along the coastal cliff tops of Costa Vicentina; and the subsp.
africanus is commonly found in northern Africa and also spread in southern Spain
[7][8]. Subsp.
ladanifer has an erect habit, generally with linear–lanceolate and lanceolate leaves
[5][9]; subspecies
sulcatus is a prostrated habit shrub (50 cm) when it grows near the sea or up to 200 cm and erect when protected from the wind, with white flowers and sessile leaves, generally elliptical or oblanceolate, with accentuated nervures in the upper surface
[9]; and subsp.
africanus has leaves with an apparent petiole, lanceolate–elliptic, or oblong to linear—generally lanceolate—with little apparent nerves on the upper side, and other leaves oval or obovate, with well visible nerves on the upper side
[10].
C. ladanifer is a shrub, generally erect, with a reddish-brown stem, hardwood, and sticky bark with striking vivid flowers (
Figure 1). The 40–80 mm long leaves are impregnated by the labdanum, which makes them sticky and with a strong and specific smell
[10]. It is considered a species of rapid growth and development that reproduces easily
[11] by natural seed propagation during winter and autumn
[5][12]. In gardening,
Cistus spp. can be multiplied by stem cuttings and layering
[13]. Vegetative propagation of
C. ladanifer is carried out preferably in September and October using lateral cuttings of 1 to 15 cm in length (without flowering) with five or six pairs of leaves or also 8 to 12 cm long mature wooden stem stakes with a heel or knot
[14]. Micropropagation by germinating seeds of
C. ladanifer and other species of Cistus has also been successfully studied
[15]. The exploitation of wild populations to produce essential oil and labdanum has been the main practice.
Figure 1. Cistus ladanifer L.: (A) whole plant; (B) flower buds; (C) flowers; (D) open cysts.
C. ladanifer occurs in a wide range of altitudes, latitudes, climates, and soil types, but it prefers acidic and siliceous soils
[16][17] and dry areas with high insolation where it can give rise to large and dense populations. It has high-stress tolerance and, consequently, is competitive under various environmental conditions, including poor soils with low organic matter content, low pH, and high concentrations of trace elements, as well as hydric stress and high temperature and solar radiation
[18]. This tolerance may be associated with the activity of different isoenzymes, namely superoxide dismutase (SOD), peroxidase (POD), and catalase (CAT)
[19], which are the major enzymes involved in the detoxification of active oxygen species (AOS)
[20]. An evaluation was made of As, Cu, Pb, and Zn concentrations and activity of the soluble and cell wall ionically bound forms of enzymes SOD, POD, and CAT in leaves of
C. ladanifer. The enzymatic activity of these enzymes varied with populations of
C. ladanifer, as well as with the seasons of the year, with specificity for each trace element and the location of the enzyme in the cells
[19].
C. ladanifer shows an important adaptative mechanism in post-fire plant dynamics, and its recovery after burning is faster when compared with other shrub species (e.g.,
Erica australis and
Calluna vulgaris)
[21].
Cistus species tend to recover by an autosuccession process in areas affected by cutting and burning, being dominant after the first or second year
[22]. Its strong heat resistance suggests that there is a break in seed dormancy by the high temperatures during a fire; e.g.,
Quercus woodlands are occupied by
C. ladanifer after fire
[5]. A study under controlled conditions demonstrated an increase in the germination of seeds preheated at 100 °C when compared to seeds stored at room temperature
[23].
C. ladanifer seeds and plantlets generally show rapid germination and growth
[24].
The fruits are globular lignified capsules (cysts) that can produce 500 to 1000 seeds, and a single plant may produce 250 thousand seeds annually
[25][26]. Mature fruits remain attached to the plant and release the seeds gradually when they open, allowing short-distance dissemination for a long time
[5]. The seeds are small, allowing easy penetration and accumulation in the soil, and have a stiff and impermeable cover important for their longevity; however, they contain few nutrient reserves, which requires a quick start for the photosynthesis process
[25]. The number of valves per fruit (five to twelve) can vary between populations, between plants, and within the same plant. Rockrose is a highly polymorphic plant and the only species of the
Cistaceae family with a variable number of valves per fruit. This variation may be a result of natural selection, phenotypic plasticity, and developmental instability of the plant
[27].
Rockrose flowers are white with a crimson spot on the base of the petals. There are two color varieties:
C. ladanifer var.
albiflorus with white petals and
C. ladanifer var.
maculatus with red stains at the petal base
[28]. The flowers are large (ca. 64 mm in diameter), appear during spring (March–May), and produce abundant pollen and nectar
[5][29]. The size and longevity of the flowers positively influence the incidence of folivores, mainly ants and beetles
[30].
The ecosystem of
C. ladanifer also provides high proliferation of edible mushroom species, some of which are in high demand due to their gastronomic interest
[31]. Rockrose is also considered promising for phytoremediation and revegetation of contaminated soil in semi-arid climates as it is capable of colonizing these soils with a great capacity for tolerance and adaptability to adverse climatic conditions, e.g., droughts and high temperatures
[17][32].
Cistus ladanifer (rock rose) is widespread throughout the Mediterranean basin, where it is a naturally occurring shrub. It is estimated that it extends to circa 2 million hectares in the south and southwest of the Iberian Peninsula
[33], where it is the main shrub species with an important ecological role but also an actor in some challenging situations, such as regarding rural fires that take place in summer in these regions. Rockrose is an example of an underused species without any regular and complete exploitation, and it is currently mainly exploited by the perfumery industry or as an ornamental plant
[14], generally associated with small family businesses or linked to rural organizations. There is still a lack of robust economic exploitation as well as developed value chains.
2. Traditional Products from Rockrose
Rockrose plants have been traditionally used as an important resource for primary health care given their low cost, accessibility, and accumulated ancestral experience. The aerial parts of rockrose are used to produce extracts, obtaining exudates and essential oils with complex composition and pharmacological properties that make interesting further application targeted investigation
[34]. Traditional use of rockrose has contributed to socio-economic development of rural communities
[35]. Both labdanum and essential oil from
C. ladanifer are much appreciated in perfumery, cosmetics, aromatherapy, and food flavors (restricted use), being used as ingredients in about 30% of modern perfumes due to their excellent fixative properties
[36][37]. The
C. ladanifer products are described in detail below.
2.1. Aromatic Extracts
Figure 2 summarizes the major conventional products obtained from rockrose for the perfumery/cosmetic industry and the main extraction methods used. Three main products can be obtained directly from the
C. ladanifer plant: essential oil, using steam distillation or hydrodistillation, labdanum gum, employing hot alkaline extraction followed by acidulation, and cistus concrete, by extraction with apolar solvents, such as hexane and isopropanol. From these extracts, other products with interesting properties odoriferous and/or commercial can be further obtained, e.g., labdanum resinoid, labdanum oil, labdanum absolute from labdanum gum, and absolute from concrete. However, the portfolio of natural extracts obtained exclusively from
C. ladanifer can still be more diversified for use in many applications, such as fragrance and flavoring ingredients, among which are hydrolate, labdanum resinoid 50%/DPG (dipropylene glycol synthetic), hydrocarboresine, dynamone, cistus absolute SIS, cistus by-absolute, cistus by-colorless, cistus organic oil, cistus water concentrate, and others
[37].
Figure 2. Main conventional aromatic extracts obtained from C. ladanifer and respective extraction methods.
Table 1 shows the major constituents detected in
C. ladanifer essential oil from plants grown in different regions. The diversity observed can be due to several factors, such as climatic and soil variations, the stage of the vegetative cycle, seasonal factors, part of the plant analyzed, and the method used to obtain the essential oil, among others
[38].
Table 1. Chemical composition (relative % of the peak area) of
C. ladanifer essential oils from plants grown in different countries: center-interior of Portugal
[29], central Spain
[39], Corsica with plants of Spanish origin
[40], and eastern Morocco
[41].
a components identified using gas chromatography (GC) and/or gas chromatography/mass spectrometry (GC/MS); b components identified by C-NMR spectroscopy and GC.
2.2. Extractives
Both labdanum and essential oil are extractives obtained by selective extraction methods, but the extractives highlighted in this section correspond to a wider set of non-structural compounds that can be removed from the plant. Extractives comprise a wide variety of chemical compounds, generally of low molecular weight, that are not structural compounds of the plant cell walls
[42]. They can come from two general sources: (1) primary metabolites that include sugars, amino acids, simple fats, and various carboxylic acids, among others; and secondary metabolites, which are generally more complex compounds, such as starch, sitosterol, simple terpenoids, chlorophyll, phenylpropanoids, and phenolics; and (2) artefacts arising from later modifications of the metabolites or even from external sources, such as metabolites of microorganisms or lichens
[43].
The usual procedures for isolating extractives include traditional techniques (maceration, decoction, digestion, infusion, boiling under reflux, Soxhlet) and a wide range of modern techniques that have been introduced in recent decades (microwave-assisted extraction, ultrasound, fluid extraction supercritical, pressurized liquid extraction, enzyme-assisted extraction, among others)
[44].
Table 2 shows the major phytochemical categories of volatile and non-volatile compounds isolated from extracts solubilized from different parts of C. ladanifer that were identified using various chromatographic and spectroscopic methods.
Table 2. Major phytochemical constituents of C. ladanifer extracts obtained from various parts of the plant.
Extractives of
C. ladanifer are rich in terpenoids, such as monoterpenes, sesquiterpenes, labdane-type, diterpenes, and phenylpropanoids, including flavonoids, phenolics, and tannins, and carbonylic compounds
[52].
Phenolic compounds are considered one of the most important groups of plant secondary metabolites due to their large participation in the development of morphological, physiological, and reproductive processes
[53]. They comprise one or more aromatic rings with hydroxyl groups
[54] and may be grouped into several categories, such as phenolic acids and analogs, flavonoids, tannins, and stilbenes, among others.
Flavonoids and diterpenes (C20-carbon) are the main secondary metabolites and can contribute to a variety of functions, such as defense purposes, and are also precursors of hormones, such as tocopherols and gibberellins
[55]. Monoterpenes (C10-carbon) and sesquiterpenes (C15-carbon) are volatile compounds that contribute to plant odors and are repellants for herbivores, respectively
[52]. Flavonoids may vary according to the season since their synthesis is induced by climatic factors
[56]. Flavonoids such as apigenin, 4′-methyl-apigenin, 7-methyl-apigenin, 7,4′-dimethyl-apigenin, 3-methyl-kaempferol, 3,4-dimethyl-kaempferol, 3,7-dimethyl-kaempferol, and 3,7,49-trimethyl-kaempferol can be found in the leaf resin
[57]. Phenolic compounds such as ferulic acid,
p-hydroxybenzoic, vanillic,
p-coumaric, and caffeic acids, in association with terpenes (α and β-pinene), have been detected not only in aqueous extracts but also on soil samples occupied by
C. ladanifer [58]. Ellagitannins, in particular punicalagins derivatives, are the main compounds found in the aqueous extract of the aerial part of
C. ladanifer, with 0.24% of the extract for gallic acid and 3.50% for all ellagitannins. Tannin content has a significant proportion of 6.8% of the total dry matter of the extract
[59].
Diterpenes and flavonoid contents vary seasonally. The maximum concentration of diterpenes in the leaves occurs in winter and the minimum in spring–summer, but the maximum production of flavonoids is detected in summer and the minimum in winter. Low temperatures increase the amount of diterpenes, and higher temperatures increase the concentration of flavonoids
[60]. Flavonoids are systematically lower in plants grown in shaded areas than in those grown in open areas. These compounds protect plants from the harmful effects of UV radiation, and irradiation is the main inductor in the production of flavonoids. This induction may be synergistically increased by drought, where there is higher production of methylated flavonoids (kaempferols and 7-methylated apigenins), suggesting that the methylated form is part of the defense mechanism of the plant against hydric stress
[61]. Seasonal variations in the levels of phenolic compounds and condensed tannins have also been reported
[62]. Accumulation of phenolic substances in various tissues and their deposition in cortical cells defend the plants from predators and protect the internal tissues against UV-B radiation, respectively
[63].
Vitamins, reducing sugars, and polyunsaturated fatty acids (PUFA) were also detected in rockrose.
C. ladanifer leaves presented a very high level of ascorbic acid (647.6 µg/g dry weight of the plant) and of sugars such as fructose, glucose, sucrose, and raffinose, fructose being the most abundant (48.2 mg/g of the dry weight of the plant). Fatty acids such as eicosadienoic acid, arachidic acid, and linolenic acid were identified in cistus extracts
[64]. The highest concentration of PUFA occurs in winter and spring and that of branched-chain fatty acids (BCFA) in summer
[65].
3. Bioactivity
Various compounds from different phytochemical groups identified in rockrose are associated with different biological activities, namely antibacterial, antiviral, and antioxidant activities (
Table 3). The functional diversities presented by these compounds offer many opportunities for the development of new drugs and represent excellent sources for production of food additives, functional foods, nutritional and nutraceutical products for pharmaceutical industries, and natural food companies
[66].
Table 3. Biological activities of C. ladanifer extracts obtained with different solvents from different plant parts.
4. Novel and Potential Applications
Other potential applications have been suggested for C. ladanifer besides the traditional use of extracts in their already well established application in the perfumery industry as a fixative of perfumes. Some of the new directions regarding the valorization of rockrose include phytoremediation of soils contaminated by heavy metals, use of the plant for feed with nutritional benefits for animal health, and use as a lignocellulosic raw material for added value products, namely the production of bioethanol.
4.1. Phytoremediation
Phytoremediation is an emerging environmentally friendly and low-cost technology that uses plants and their associated microorganisms to remove pollutants from contaminated sites, especially heavy metals
[73].
C. ladanifer was reported to survive and grow in soils with high concentrations of toxic elements, such as Mn, Cu, Zn, Pb, and As
[74][75][76]. Observations in mining areas suggest selectivity in the absorption and translocation of metals. Therefore, the tolerant behavior of this species in soils with toxic elements can expand the possibilities of phytoremediation in environments with significant rates of contamination
[74].
One study on rockrose resistance to heavy metals was conducted in hydroponic experiments
[16]. Higher tolerance to metals, such as Cd, Co, Cr, Mn, and Ni, was observed in plant populations originating from ultramafic soils or soils developed on basic rock, while populations originating from acid rock soils exhibited higher tolerance to Cu and Zn. Different patterns were observed in the accumulation of metals in the plant: Cd, Co, and Mn accumulated in the aerial part, while Cu and Pb were not transported efficiently through the roots up to the shoots. In general,
C. ladanifer can accumulate heavy metals in the aerial parts without inhibiting plant growth
[16].
Thus, its potential for phytostabilization in mine soil can be considered suitable considering trace element concentrations in leaves and seeds and seed germination rates
[76]. Beyond immobilization of chemical elements, phytostabilization with autochthonous species also increases organic matter and water retention capacity, improving soil structure and reducing erosion
[77].
It is worth mentioning that hexane extracts from
C. ladanifer plants growing in mining areas did not exhibit potentially hazardous heavy metals, suggesting no human health risks
[32]. Therefore, the high resistance of
C. ladanifer to nutrient unbalanced soils, with potentially toxic elements and adverse climatic conditions, makes this species appropriate for phytoremediation and revegetation of contaminated soils
[17].
4.2. Animal Feed
The use of
C. ladanifer (soft stems and leaves or extracts) as a food supplement for animal nutrition and productivity increase has shown interesting results. The inclusion of leaves and soft stems of
C. ladanifer in diets of lambs supplemented with an oil blend (sunflower and linseed oils) increased intramuscular fatty acids content
[78]. The fatty acids provided by the diet with
C. ladanifer benefited the health and did not jeopardize animal performance.
Another approach using a
C. ladanifer diet, with or without oil supplementation, reduced lipid oxidation of lamb meat in pro-oxidant conditions and did not affect the meat’s sensory properties
[79].
Incorporation of ethanolic
C. ladanifer extracts in rabbit feed showed also to be possible since it did not affect productivity, although the consumption rate was higher due to excess fiber and low protein
[80]. Phenolic crude extracts from
C. ladanifer were also employed in the treatment of soybean meal to reduce rumen degradation, which may be advantageous to increase the flux of potential feed protein into the post-ruminal compartments
[81].
Several studies evaluated the effect of adding rockrose-condensed tannins in diets for lambs, showing that they can improve the digestive efficiency of soybean meal protein without compromising growth performance, blood metabolites, carcass characteristics, and meat quality, thus being able to reduce feed costs by reducing the content of protein used in diets
[82]. Inclusion of low levels of
C. ladanifer extracts of condensed tannins (1.25%) in the diet of lambs also showed favorable results in the pattern of ruminal biohydrogenation, while addition of 2.5% of condensed tannins negatively affected lamb growth, with no beneficial effect on the fatty acids composition of intramuscular and subcutaneous fat
[83]. An increase in the α-tocopherol content in the muscle, with a reduction in the lipid oxidation of the meat, has also been reported in studies with the incorporation of leaves and soft stems of
C. ladanifer in lamb diets
[84].
4.3. Added-Value Products from Lignocellulosic Material
Development of industrial activity, fluctuations in the fossil fuel markets, and the need to minimize global climate change impacts have led to increasing interest in the use of natural resources, in particular lignocellulosic residues and byproducts in the context of a transition to a circular bio-based economy. In this sense, the valorization of biomass within the biorefinery concept has been gaining increasing relevance since a biorefinery integrates biomass conversion processes to obtain energy, materials, and chemical products, namely of added value
[85]. These include biofuels, biochemical, and biobased compounds. Among these, organic acids, as well as bioactive compounds, are quite relevant. The phenolic compounds, both derived from extractives and from lignin, have strong potential for application in various industries, such as cosmetics, pharmaceuticals, and food, due to their potential functional activities.
Oligosaccharides, in particular xylooligosaccharides (XOS), are potential functional products that can be obtained from hydrolysis of hemicelluloses. These compounds have already been used as food ingredients (sweetener, weight control agents, humectants, etc.) and pharmacological supplements (prebiotic, anti-cariogenic, immunostimulant, antioxidant, antibiotic alternative, glycemic regulators, etc.), as well as in the cosmetic industry, animal and fish food, and agriculture
[86]. In a study involving the techno-economic and environmental assessment of lignocellulosic-based small-scale biorefineries, it was demonstrated that the market price for products, such as XOS (USD 4.05/kg), for example, can give rise to significant economic profits, taking into account that the associated production costs are rather competitive (USD 1.18/kg)
[87].
Rockrose lignocellulosic residues, from the production of essential oils and labdanum gum, are produced in significant amounts as these traditional products represent a small percentage of the total raw material. Thus, the products to be derived from the lignocellulosic fraction, even if they have a cheaper commercial cost, can be obtained in larger quantities. These residues are rich in polysaccharides and lignin, and, when they result from distilleries as extracted solid residues, they still contain an important fraction of extractives
[88][89][90]. According to the literature, the chemical composition of
C. ladanifer presents some variations depending on the plant parts and plant age. In ten years old whole plants, contents of polysaccharides, Klason lignin, and extractives of 41.5%, 15.6%, and 6.2%, respectively, were reported
[91]. For distillery residues, composed mainly of the aerial part of the plant, the polysaccharide values are around 29.2–33.3%, Klason lignin 17–19.2%, and extractives 39.2–43.7%
[88][90]. For extractive-free biomass, polysaccharide and lignin values increase to about 50% and 30%, respectively. Some abundant agro-industrial residues, such as olive pomace, sugar cane bagasse, or rice straw, have lower lignin contents than those found in extractive-free cistus biomass (16–26% vs. 29%) but higher polysaccharide contents (55–67% vs. 50%)
[92].
Recently, the researchers presented a set of works that suggest an integrated upgrading strategy for C. ladanifer distillery biomass residues obtained after essential oil steam distillation. The strategy started with the removal of extractives (40% of the dry biomass), producing an extract rich in phenolic compounds (mainly gallic acid and flavonoids) with antioxidant properties [89][90]. The remaining lignocellulosic material, containing mainly polysaccharides (51%) and lignin (33%), was subjected to selective fractionation processes for sequential recovery of hemicelluloses by an autohydrolysis process [89] and lignin by organosolv and alkaline processes [93], producing a solid enriched in cellulose that had increased enzymatic digestibility (approximately four times higher than the initial feedstock) [94].
Optimization of the autohydrolysis process enabled to obtain XOS with potential prebiotic activity, in a maximum concentration of 16 g/L, corresponding to a yield of 10.2 g/100 g extracted feedstock [89]. The alkaline and organosolv delignification processes affected the monomeric composition of the residual lignin, with a decrease in the S/G ratio (quantified by analytic pyrolysis, Py-GC/MS) and solubilization and recovery of several phenolic compounds with high added value, namely vanillic acid, p-coumaric acid, and epicatechin [93]. The alkaline treatments lead to higher delignification (87%) and subsequent higher cellulose enzymatic saccharification yields (79%) [93]. Glucan-rich solids and pentoses-rich hemicellulosic hydrolysates were used, separately or together, for the selective production of the D-lactic acid isomer (D-LA) by the recombinant strain E. coli JU15 in different fermentation modes: simultaneous saccharification and co-fermentation (SSCF), SHF, and SSF. In this study, high lactic acid yields, in the range of 92–99 g D-LA/100 g sugars were achieved [95].
5. Future Perspectives
The use of C. ladanifer, not only as a source of essential oils or labdanum gum but also as a feedstock to obtain other products, namely from its industrial residues, can be strategic in the expansion of essential oil distilleries and consequently in the development of rural areas where endogenous biomass potential is still poorly explored, promoting the bioeconomy and circular economy models.
The possible integration of processes and products for the valorization of C. ladanifer in a biorefinery framework is shown in Figure 3 with full resource use and exploitation to obtain added-value products or novel applications, conveying the biorefinery goals, i.e., integral and sustainable use of biomass for concomitant production of biofuels, energy, materials, and chemicals, preferably with added value [85].
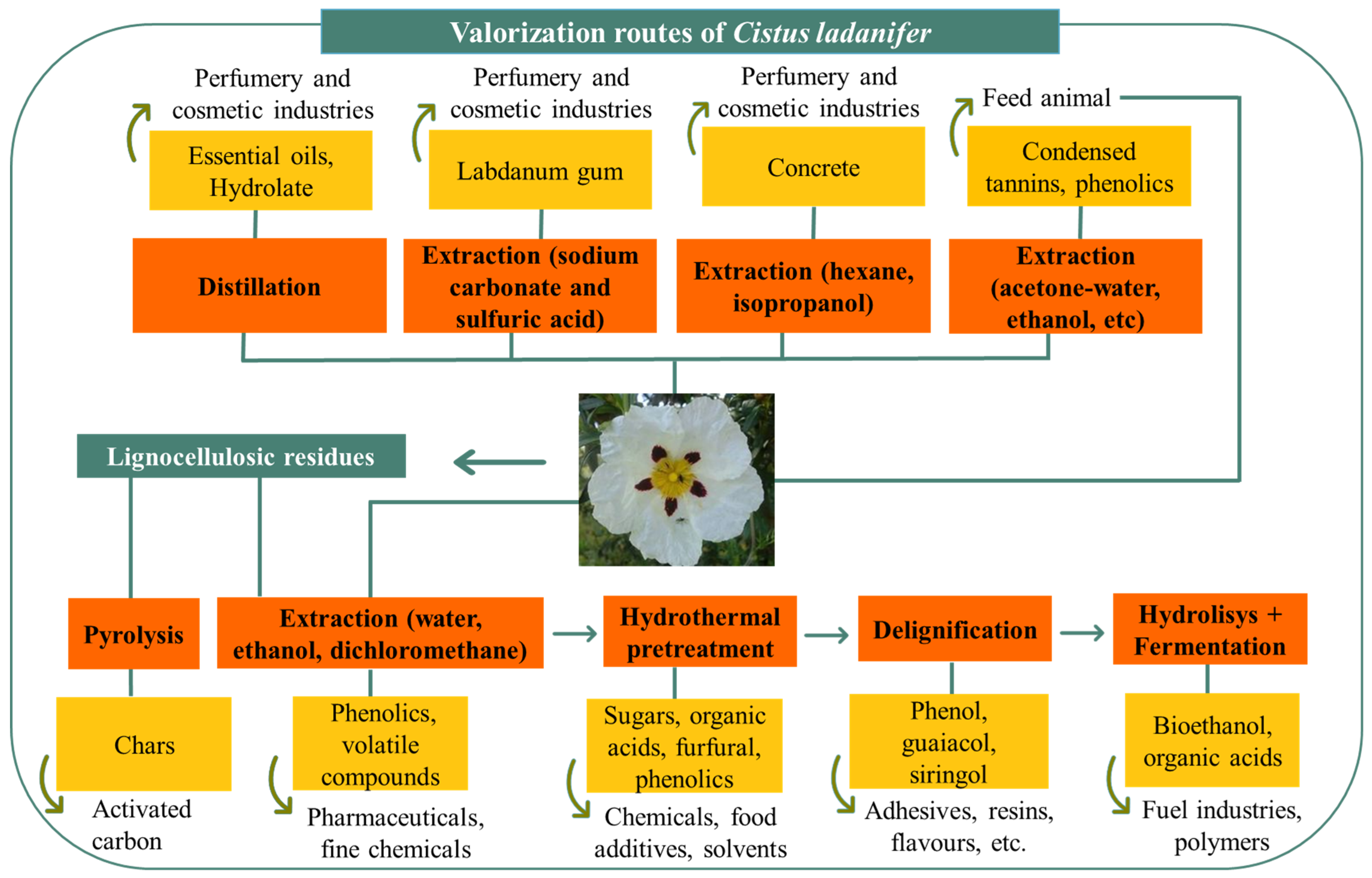
Figure 3. Potential path for use of Cistus ladanifer with an integrated valorization in a biorefinery framework for obtaining different added-value products.
Valorization of C. ladanifer may contribute to better use of an underexploited endogenous resource while promoting residue management, reducing pressure on the environment, and promoting sustainable development through the creation of new products and the generation of new jobs. Nevertheless, to shift biorefineries into industrial reality, the development of a distribution map highlighting local potential availability and aspects related to logistics of transport must be taken into consideration to build a complete sustainability analysis. Additionally, a comparative techno-economic analysis of the different processes, namely with the help of modeling tools, as well as a life cycle assessment in terms of environmental, social, and economic sustainability should also be carried out before selecting biomass commercial valorization pathways.