1. Introduction
Dendrimers are hyperbranched macromolecules composed of monomers radially emanating from a central core
[1] (
Figure 1). Due to their repetitive structure, dendrimers can be considered polymers, but in contrast to polymers, they are never synthesized by polymerization reactions, but step-by-step in an iterative fashion, creating layers. Each layer is called “generation”
[2][3]. Higher-generation dendrimers (G > 3) have a near-globular shape
[4].
Figure 1. Schematic drawings of a dendrimer and dendron.
Owing to their peculiar synthesis, the structure of dendrimers is perfectly defined and highly reproducible. An isolated branch of a dendrimer is called a “dendron”. By choosing structural elements of dendrimers, one can opt for their physicochemical properties, adjust the molecular weight, functional group density, as well as modify dendrimers with different functionalities. The synthetic flexibility allows one to optimize their structure according to a specific task.
Dendrimers are a promising platform for designing functional species owing to their multivalency, which is reminiscent of the multivalent interactions widely found in nature. Multivalent interactions can be collectively much stronger than the corresponding monovalent interactions, in particular through multiple ligand–receptor interactions. Dendrimers, which are inherently multivalent species, are widely used in different fields of science and technology, such as catalysis
[5], biomaterials
[6][7], regenerative and cell biology
[8][9][10][11], and nanomedicine
[12][13][14][15].
One of the most intriguing properties of dendrimers and dendrons is the so-called “dendritic effect”
[16], i.e., a dramatic increase in efficiency when using a dendrimer compared to monomer. The dendritic effect is influenced by the nature and size of the core as well as by the cooperativity of functional groups at the periphery. The dendritic effect-enhanced multivalency and structure uniformity are the major differences between dendrimers and hyperbranched polymers. They frequently define the dendrimer properties and behavior in different processes at the nanoscale level.
Apart from dendrimers, the use of dendrons is considered highly promising. Unlike dendrimers, dendrons have two topologically and chemically different sites: the focal point and the periphery, which enables their selective functionalization with various moieties, depending on the desired application. Such a feature allows dendrons to exhibit enhanced functionality compared to that of dendrimers. Today, dendrons have been applied as agents for imaging, catalysis
[17][18][19], healthcare
[20][21][22][23][24], nanoparticle stabilization and functionalization
[25][26][27][28][29], surface modification
[30][31], etc.
Amphiphilic dendritic species are an emerging class of dendrons holding great potential in a wide range of applications. By varying the nature and the structure of a functional moiety in the focal point, one can achieve the controllable self-assembly of dendrons into supramolecular associates
[29][32][33][34]. Such molecules, which are in fact Janus-type particles, expand the functionality of dendrimers for various applications. For instance, amphiphilic dendrons have been shown to deliver chemotherapy drugs
[35][36][37] and anti-cancer siRNAs
[38][39][40] in tumors, thus efficiently suppressing tumor growth. Controllable assembly of complex supramolecular structures mimicking the cell surface
[41], along with the possibility to decorate them with functional moieties, nanoparticles, or (bio)macromolecules
[42] suggests that amphiphilic dendrons are highly emerging for designing precisely engineered biomaterials.
Most dendrimers currently in use for biomedical applications are composed of organic branches and generally mimic biopolymers: poly(amidoamine) dendrimers (PAMAM), poly(propylene imine) dendrimers (PPI), and poly(L-lysine) dendrimers (PLL) etc.
[3][43][44][45][46][47][48][49][50][51][52][53]. Furthermore, there are dendrimers containing main group heteroatoms as core components and branching points
[5], such as silicon
[54] or phosphorus
[55], as well as their combinations with organic branches (PAMAM-organosilicon dendrimers (PAMAMOS)), phosphorus-viologen dendrimers
[56], etc.
The major advantage of dendrimers and dendrons compared to other types of synthetic polymers is the precisely defined molecular structure
[57], which facilitates their certification for use in drug formulations and medical devices. Furthermore, dendrimers and dendrimer-based nanoformulations are efficiently taken up by different cell types through clathrin-mediated endocytosis pathway
[58]. In order to improve biocompatibility and biological activity, as well as achieve active targeting, dendrimers can be functionalized by various chemical and biological entities, such as polyethylene glycol, folate, signal peptides, ligands of cell surface receptors, etc.
[57]. Reporter groups (fluorescent dyes, radioactive, or MRI-active groups) are introduced to monitor the biodistribution of dendrimer-based formulations. Rich dendrimer chemistry makes it possible to flexibly choose conjugation methods. The presence of multiple functional groups on the dendrimer surface gives room for obtaining multifunctional agents bearing different types of functional groups in a single construct. This helps decrease side effects of formulations
[59].
Bioactive molecules can be either conjugated or complexed to dendrimers, and the complexation may involve hydrophobic or electrostatic interactions. In the case of nucleic acid therapeutics, they are usually bound by electrostatic interactions to dendrimers bearing cationic groups on the surface to form polyelectrolyte complexes
[60].
2. The Use of Dendrimers for Biomedical Applications
Distinct dendrimer-based constructions have been subjected to clinical trials; their safety and efficacy have been demonstrated in Phases 1–3, although some studies are still ongoing. Table 1 lists the dendrimer-based products involved in clinical trials, according to the clinicaltrials.gov database. These examples clearly highlight the growing employment of dendrimer-based drug delivery systems in the pharmaceutical industry.
Table 1. Dendrimer-based drugs in clinical trials.
The first dendrimer-based, anti-microbial drug approved for use in humans was Vivagel® (SPL7013) (Starpharma, Abbotsford, Australia), a poly-L-lysine dendrimer derivastive used as a topical microbicide for the prevention and treatment of bacterial vaginosis (BV). There have been at least 13 clinical studies of this product, with the first clinical trial completed in 2004. SPL7013 is a polyanionic dendrimer with a benzylhydramine lysine core and has 32 naphthalene disulfonate surface functionalities (Figure 2). This product also provides the prevention of genital herpes (HSV-2), HIV, and other sexually transmitted infections (STIs). Studies have shown that SPL7013 is generally well tolerated with minimal side effects [82][83][84][85][86][87][88][89][90][91]. SPL7013, in the form of a nasal spray called VIRALEZE, has also undergone a first growth phase as registered in the Australian and New Zealand Clinical Trials Registry [92].
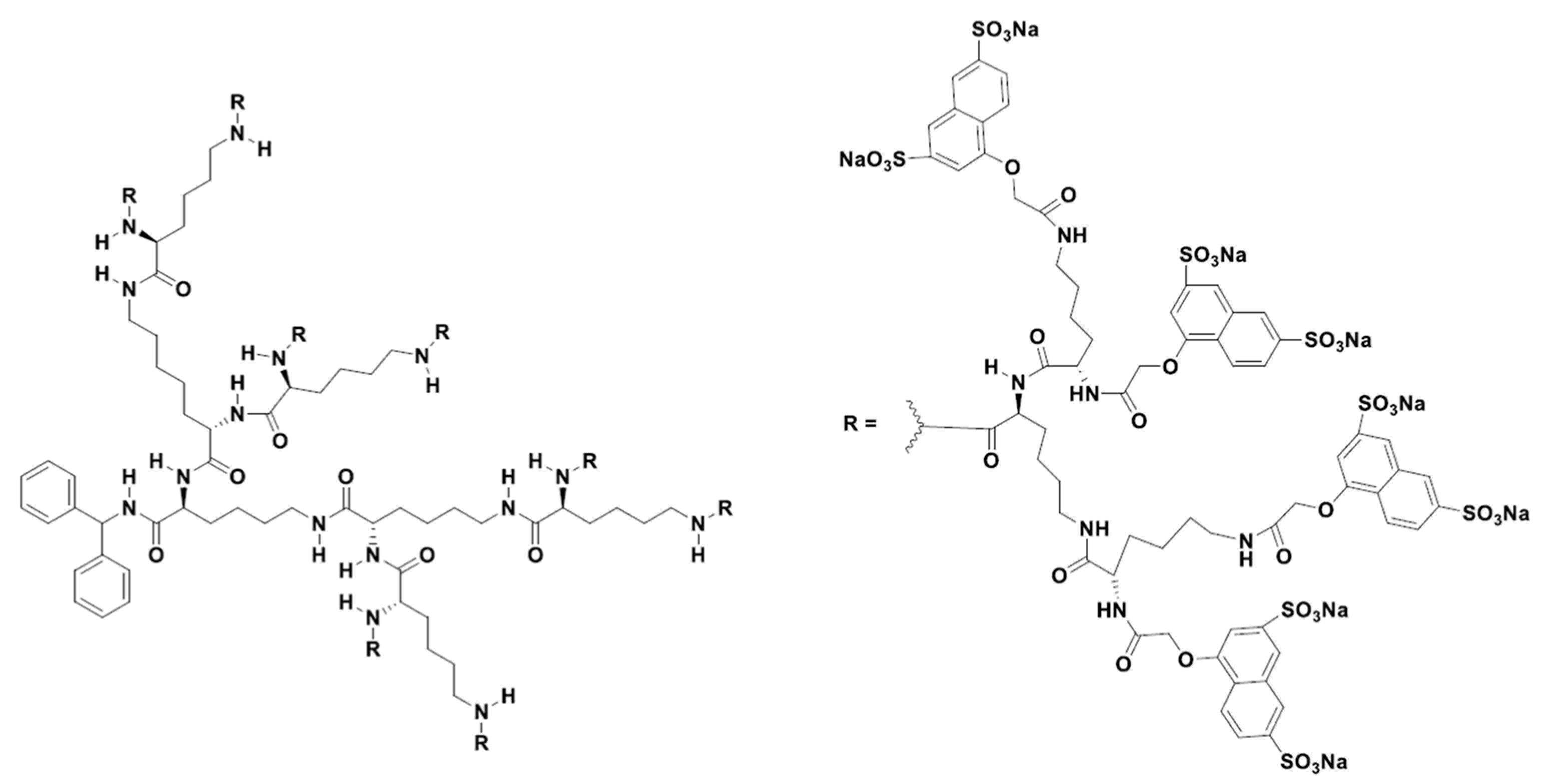
Figure 2. Two-dimensional chemical structure of VivaGel® [92].
Another example of a poly-L-lysine dendrimer developed by Starpharma and AstraZeneca is AZD0466. This is a fifth generation poly-L-lysine dendrimer, a highly optimized molecule containing the anti-cancer drug AZD4320, and a PEGylated poly-L-lysine dendrimer. AZD0466 belongs to a new class of oncology drugs that provide efficient delivery of a dual Bcl-2/xL inhibitor with an optimized release profile that is designed to reduce the potential for toxicity associated with dual Bcl-2/xL inhibition [93][94][95][96]. The AZD0466 construct is currently undergoing simultaneous phase1/phase 2 clinical trials in patients with advanced hematologic malignancies as monotherapy or in combination with certain combination therapies, such as antifungals.
Another example is a fifth-generation poly-L-lysine-based dendrimer named ImDendrim, which has proven to be suitable for encapsulating rhenium and radioactive 188Re. This potential radiopharmaceutical agent showed antitumor activity in liver cancer in mice
[97]. The clinical study of this drug concerns patients with an inoperable liver tumor that is resistant to other classical chemotherapy.
PAMAM dendrimers
[44][98][99][100][101][102][103] are the most widely used dendrimers. In their native form, they can be easily modified as desired. However, low storage stability has long precluded the use of PAMAM dendrimers in clinical trials
[104]. However, PAMAM dendrimers have recently passed clinical trials proposed by Ashvattha Therapeutics.
The first PAMAM dendrimer-based injectable is OP-101, a covalent conjugate of generation 4 hydroxyl-terminated poly(amidoamine) dendrimer and N-acetylcysteine (NAC) developed by the American firm Ashvattha Therapeutics and its subsidiary Orpheris, Inc. Safety trials for intravenous and subcutaneous injections in healthy volunteers began in 2018 and continued into 2020. The study is not yet complete, but preliminary results indicate that OP-101 is well tolerated and may have the potential to treat systemic inflammation and neuronal damage, reducing the disease severity and mortality in patients
[105].
Another drug from the same company, Ashvattha Therapeutics, with the open name D-4517.2, is a hydroxyl dendrimer, a VEGFR tyrosine kinase inhibitor. Initial studies have shown that this drug is safe and well tolerated, with only a few patients experiencing mild transient reactions at the injection site associated with the technique
[106].
Two clinical trials against COVID-19 were conducted in 2021, both based on the same cationic peptide dendritic structure containing lysine as branch elements, named KK-46, the structure of which is shown in Figure 3.
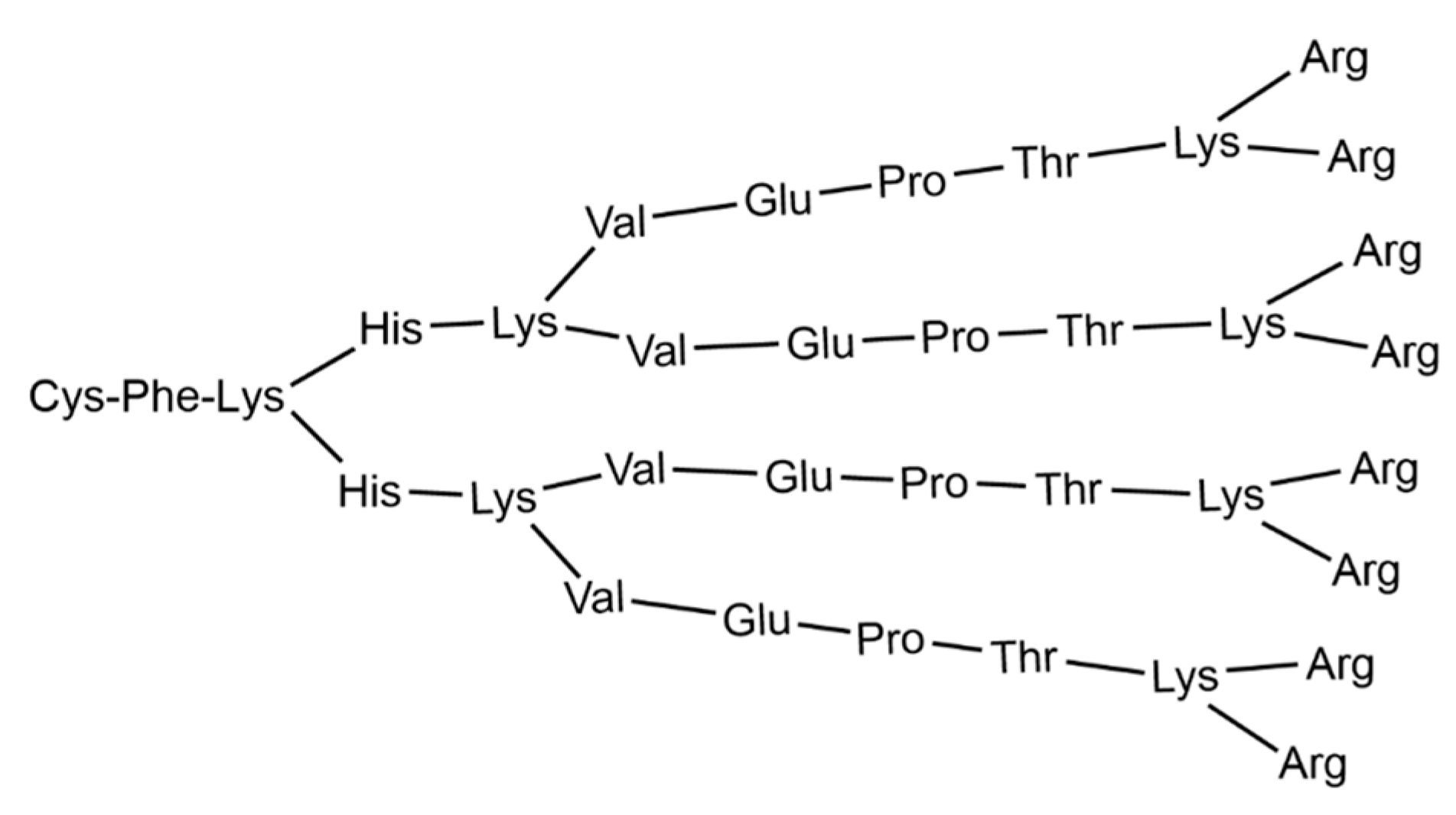
Figure 3. Structure of the cationic peptide dendrimer KK-46, usable as carrier of siCoV [107].
This compound, developed by the Institute of Immunology of the Federal Medical and Biological Agency of Russia, in collaboration with the St. Petersburg Research Institute of Vaccines and Serums, is used as a carrier of siRNA, modified to suppress SARS-CoV-2 (siCoV) by inhibiting its replication [108]. The association of KK-46 with siCoV has been named MIR 19® [109]. Based on preclinical data, the researchers hypothesized that SARS-CoV-2 inhibition by siCoV/KK46 could potentially reduce lung inflammation, thereby improving treatment outcomes. According to the test results, the drug was registered and introduced into civil circulation by the Ministry of Health of the Russian Federation on 22 December 2021 LP-007720 as a direct-acting antiviral agent [110].
3. Conclusion
In summary, distinct dendrimer-based constructs have been clinically tested and have been shown to be safe and effective in Phases 1–3, with some studies still ongoing. Table 1 lists dendrimer-based products in clinical trials, according to the Clinictrials.gov database. These examples clearly highlight the growing use of dendrimer-based drug delivery systems in the pharmaceutical industry.
Thus, dendrimers represent a useful platform for the development of safe vaccines with new properties and application potential and will also be useful for basic research on the mechanisms underlying the induction and control of immunity.