The gut microbiota has been designated as a hidden metabolic ‘organ’ because of its enormous impact on host metabolism, physiology, nutrition, and immune function. The connection between the intestinal microbiota and their respective host animals is dynamic and, in general, mutually beneficial. This complicated interaction is seen as a determinant of health and disease; thus, intestinal dysbiosis is linked with several metabolic diseases. Therefore, tractable strategies targeting the regulation of intestinal microbiota can control several diseases that are closely related to inflammatory and metabolic disorders. As a result, animal health and performance are improved. One of these strategies is related to dietary supplementation with prebiotics, probiotics, and phytogenic substances. These supplements exert their effects indirectly through manipulation of gut microbiota quality and improvement in intestinal epithelial barrier.
1. Introduction
The permeability of the intestinal tract controls the uptake of nutrients and the transport of unwanted extracellular substances such as bacteria and xenobiotics, in addition to the non-digested substances. Therefore, gut health plays an essential role in the pathogenesis of various intestinal disorders. The permeability of the intestine is controlled by gut microbiota, digestive secretions, physical barriers (mucin, intestinal epithelial cells lining and tight junctions), and chemicals such as cytokines
[1].
Under normal conditions, the symbiotic relationship between the gut microbiota and the host crucially determines intestinal health. However, a disturbance in the gut microbiota can lead to an imbalanced host–microbe relationship, which is called “dysbiosis”
[2]. Several factors, such as antinutritional factors in feed, heavy metals, toxic substances, bacterial toxins, herbicides, and antibiotics, can disrupt the gut microbiota. These impacts can lead to localized inflammation, extensive infection, or even intoxication
[3][4][5], Additionally, the intestinal epithelium forms tight connections, acting as a biological barrier that controls the paracellular transit of different materials across the intestinal epithelium, including ions, solutes, and water. It also functions as a barrier of extracellular bacteria, antigens, and xenobiotics.
The impaired intestinal barrier function, commonly known as “leaky gut”, is a condition in which the small intestine lining becomes damaged, leading to infiltration of luminal contents such as bacteria and their associated components including toxins to pass between epithelial cells. These conditions subsequently lead to cell damage and/or inflammation of the intestine, characterized by increased levels of bacteria-derived endotoxins in blood. This inflammatory process consumes significant amounts of nutrients, and, subsequently, has negative effects on metabolic responses, in particular on immunometabolic and endocrine responses. As a result, animal performances are severely reduced
[6].
2. Intestinal Microbiota in Poultry
Microorganisms that live in animals’ gastrointestinal tracts (GITs) are a prime example of beneficial bacteria
[7]. Indeed, the GIT is the home of a diverse and plentiful microbial community providing essential functions to their host animals. Although the intestine is exposed to microflora components from birth or hatching, little is known about their impact on healthy development and function. Microorganisms are more densely populated in the GIT than in any other organ
[7]. Animals have evolved the ability to host complex and dynamic consortia of microbes over their life cycle during millions of years of evolution
[8].
Colonization of avian guts could already start during embryogenesis
[9] and progresses to the formation of a complex and dynamic microbial society
[10]. Based on principles established during animal history, extensive and combinatorial microbial–microbial and host–microbial interactions are likely to govern the microbiota assembly
[11]. Comparing germ-free rodents that were raised without exposure to microorganisms to those that built up a microbiota since birth, or those that were colonized with microbiota components during or after postnatal development, a variety of host functions influenced by indigenous microbial communities were identified
[12].
3. Intestinal Barrier and Tight Junctions
Enterocytes are the cornerstone of the intestinal mucosal monolayer that protects the host from the external environment. A scheme of the intestinal epithelial barrier and some interactions with intestinal microbiota is shown in
Figure 1. Enterocytes are connected by the so-called tight junctions (TJs), which constitute a continuous belt of intimate contacts formed during the assembly process of integral transmembranes (occludin, claudins, junctional adhesion molecules (JAMs), and tricellulin) and peripheral membranes (zonula occludens-1 (ZO-1), ZO-2, and ZO-3). The TJ proteins are located between adjacent enterocytes, sealing the paracellular space and regulating the permeability of the intestinal barrier. Therefore, these proteins prevent the transit of microorganisms, toxins and other antigens from the intestinal lumen to the systemic circulation
[13][14]. The formation and function of tight junctions are controlled by intracellular signal transduction pathways: (i) protein kinase C (PKC), A (PKA), and G (PKG) signaling, (ii) phosphatase-Rho, myosin light chain (MLC) kinase (MLCK), MAPK signaling, and (iii) the PI3K/Akt pathway
[15][16].
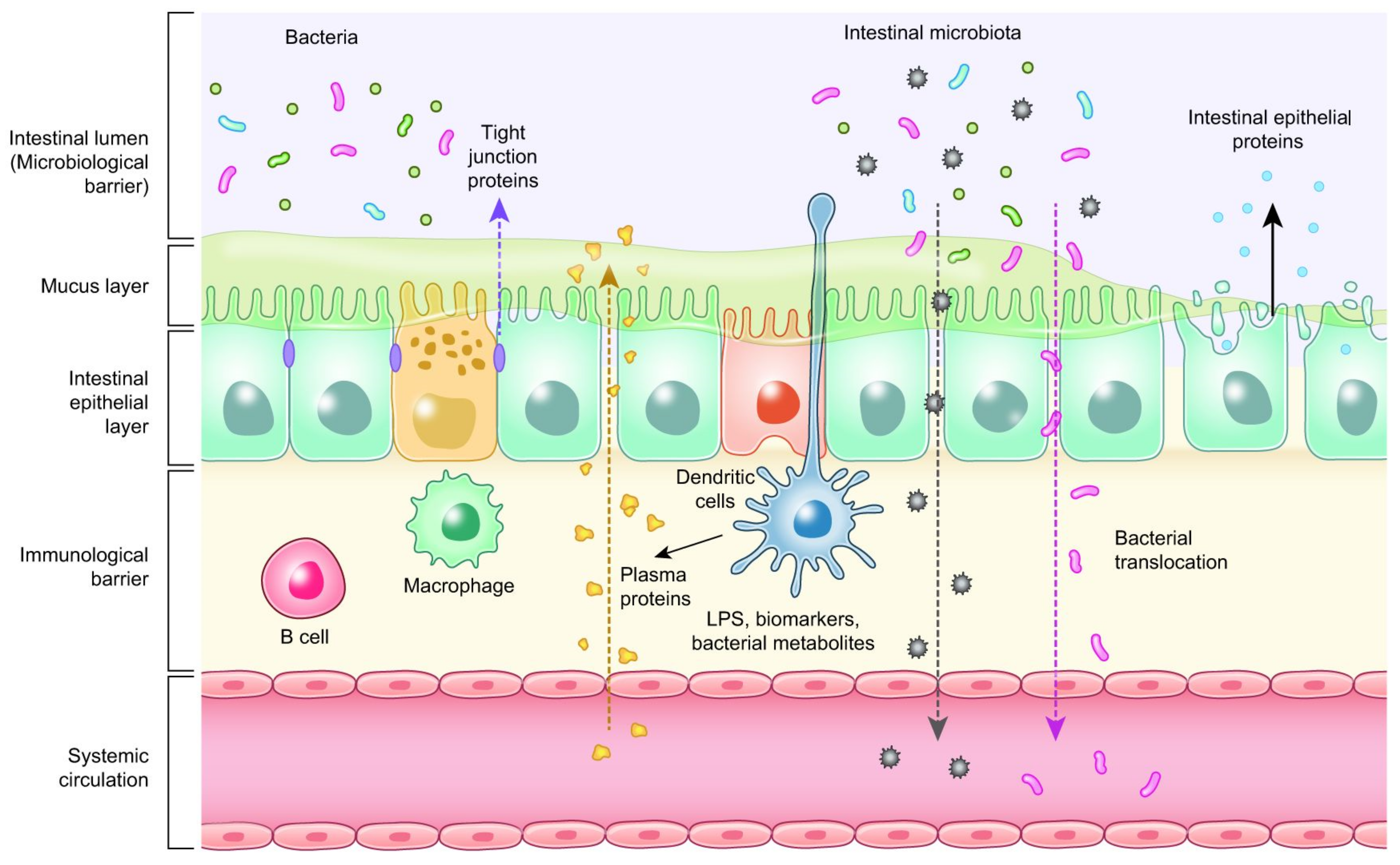
Figure 1. Intestinal epithelial barrier and intestinal microbiota interaction.
4. Biomarkers Related to Intestinal Health of Animals
The interactions between the epithelial barrier function, intestinal inflammation, and the microbial environment influence gut health
[17][18]. Therefore, the discovery of reliable, widespread biomarkers to measure intestinal inflammation and barrier function is an important ongoing area of research. A summary of some of the known biomarkers related to intestinal health is presented in
Table 1. To study intestinal health, it is also important to develop inflammatory gut models with different challenge conditions (anti-nutritional factors, pathogens, toxins, and environmental triggers)
[19][20]. Inflammation can also be associated with oxidative stress and changes in the expression of genes related to oxidative stress, indicating that oxidative stress may have a critical role in the physiological intestinal function
[21]. One quantitative technique that is used to evaluate the integrity of tight junction proteins in epithelial cell monolayers is the measurement of transepithelial electrical resistance (TEER)
[22]. Mitochondrial respiration is required to maintain TEER, implying that oxidation plays a critical role in Caco-2 cell tight junction stability
[23].
Table 1. Potential biomarkers to evaluate intestinal health.
Measurement/Function |
Biomarker Type |
Antioxidant activity |
Superoxide dismutase (SOD), Thiobarbituric acid reactive substances (TBARS), Total antioxidant capacity |
Gene expression of host protein biomarkers and tight junction |
Fatty acid binding protein (FABP), Fibronectin, Occludin, Zonula occludens, Claudins |
Immune activity |
Acute phase proteins, Calprotectin, Lipocalin, Immunoglobulins (IgA), Interferon gamma (INF-γ) |
Intestinal permeability |
Fluorescein isothiocyanate dextran (FITC-d), Trans epithelial electrical resistance (TEER), Bacterial translocation |
Enterocyte function |
Extracellular signal-regulated kinase (ERK), Citrulline |