Any compound or mixture of compounds, which interferes with any aspect of the endocrine system is classified as an endocrine-disrupting chemical (EDC). There is increasing evidence that male infertility is associated with the widespread exposure to EDCs, and in particular to obesogens. These compounds interfere with hormones involved in the regulation of metabolism and are associated with weight gain, being also able to change the functioning of the male reproductive axis and, consequently, the testicular physiology and metabolism that are pivotal for spermatogenesis. The disruption of these tightly regulated metabolic pathways leads to adverse reproductive outcomes. The permanent exposure to obesogens has raised serious health concerns. Evidence suggests that obesogens are one of the leading causes of the marked decline of male fertility and key players in shaping the future health outcomes not only for those who are directly exposed but also for upcoming generations. In addition to the changes that lead to inefficient functioning of the male gametes, obesogens induce alterations that are “imprinted” on the genes of the male gametes, establishing a link between generations and contributing to the transmission of defects.
1. Introduction
Today, people are exposed to a cocktail of man-made chemicals, some of which have the potential to disrupt the hormonal system. Any compound or mixture of compounds, which interferes with any aspect of the endocrine system is classified by the Endocrine Society as an endocrine-disrupting chemical (EDC)
[1]. These chemicals are ubiqutious in consumer products, being widely detected in the indoor environment (e.g., homes, workplaces, schools, gyms, transportation) and in diet samples. Generally, their occurrence in the indoor environment is a consequence of their widespread use in construction materials and consumer products, including, for example, house furnishings, electronic devices, wallpaper, textiles, kitchen utensils, cleaning products, and cosmetics, amongst many others. Indoor activities such as the use of biocides, deodorisers, the burning of incense and candles, smoking and cooking are also responsible for the release of EDCs into the indoor environment. Furthermore, all materials carried from outdoors on our shoes or clothes also increase our exposure. EDCs are also present in food items due to food production (e.g., pesticides), processing (antimicrobials, preservatives) and storage (plasticizers), which makes diet a significant route of EDCs. The group of compounds classified as EDCs is extremely heterogeneous and includes industrially produced chemicals, such as polychlorinated biphenyls (PCBs), bisphenol A (BPA), phthalates, pesticides, fungicides, and pharmaceutical drugs
[1]. Likewise, natural chemicals found in human and animal diets (e.g., phytoestrogens, such as genistein and daidzein, or even mycotoxins) can also act as EDCs
[1]. Furthermore, several of these compounds are lipophilic which make them particularly relevant from a physiologic point of view. EDCs act by different mechanisms and elicit several responses. One recent concern about the adverse effects of EDCs is the ability that some of them have to induce weight gain
[2]. Today these chemicals are classified as obesogens, a term coined by Blumberg and Grun in 2006
[3]. Obesogens are a limited group of EDCs capable of inducing adipogenesis and altering the mechanisms that regulate appetite, satiety and energy metabolism. Though the relationship between lifestyle habits of modern societies and the obesity rates has been established, obesogens have been considered major contributors to the obesity epidemic
[4][5][6]. The mechanisms by which obesogens exert their effects are much broader than was initially postulated, see reviews
[7][8][9][10][11]. In Europe, exposures to EDCs have contributed substantially to obesity
[4][12][13][14][15] and albeit obesogens are only part of the problem, it is necessary to become aware of the risks of permanent exposure to these chemicals.
In parallel to the adverse effects of obesogens on whole body metabolism, another issue of concern is male reproductive health problems resulting from exposure to EDCs, whether they are obesogenic or not. In the last decades, male fertility has decreased significantly. Today, 15% of the population is infertile, and one in seven couples is infertile at reproductive age
[16]. About 50% of the cases are due to the male factor, and in many of them, it occurs due to idiopathic infertility
[16]. The decline of male fertility has also been concurrent with the overall increase in exposure to EDCs, leading to these contaminants being singled out as an important cause of male infertility
[17].
The male reproductive function is highly susceptible to the effects of EDCs
[17][18], and most of these chemicals are lipophilic, mimicking naturally occurring hormones. Male reproduction is highly dependent on endocrine regulation, especially the reproductive events, such as steroidogenesis and spermatogenesis that are dependent on Leydig (LCs) and Sertoli cells (SCs), respectively
[19]. The majority of EDCs interfere with the receptors of endogenous hormones, impairing genome and non-genomic responses
[20], which is extremely relevant since most of the effects are exerted through disturbance of estrogen-, anti-estrogen, androgen- and anti-androgen-mediated processes
[21][22]. However, some EDCs can modify hormone bioavailability by interfering with its secretion and transport or by disrupting the enzymatic pathways involved in hormone synthesis and metabolism
[23][24]. It is essential to understand how EDCs, especially obesogens, induce male infertility namely in the deregulation of the mechanisms that govern the function of the reproductive axis, sperm formation, and its functioning (
Figure 1).
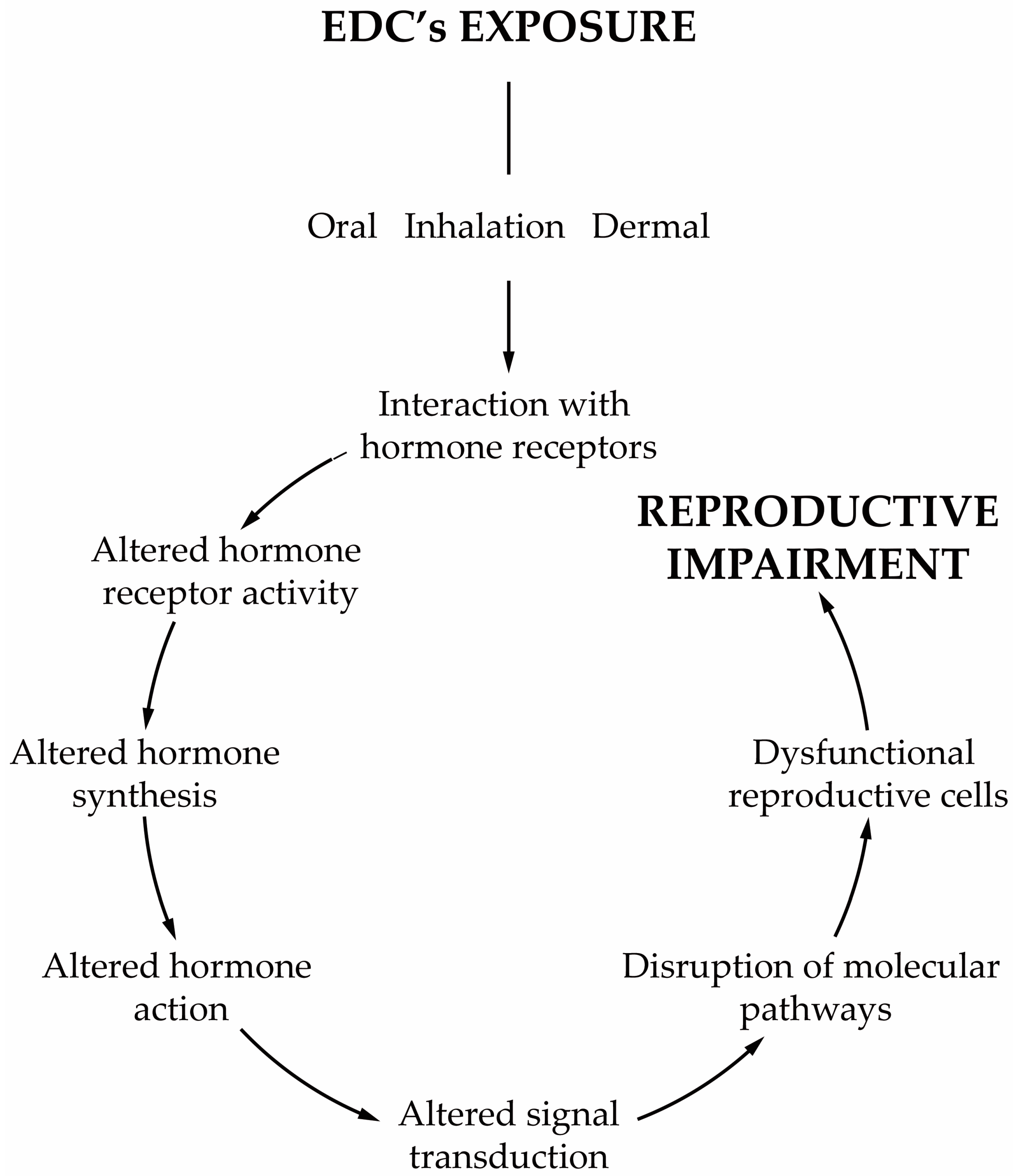
Figure 1. Schematic representation of the most important characteristics of obesogens’ effects.
2. Obesogens as a Threat to Male Fertility
The obesogen hypothesis proposes that exposure to obesogens affects the biochemical pathways that control the whole metabolic homeostasis. Obesogens are present everywhere, and one of the first studies demonstrating that obesogens could affect the reproductive capacity through “modulation” of the endocrine system dates from the late 1960s
[25]. Since then, several reports evidenced that the primary concern regarding obesogens is based on their capacity to induce endocrine disruption
[1]. Taking into account that today the time period in which men are exposed to obesogens is large, this issue deserves special attention from all professionals of the reproductive area to understand how these toxics impact male fertility. Compelling evidences have linked the decline of male reproductive health with permanent exposure to obesogens in both developed and developing countries
[26][27]. Obesogens alter the male reproductive function primarily by exerting adverse effects on the central nervous system. Below, a brief description of the effects of obesogens in the neuroendocrine control of male fertility, namely in the hypothalamus, is provided. Male reproductive organs may be a free spot where obesogens can be stored, albeit this is a subject of conjecture
[28]. It is known that obesogens effectively cross the biological barriers
[29], but the extent to which environmental chemicals pass the blood–testis barrier (BTB) remains mostly unknown.
3. Reproductive Axis at the Interface with Environmental Obesogens
The central neuroendocrine system controls all processes in the body, reproduction included. All molecular events are initiated at the base of the brain, where the hypothalamus is located, which serves as the primary interface between the central nervous system and the testicles, in this case. The hypothalamic neural cells synthesize and release the gonadotropin-releasing hormone (GnRH) into the capillary portal system that vascularizes the anterior pituitary. The gonadotrophs in the pituitary response to this stimulus is the release of the corresponding hormones, luteinizing hormone (LH) and follicle-stimulating hormone (FSH) into the bloodstream. FSH and LH act as a functional link between the brain and testes by working on testicular cells to regulate the spermatogenic event
[30]. LH binds to membrane receptors of LCs and stimulates T synthesis, influencing the development of peritubular cells, SCs and, consequently, germ cells
[31]. LCs irreversibly convert T into 17β-estradiol (E
2) by aromatase P450
[32]. On the other hand, FSH binds to membrane receptors on SCs, stimulating the production of E
2, activin and inhibin B. E
2 inhibits T production by LCs, while activin and inhibin B produce a positive/negative feedback on the pituitary, respectively. There is evidence that endocrine disruptors affect the neuroendocrine systems, and most of the studies have focused on the hypothalamus–pituitary–testicular (HPT) axis, termed the reproductive axis. Endocrine disruptors can exert diverse actions over the target cells according to their chemical structure and activities. In vitro evidences demonstrate that GnRH GT1–7 cell lines exposed to low doses of PCB mixtures, Aroclor 1221 or Aroclor 1254, increased the expression of the GnRH gene
[33]. In this case, the endocrine disruption occurs at the level of the hypothalamus
[33]. Similar studies using GT1–7 cell lines exposed to small doses of organochlorine pesticides demonstrated an increased expression of GnRH, whereas when exposed to high doses, the opposite effect was observed
[34]. Although these works showed a positive response of GnRH cell lines to environmental obesogens, other studies demonstrated different outcomes. In fact, explanted hypothalamus from male rats exposed in utero to dioxins displayed an increased content of GnRH peptide, which was, however, accompanied by an impaired release
[35]. Obesogens can favor or reduce the release of neuroendocrine hormones, but this will depend on the type of obesogens, the time of exposure and perhaps the levels to which disruption in the reproductive axis occurs. Different environmental compounds may target the HPT axis at various sites with different intensities, disrupting its regulation.
4. Leydig Cells Are a Sensitive Target of Obesogens
The synthesis of sex steroid hormones occurs in the Leydig cells (LCs) which are extremely sensitive to the toxic effects of environmental contaminants. The deleterious effects of EDCs often conduct the impairment of hormone synthesis since they are associated with the inhibition of the activities of enzymes involved in steroidogenesis. Lipids are essential for this process and cholesterol is one of the primary precursors, so it would be expected that any dysregulation in the homeostasis of these components compromises the synthesis of steroid hormones. Indeed, the pesticide 2,4-dichlorophenoxyacetic acid (2,4-D) decreases the expression of 3-hydroxy-3-methylglutaryl coenzyme A synthase (HMG-CoA synthase) and reductase (HMG-CoA reductase) in LCs of mice
[36]. These enzymes are pivotal for cholesterol synthesis, where HMG-CoA synthase is responsible for the condensation of acetyl-CoA with acetoacetyl-CoA to form HMG-CoA, which is reduced to mevalonate for the synthesis of cholesterol. This is one of the most important steps of the cholesterol biosynthesis, where the enzyme responsible for this committing conversion is HMG-CoA reductase. The impairment of cholesterol synthesis after exposure to 2,4-D disturbs testosterone synthesis. In the steroidogenesis, cholesterol is then carried from the outer mitochondrial membrane by steroid acute regulatory protein (StAR), where it is converted to pregnenolone via P450 side chain cleavage enzyme (P450scc). Then, this intermediate proceeds to the smooth endoplasmic reticulum where 3β-hydroxysteroid dehydrogenase (3β-HSD) turns it into progesterone. Progesterone is converted by P450c17 into 17-hydroxyprogesterone and into androstenedione, where the enzyme 17β-hydroxysteroid dehydrogenase converts it into T. It has been demonstrated that exposure to plasticizers such as di(n-butyl)phthalate decreased the expression of cholesterol transport genes such as StAR, the high-density lipoprotein receptor, also known as SRB1
[37]. Furthermore, the expression of genes involved in T biosynthesis, namely P450scc, 3β-HSD, and P450c17, was downregulated, which probably contributes to T deficiency
[38]. It seems that the plasticizer DEHP exerts anti-androgenic effects directly onto LCs, inhibiting T synthesis probably through dysfunction of CYP17
[39]. However, the impact of its metabolite, MEHP, is much more pronounced since it inhibited the expression of all steroidogenic enzymes, as well as all T precursors, in both the Δ4 and Δ5 steroidogenic pathways
[39]. The effects of both phthalates appeared to be specific for steroidogenesis since they did not alter the expression of the insulin-like 3 gene, a specific marker of LCs. In vivo models have also provided evidence of the cytotoxic effects of similar obesogens in testicular function as observed after daily exposure to 200 mg/kg/day of BPA for six weeks. Such exposure not only revealed the same results, but also disclosed a reduced number of LCs
[40].
5. Mechanisms Mediating Obesogen-Related Sertoli Cell Dysfunction: A Metabolic Standpoint
Typically known as “nurse cells”, Sertoli cells (SCs) are extremely important in male fertility, ensuring all physical and nutritional support of the germ cell line. Spermatogenesis is under the strict control of hormonal and endogenous factors
[41][42], so SCs become an easy target of obesogenic EDCs mimicking endogenous hormones
[43]. EDCs (obesogens included), not only compromise all structure of SCs, but also induce various cellular and molecular insults
[44][45]. Additionally, the interactions between adjacent SCs are disrupted, provoking the premature exfoliation of germ cells
[46]. The impact of EDCs on the integrity of cytoskeleton disruption of SCs and BTB has been extensively explored and well documented by Cheng and his collaborators
[18][29][45][47][48][49][50][51][52].
6. How Can Germ Cells Be Affected by Obesogens?
Compelling evidence shows that environmental contaminants induce several dysfunctions associated with the testicular dysgenesis syndrome, including seminiferous tubules atrophy and germ cell degeneration
[53]. Furthermore, lipids are essential components of the membranes of germ cells. Deficient lipid incorporation into these cells leads to a defective germ cell structure and contributes to the impairment of sperm parameters, and consequently to alterations of sperm functionality. The oral administration of TBT has also been associated with induced apoptosis in testicular germ cells in prepubertal mice
[28]. Those authors did not describe the molecular mechanisms by which germ cell apoptosis was induced, but according to recent evidence, it seems that environmental contaminants activate both intrinsic and extrinsic pathways of apoptosis. Mitra and collaborators
[54] observed a caspase-3 activation and increased levels of pathways of phosphorylated c-Jun N-terminal kinase (JNK) and mitogen-activated protein kinase p-38 (p38-MAPK) in SC–germ cell co-cultures after being exposed to 600 nM of TBT. JNK activation serves as a pro-apoptotic signal and regulates the mitochondrial apoptotic pathway through a balancing act between the activation of pro-apoptotic members and inhibition of anti-apoptotic members of the Bcl2-related protein family
[55]. On the other hand, the p-38 caspase-independent pathway seems to be also activated by TBT and thus plays a role in the germ cells’ death
[56].