Xanthones are widely distributed in nature in higher plants, lichens, and fungi from terrestrial origins
[4][5][6][7][8][9]. In addition, the marine environment, the least explored area of this planet, has also proven to be an invaluable source
[10][11]. Many xanthones have been isolated from marine-derived fungi
[10][11][12][13][14][15][16][17][18][19][20][21][22][23][24][25][26][27][28][29][30][31][32][33][34][35][36][37][38][39][40], which can be found in marine sediments and associated with other marine organisms
[12][13][14][15][16][17][18][19][20][21][22][23][24][25][26][27][28][29][30][31][32][33][34][35][36][37][38][39][40]. Marine environments, such as temperature, salinity, and pressure, to which marine organisms are subject, sometimes force them to develop unique defenses against the conditions in which they live
[41][42]. These unique defenses can lead to the biosynthesis of new secondary metabolites, different from those synthesized by terrestrial sources
[41]. The chemically different/unique structures allow xanthones to have important biological activities, such as cytotoxic
[12][15][16][17][21][22][23][27][32][37][43], antibacterial
[12][16][17][18][19][20][22][26][29][30][35][36][39], and antifungal
[20][22][33][40] activities, giving them great potential as natural products with medicinal value
[1].
Therefore, the chemical uniqueness of marine-derived xanthones and the significant bioactivities support the idea that the marine environment could be a valuable source of new hits, leads, and drugs
[44]. However, access to some marine natural products may be difficult, and in some cases, isolation may yield small amounts of compounds
[45]. Different techniques have been applied to increase the availability of the new natural compounds, which can be produced by bacteria or yeasts, or through a chemical approach, such as laboratory synthesis
[45]. Synthetic routes can help to overcome supply problems, but it is also very important since it allows the preparation of structures with different substitution patterns relative to those provided by nature, which rise to the opportunity to generate new bioactive agents
[46][47][48]. Bioinspiration is very useful in medicinal chemistry as it allows the selection of molecular structures to be used as scaffolds and the strategy to follow for molecular transformations
[2].
2. New Marine Xanthones Isolated since 2010
Under the period covered by this research, several studies were carried out to identify biologically active marine compounds, of which the xanthones stand out. Around 100 xanthones were isolated, from which 51 are considered new natural compounds.
Nowadays, the isolation of xanthone derivatives from marine sources usually involves fermentation to increase the compound’s final amount. Fermentation involves putting the fungi in a culture broth, which comprises all the nutrients, such as glucose, iron phosphate, calcium carbonate, malt extract, and controlled pH
[21][26], necessary for its growth. The duration of fermentation can be variable but usually involve several days, at least 14 days and the highest 45 days
[14][17]. Then, the cultured broth is filtered and extracted, most often, with EtOAc. This extract is subjected to a vacuum liquid chromatography over a silica gel column
[15][16][21][23], flash chromatography
[19] or, most commonly, to normal column chromatography (CC)
[14][17][18][20][22], originating different fractions, which can be subjected to analysis by HPLC or TLC, obtaining sub-fractions, or be directly purified to obtain pure compounds. Most of the xanthones herein referred to were obtained after semi-preparative HPLC purifications of these sub-fractions. Naturally, the eluents and gradients used differ depending on the extracts/fractions being analyzed. The most common eluents used are organic solvents mixtures, such as
n-hexane/CH
2Cl
2/MeOH
[16],
n-hexane/EtOAc
[15], CH
2Cl
2/MeOH
[15][18][20][21][23], gradient of petroleum ether/EtOAc
[14][17][18], but mixtures of H
2O/MeOH can also be used successfully
[19].
The new xanthone derivatives isolated include simple ones (
Figure 2), mainly isolated from marine-derived fungi found in marine sediments
[15][18][20][31][32][33][34][35][36][46][47][48][49]. These xanthones are usually highly substituted, with different substituent patterns being the substituents found to be essential for four types; hydroxy, methoxy, methyl, and methoxycarbonyl groups. It seems that the methoxy group is not common in bioactive marine xanthones
[50], but yicathins B (
3) and C (
4) (
Figure 2), which were recently prepared by total synthesis
[51], present such a group at C-8. Other examples include xanthones bearing a 6-methoxy group (
2) and (
6) and 7-methoxy group (
13) and (
15) (
Figure 2). On the other hand, marine xanthones (
14) and (
16) present two methoxy groups, respectively, at C-3 and C-7 and at C-2 and C-7 (
Figure 2).
Figure 2. Marine xanthones with simple structures isolated for the first time from 2010 to 2021.
The more complex xanthones isolated during the covered period are depicted in
Figure 3 and present the xanthone skeleton fused with other rings. The most interesting examples include (2
S,3a
S,12c
S)-8-hydroxy-2,6-dimethoxy-1,2,3a,12c-tetrahydro-7
H-furo [3’,2’:4,5] furo [2,3-
c] xanthen-7-one (
18), isolated from the marine fungus
Aspergillus versicolor and named oxisterigmatocystin D due to its similarity with the known oxisterigmatocystin C
[37]. Although the similarities with oxisterigmatocystin C and other isomers were previously isolated, the researchers established the stereogenic centers’ configurations and demonstrated that oxisterigmatocystin D is a new natural compound
[37]. Compound (
19), named monacyclione G, is another example isolated from
Streptomyces sp. HDN15129
[21]. Its stereochemistry was confirmed by the NMR data mainly through the correlations found in HMBC
[21] and was established as depicted in
Figure 3.
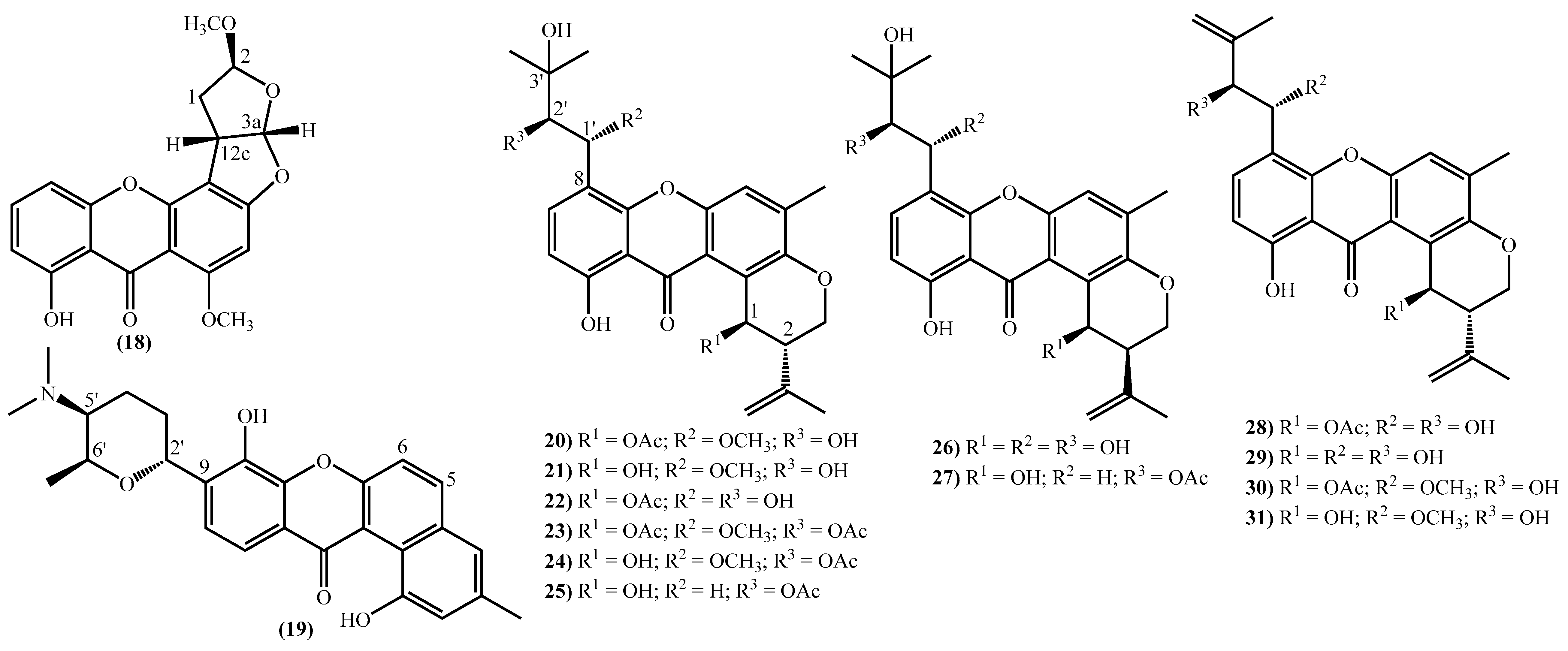
Figure 3. Marine xanthones with more complex structures isolated for the first time from 2010 to 2021.
The most prevalent nucleus in these new marine xanthones is the 11-hydroxy-5-methyl-2-(prop-1-en-2-yl)-2,3-dihydropyrano [3,2-
a]xanthen-12(1
H)-one, found in fourteen of the twenty-one new derivatives (
Figure 3). Twelve marine xanthones with this core were isolated from the fungus
Aspergillus sp. ZA-01, respectively, aspergixanthones A-K (
20–
24 and
26–
31) and the 15-acetyltajixanthone hydrate (
25) (
Figure 3)
[14][17]. Varioxiranols F (
37) and G (
38) (
Figure 3) were isolated from the
Emericella variecolor, a fungus associated with a
Cinachyrella sp. Sponge
[28].
The aspergixanthones (20–24, 26 and 27) and the 15-acetyltajixanthone hydrate (25) have a 3-hydroxy-3-methylbutyl moiety at C-8, whereas the remaining aspergixanthones (28–31) have at the same position a 3-methylbut-3-en-1-yl substituent (Figure 3), in both cases, hydroxy, methoxy or acetyloxy groups at C-1′ and C-2′ are also present (Figure 3).
The last examples are xanthone derivatives bearing a 1,7,8,10-tetrahydroxy-11-methoxy-5,6-dihydro-9
H-naphtho [2,1-
c]xanthen-9-one (
32,
33) and a 1,9,14-trihydroxy-5,6-dihydro-8
H-naphtho [2,1-
b]xanthen-8-one nucleus (
34–
36) (
Figure 3). Buanmycin (
34) was extracted from a marine
Streptomyces cyaneus [52], and the remaining examples, citreamicin θ A (
32), citreamicin θ B (
33), citreaglycon A (
36), and dehydrocitreaglycon A (
35), were isolated from
Streptomyces caelestis [19].
In the last years, complex tetrahydroxanthone derivatives were also isolated (
Figure 4)
[23][26][27][40]. Compounds (
39–
44), named versixanthones A-F, were isolated from the fungus
Aspergillus versicolor HDN1009
[27]. Compounds (
45,
46) were isolated from the fungus
Engyodontium album strain LF069
[26] and the
Tritirachium sp. SpB081112MEf2 a marine sponge fungus
[40]. Finally, compounds (
47–
51) were also isolated from the fungus
Aspergillus versicolor HDN1009
[23].
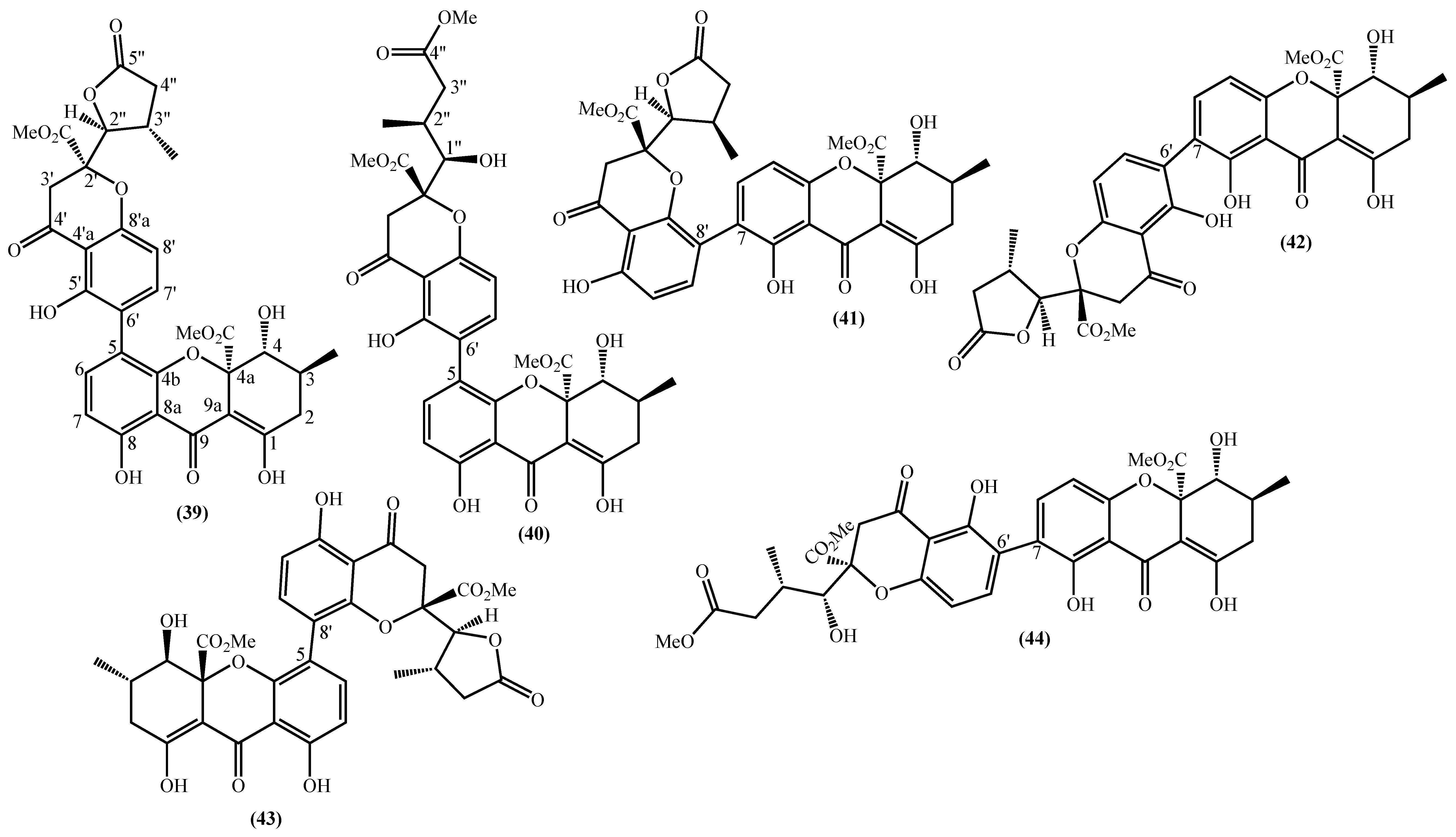
Figure 4. Marine tetrahydroxanthones isolated for the first time from 2010 to 2021.
Versixanthones A–F have a methyl (3
R,4
S,4a
S)-1,4,8-trihydroxy-3-methyl-9-oxo-2,3,4,9-tetrahydro-4a
H-xanthene-4a-carboxylate moiety linked to a methyl (2′
R,2″
S,3″
S)-5-hydroxy-2-(3-methyl-5-oxotetrahydrofuran-2-yl)-4-chromanone-2-carboxylate (
39,
41–
43) and methyl (2′
R,1″
R,2″
S)-5-hydroxy-2-(1-hydroxy-4-methoxy-2-methyl-4-oxobutyl)-4-chromanone-2-carboxylate (
40,
44). The main differences are the linkage between the two moieties since the researchers establish the stereocenter configurations as equal in all isomers (
Figure 4)
[27].
Compounds (
45,
46) represent two diastereomers fully characterized by Wu et al. in 2016
[26], although the isomers JBIR-99 (
45a) and JBIR-97/98 (
46a) were previously isolated by Ueda et al. in 2010
[40]. The absolute configuration of these isomers and the engyodontochone A (
46b) and B (
45b) was established using NMR experiments and is depicted in
Figure 4 [26].
The final examples are the dimeric tetrahydroxanthones (
47–
51) isolated from the fungus
Aspergillus versicolor HDN1009 and named versixanthones G-K (
Figure 4)
[23]. The structure depicted in
Figure 4, including the absolute configuration, was established using NMR experiments.