1. Wound Dressings as Polymer Membranes
The matrices of commercially available wound dressings (WDs) are made of one or more natural polymers, modified natural polymers or synthetic polymers. These polymeric matrices, which commonly have the form of a sheet or pad, act as selective membranes, controlling mass transport of a particular species across them. In particular, they prevent the transport of microorganisms and other foreign agents present in the outside environment to the wound, while allowing transport of water vapor, oxygen and carbon dioxide to or from the outside environment. Membranes for WDs are most commonly produced by (i) weaving or knitting, (ii) non-weaving and (iii) phase inversion (Figure 1).
Figure 1. Methods commonly employed in the preparation of membranes for wound dressings.
In the production of membranes for WDs by weaving, a fabric-like structure is achieved by interlacing two distinct sets of fiber yarns at right angles, with the aid of a weaving machine; in knitting, the yarn interlacing pattern is more elaborate and it is achieved by yarn interlocking
[1].In non-weaving, fabrics are made directly from the polymer or from its fibers, without the need for yarn. The methods most commonly employed in the production of non-woven fabrics for WDs are hydroentanglement (also known as spunlacing) and electrospinning. In hydroentanglement, fibers are entangled as a result of the action of a curtain of fine, high-pressure water jets that penetrate fiber webs and hit a conveyor belt below the fiber webs, bouncing back through the fiber webs
[2]). In electrospinning, a polymer solution or melt is forced through a narrow needle in a high-voltage electrical field created between the needle and a collector surface. This solution or melt is, thus, converted into a charged polymer jet that is accelerated towards the collector by the electrical field. Solvent evaporation occurs and a non-woven web composed of fibers with diameters in the nanometer range is deposited on the collector’s surface
[3]. In addition, a natural non-woven membrane of cellulose is produced by bacteria such as
Acetobacter xylinum and designated bacterial cellulose
[4]. Currently, non-wovens are favored in relation to wovens, since they are faster and more economical to produce
[1][5]. In phase-inversion methods, a polymer solution, composed of a solvent (continuous phase) and a polymer (non-continuous phase), is converted into a polymer membrane, commonly after casting on a surface
[6][7]. In the resulting membrane, the component phases are inverted with respect to the polymer solution, with the polymer becoming the continuous phase, and the solvent in the membrane’s pores becoming the non-continuous phase. Phase inversion can be accomplished by several methods, including the following (
Figure 1):
i) Precipitation by immersion in a non-solvent, in which precipitation occurs due to the exchange of the solvent in the polymer solution by a non-solvent miscible with the solvent present in a coagulation bath;
ii) Precipitation in non-solvent vapor, in which phase inversion occurs inside a closed chamber in which the non-solvent is in the vapor phase and causes precipitation of the polymer in the solution;
iii) Solvent evaporation (also known as solution casting), in which the solvent of a polymer solution cast on a substrate is allowed to evaporate or is removed by heating the polymer solution in an oven;
iv) Thermally induced phase separation, in which a solvent that dissolves the polymer at a given temperature loses this ability when the temperature is decreased, with the solvent being subsequently removed by extraction, evaporation or lyophilization (freeze-drying); and
v) Crosslinking, in which a polymer in solution is insolubilized by the formation of chemical bonds or by physical interactions between its molecular chains.
2. The Antiseptic Polyhexanide (PHMB)
PHMB is a synthetic polydispersed mixture of polymers that has been widely employed as an antiseptic since the 1950s. In addition to its use in wound antisepsis
[8], PHMB has found a wide range of other applications, including the following: (i) disinfection of medical utensils and trays, food and non-food contact surfaces, animal drinking water, recreational water, filters and toilets, (ii) preservative in contact lens solutions, cosmetics and personal care products, wet wipes, fabric softeners, and hand and mouth washes, (iii) preservation of hides and skins, (iv) anti-odor finishes in textiles and (v) antimicrobial high-pressure paper–phenol-formaldehyde resin laminates
[9][10][11]. It has also found other types of applications, such as in gene delivery
[12], DNA capture for biological threat surveillance
[13], antibiofouling filtration membranes
[14], dental-plaque control
[15], cotton fabric dyeing
[16], fuel cells
[17], optoeletronics
[18], CO
2 capture and sensing
[19], uranium extraction from seawater
[20] and sewage dewatering
[21]. As a preservative in cosmetic products, it has been considered by the European Commission’s Scientific Committee on Consumer Safety (SCCS) as safe for consumers up to a concentration of 0.1%
[9].
In terms of antimicrobial spectrum, PHMB is effective against a wide range of both Gram-positive and Gram-negative bacteria, including difficult to control bacterial strains, such as methicillin-resistant
Staphylococcus aureus (MRSA) and vancomycin-resistant
Enterococci (VRE)
[22], although a single polyhexanide decolonization course may not be sufficient to eradicate MRSA in clinical cases
[23]. Of note, no PHMB-resistant MRSA could be isolated in clinical cases in which decolonization by PHMB was attempted
[23]. On the contrary, decreased susceptibility to PHMB could be artificially induced in vitro by repeated exposure to very low PHMB concentrations (0.1–1 µg/mL or 0.00001–0.0001%). This reduced susceptibility was associated with specific genomic alterations
[24]. PHMB is also active against yeasts and other fungi
[25], amoeboids such as
Acanthamoeba spp.
[26], enveloped viruses, such as
Herpes simplex [27] and human immunodeficiency virus type I (HIV-I)
[28], and non-enveloped viruses
[29]. PHMB has minimum inhibitory concentration (MIC) and minimum bactericidal concentration (MBC) values against bacteria commonly found in wounds in the low µg/mL range, varying from 0.5 to 8 µg/mL
[31][32][33]. MIC and MBC values may be higher when bacteria form biofilms, as suggested by the results of a study that showed that the antimicrobial activity of a commercial PRWD was higher in a planktonic cell model than in an immobilized cell model used to mimic biofilms
[30].
When administered topically to wounds, PHMB is well tolerated, showing low cytotoxicity and poor skin penetration [9][25][34], and also promotes wound healing, by favoring tissue granulation on the wound surface [8]. In humans, repeated prolonged exposure to PHMB at concentrations of 2% may cause sensitization [35], most notably in patients with suspected allergic contact dermatitis [36]. However, this PHMB concentration is 4-times higher than the highest concentration employed for antisepsis. For instance, the wound irrigation/cleaning solution Serasept® (Serag-Wiessner GmbH & Co. KG, Naila, Germany) contains PHMB at a concentration of 0.03% or 0.04%, and Prontosan®, in all its three formats (solution, gel or spray; B. Braun Melsungen AG, Germany), contains 0.1% PHMB (in combination with a betaine antiseptic). In rats, acute skin toxicity was only observed for a concentration of 5% [25].
PHMB is commonly synthesized as a hydrochloride salt by polycondensation of 1,6-hexanemethylenediamine and sodium dicyanamide, but can also be synthesized through other routes (
Figure 2). The polycondensation process generates a mixture of oligomers, composed of a minimum of 2 and a maximum of 40 to 42 repeating units, with an average degree of polymerization of 12. The oligomer chains can have different terminal groups, including guanidino, cyanoguanidino, amino and cyanoamino groups (
Figure 3)
[37][38][39].
Figure 2. Synthesis of poly(hexamethylene biguanide) hydrochloride (PHMB.HCl) via polycondensation of 1,6-hexamethylenediamine and sodium dicyanamide. n = 2–42.
Figure 3. Terminal groups that may occur in poly(hexamethylene biguanide) (PHMB) chains. (a) Amino; (b) cyanoguanidino; (c) guanidino; and (d) cyanoamino.
Due to the occurrence of polymerization, depolymerization and repolymerization reactions during its synthesis, a polydisperse polymer is obtained. Reported molecular weight (MW) values range from below 500 g/mol up to ca. 4200 g/mol
[9], and MW theoretical estimates point to ca. 1400 g/mol for its number-average MW and ca. 2600 g/mol for its weight-average MW
[39]. Of note, a positive correlation was observed between polymer chain length and antiseptic/antimicrobial activity
[40]. PHMB is structurally related to chlorhexidine (CHX), another synthetic biguanide-based antiseptic that possesses two biguanide units linked by a hexamethylene chain, with 4-chlorophenyl as the terminal group.
PHMB is also structurally related to naturally occurring antimicrobial peptides (AMPs)
[22], which similarly exhibit a broad spectrum of activity against bacteria, viruses and fungi. These peptides are part of the innate immune response, being produced by cells within wounds, such as keratinocytes and neutrophils
[37]. Chemically, AMPs and PHMB can both be described as polycations, the former containing positively charged amino acids among a larger proportion of hydrophobic amino acids, the latter containing repeating biguanide units of high basicity (calculated p
Ka values above 13
[41]), attached to flexible hydrophobic hexamethylene chains (
Figure 2). The mechanisms of action of PHMB and AMPs are also related, both relying upon their binding to the negatively charged bacterial cell membranes and walls, ultimately inducing cell lysis through the disruption of membrane integrity. In Gram-positive bacteria, this negative electrical charge is due to the presence of anionic phospholipids, teichoic acids and polysaccharides, whereas it is due to lipopolysaccharides in Gram-negative bacteria. In PHMB, the positively charged biguanide units are able to displace Ca
2+ ions that stabilize the lipid bilayer and its hydrophobic hexamethylene portion is able to sink into the cell membrane, resulting in increased membrane permeability and, ultimately, membrane disruption
[37][42][43][44]. Complexation with negatively charged phospholipids also impairs the function of ion pumps, receptors and enzymes in the cell membrane
[37]. In addition, PHMB is able to enter the bacterial cell and arrest cell division by binding and condensing bacterial chromosomes
[45]. The specificity of PHMB to bacterial cells can be partially explained by the relatively neutral surface of eukaryotic cells, due to the presence of phosphatidylcholine-based phospholipids and other zwitterionic lipids, as well as cholesterol. PHMB is still able to enter the eukaryotic cell, but no damage to the cell membrane occurs. Additionally, it does not penetrate the nucleus, being trapped within endosomes, which are absent in bacteria
[45].
In terms of physical and chemical properties, PHMB exhibits good thermal and hydrolytic stability
[46][47]. It is highly soluble in water and methanol (ca. 40%), but only sparingly soluble in ethanol (ca. 0.5%) and in some other organic solvents, such as acetone, acetonitrile, dichloromethane and toluene
[9]. In water, but not in ethanol
[39], the alternating sequence of hydrophilic (biguanide) and hydrophobic (hexamethylene) segments may give rise to polymeric micelles of the core/shell type, in which the hydrophobic segments point towards the center of a sphere (core), while the hydrophilic groups point outwards (shell). However, at the concentrations normally employed for wound antisepsis and in commercial PHMB-releasing WDs (PRWDs), which is between 0.01% and 0.05%
[48], micelle formation is not expected, as PHMB’s critical micellar concentration (CMC) is considerably higher (0.02–0.05 M in water
[39], equivalent to ca. 50–130 mg/mL or 5–13%). On the other hand, in water and at concentrations below its CMC, PHMB may form aggregates larger than the above-mentioned micelles, with particle dimensions in the micrometer range
[39]. When in aqueous solutions of monovalent counterions, molecular dynamics simulation studies suggested that PHMB can self-assemble in a compact, ordered hairpin-like structure
[49] of the type that occurs in biological polyelectrolytes, such as RNA
[50]. With increasing salt concentration, these hairpin-like structures can collapse further into three- or five-folded structures. Neither ions nor water molecules mediate this self-assembly, which, counterintuitively, is driven by like-charge pairing of free biguanidinium ions
[51].
3. Loading of PHMB into Membranes
There are three main approaches to load a drug into a membrane with the aim of creating drug-releasing membranes, which are as follows (Figure 4):
i) Soaking, in which the membrane to be loaded is immersed in a drug solution for a certain period of time, usually under agitation;
ii) Impregnation, in which a drug solution is added to the membrane, being fully absorbed; and
iii) Addition, in which the drug is added to the formulation used to prepare the membrane.
Drug loading by soaking is the most straightforward method, but the loading yield can be low, as drug entry into the membrane occurs by diffusion across a concentration gradient. As such, some of the drug present in the solution will not be loaded. Loading yields depend not only on drug concentration, but also on other loading conditions, such as solvent, temperature, pH, ionic strength and time, as well as on drug/membrane interactions, molecular size of the drug, surface area, porosity and pore size of the membrane. In drug loading by impregnation, a contact between the membrane and a drug solution occurs but instead of occurrig by immersion of the membrane into the drug solution, the solution is added to the membrane until the membrane becomes fully saturated with drug solution. In drug loading by addition, the drug is added to the formulation employed to prepare the membrane. As such, it must be able to withstand the conditions employed in membrane preparation and, usually, it has to dissolve in the formulation. With this method, non-toxic materials and reagents have to be employed, since extensive drug loss would occur if the final drug-loaded membrane was washed to extract cytotoxic leachables. PHMB is amenable to all of these three membrane-loading approaches, due to its good hydrolytic, thermal and photo stabilities [46][47][52], solubility in water and ability to be dissolved in some organic solvents [9][53][54][55].
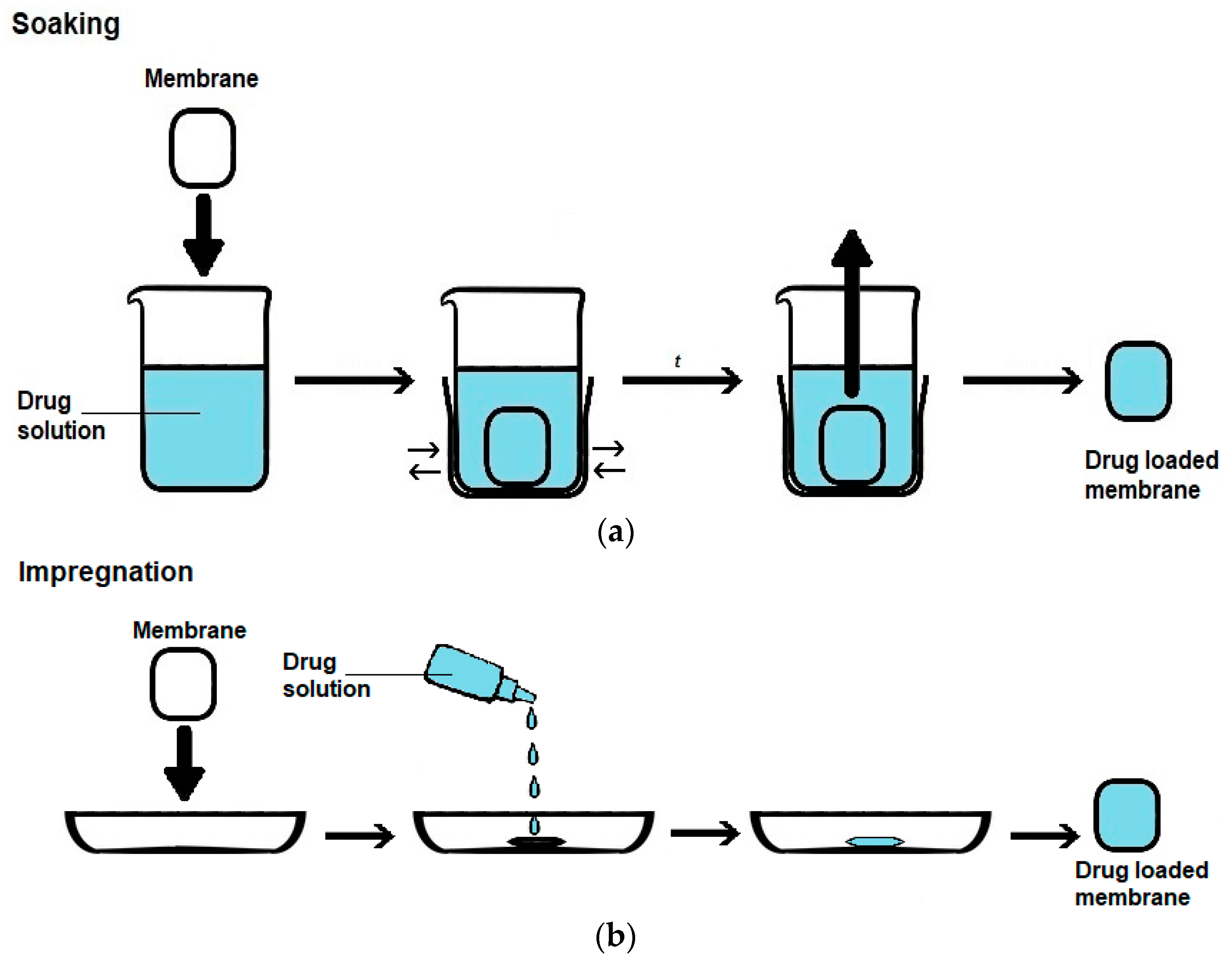
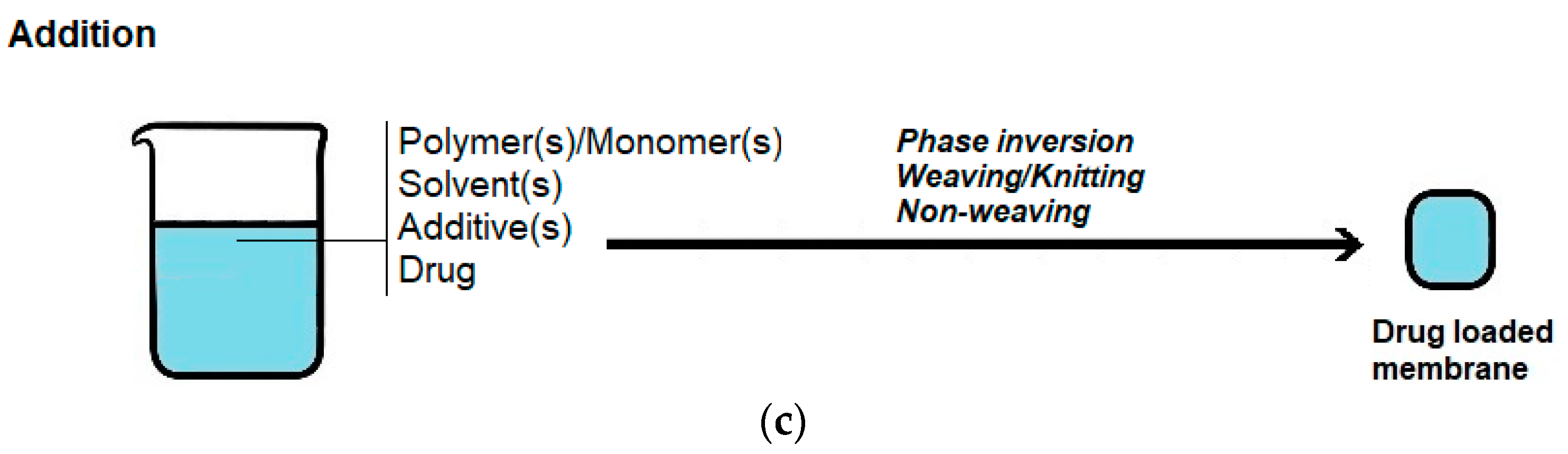
Figure 4. Schematic representation of the methods employed in loading a drug into a membrane. (a) Drug loading by soaking. (b) Drug loading by impregnation. (c) Drug loading by addition.
4. Commercially Available PHMB-Releasing Wound Dressings (PRWDs)
A first proposal for the medical use of a PHMB-releasing membrane can be found in a 1984 patent filed by Johnson & Johnson
[56] for the invention of a non-woven, cellulose-based PHMB-containing fabric, to be used in surgical procedures as a means to isolate the surgical area from the rest of the body and other sources of contamination. This surgical drape was prepared by spraying the non-woven fabric with PHMB dissolved in a solution of a fabric binder. Approximately two decades later, PHMB was successfully introduced into wound management in the form of an antimicrobial dressing (AMD). This success was due, at least partially, to PHMB’s high stability and broad antimicrobial spectrum
[46][47].
Table 1 summarizes some of the characteristics of commercially available PRWDs. As can be observed, the matrices of these PRWDs were made from a wide variety of polymers, either of natural origin (cotton, viscose, rayon, bacterial cellulose and extracellular matrix biopolymers) or synthetic (polyesters and polyurethanes (PUR)). Whenever this information was disclosed, PHMB loading was always by impregnation, employing PHMB solutions of concentrations ranging from 0.1% to 0.5%, and antibacterial activity lasted for up to 3–7 days. The biological properties of some of the PRWDs were assessed in reported studies. ActivHeal
® PHMB was employed in 32 patients with hard-to-heal wounds (such as post-operative surgical wounds and leg, diabetic and pressure ulcers), resulting in reduced bacterial load and pain and in the effective management of exudate levels
[57]. CelluDress-PHMB, a more recent addition to the market, was associated with good outcomes in the treatment of non-healing venous leg ulcers
[58]. In clinical cases, the Excilon
™ AMD drain sponge extended the number of days during which tracheostomy sites remained free of pathogens, compared to a non-AMD dressing, while normal flora remained unaffected
[59]. Kendall™ AMD foam dressing resisted in vitro MRSA colonization within the WD more efficiently than an equivalent, non-impregnated WD
[60]. In vivo, this PRWD performed well in a small-scale (25 subjects), retrospective uncontrolled environment study, with improvement in all wounds
[61], and its employment in a multicenter, prospective, double-blind, pilot, randomized controlled clinical trial that involved 45 patients with chronic wounds resulted in higher reductions in bacterial burden, pain and wound size than the employment of a similar, non-AMD, PUR-based WD (Kendall™ foam dressing)
[62]. Kerlix™ AMD inhibited the in vitro proliferation, both within and underneath the WD, of Gram-negative and Gram-positive bacteria
[63], including antibiotic-resistant bacterial strains, such as VRE
[64] and MRSA
[63][65]. In humans with difficult-to-heal wounds and in a wound-healing porcine model, it decreased the bacterial burden, compared to a similar WD without PHMB, while sparing the normal skin flora (only assessed in the porcine model)
[66][67]. In the same porcine model, no adverse effects on the wound epithelization rate were observed
[68]. However, in another study that also employed a porcine wound-healing model, enhancement or lengthening of the wound inflammatory response occurred, resulting in bleeding when changing the WD
[69]. Suprasorb
® X + PHMB was assessed in case studies of patients with traumatic and surgical wounds, pressure and leg ulcers, and burns, where it reduced infection, exudate levels and pain, while increasing granulation tissue and epithelialization and improved necrotic tissue debridement
[70]. In a co-culture of
S. aureus and keratinocytes, Suprasorb
® X + PHMB protected keratinocytes from damage by reducing the number of viable bacterial cells
[71]. In a prospective, randomized, controlled single-center study designed to compare its clinical efficacy with that of a silver-sulfadiazine cream and dressing in 60 patients with partial-thickness burns (i.e., second-degree burns), no difference in healing times was found, but Suprasorb
® X + PHMB showed better and faster pain reduction and the need for fewer WD changes
[72]. Additionally, in a prospective, randomized, controlled, multicenter trial with 25 patients per treatment group, it was found that Suprasorb
® X + PHMB and a silver-containing WD were both effective in reducing pain and bacterial burden in the treatment of critically colonized and locally infected wounds, but Suprasorb
® X + PHMB exhibited superior antimicrobial properties
[73]. Finally, Telfa™ AMD was assessed in a prospective, randomized controlled study that comprised 32 patients treated for skin burns with skin grafts. In this study, patients treated with this AMD exhibited shorter re-epithelialization times and lower pain levels than the patients treated with Bactigras
®, a commercial AMD with the antiseptic CHX
[74].
Table 1. Commercially available PHMB-releasing wound dressings (PRWDs), in alphabetical order.
Commercial Name |
Format |
Material |
PHMB Loading Mode/[PHMB] |
Duration of Antimicrobial Activity |
Sources |
ActivHeal® PHMB |
Foam, pad |
Polyurethane |
Impregnation/nd |
7 days |
Advanced Medical Solutions, Ltd., Winsford, UK; [75] |
CelluDress-PHMB |
Pad |
Polyester + viscose |
nd 1 |
nd |
Medicareplus International Ltd., Wembley, UK; [58] |
CurityTM AMD Antimicrobial Woven Sponges |
Sponge, strip |
Cotton |
Impregnation/0.2% |
Up to 3 days |
Cardinal Health, Dublin, OH, USA; [76] |
DracoFoam PHMB |
Foam |
Polyurethane |
nd |
Up to 7 days |
Dr. Ausbüttel & Co. GmbH, Dortmund, Germany; [77] |
ExcilonTM AMD |
Sponge, gauze |
Polyester + rayon |
Impregnation/0.2% |
Up to 3 days |
Cardinal Health, Dublin, OH, USA; [76] |
Fitostimoline® Plus Gauze |
Gauze |
nd |
nd 2 |
nd |
Farmaceutici Damor S.p.A., Napoli, Italy |
Gemcore360°TM PHMB Foam Border Dressing |
Foam |
nd |
Impregnation/nd |
Up to 7 days |
GEMCO Medical, Hudson, OH, USA; [76] |
Gemcore360°TM PHMB Non-Adhesive Foam Dressing |
Kendall™ AMD |
Foam, disc |
Polyurethane |
Impregnation/0.5% |
Up to 7 days |
Cardinal Health, Dublin, OH, USA; [76] |
Kerlix™ AMD |
Gauze, sponge |
Cotton |
Impregnation/0.2% |
Up to 3 days |
McKesson PHMB Hydrophilic Foam Dressing |
Foam |
Polyurethane |
Impregnation/0.8–1% |
Up to 7 days |
McKesson Medical-Surgical, Irving, TX, USA; [76] |
PuraPly® AM |
Sheet, disc |
Crosslinked ECM |
nd |
nd |
Organogenesis Inc., Canton, MA, USA; [78] |
Sterilux® AMD Antimicrobial Gauze |
Gauze, sponge |
Cotton |
nd/0.1% PHMB (+0.02% BKC) |
Up to 7 days |
Hartmann USA, Inc., Rock Hill, SC, USA; [76] |
Suprasorb® X + PHMB |
Sheet |
Bacterial cellulose |
nd/0.3% |
nd |
Lohmann & Rauscher GmbH & Co. KG, Neuwied, Germany; [79] |
Suprasorb® P + PHMB |
Foam |
Polyurethane |
nd |
Telfa™ AMD |
Pad, island |
Cotton |
Impregnation/0.2% |
Up to 3 days |
Cardinal Health, Dublin, OH, USA; [76] |
TielleTM PHMB |
Foam |
Polyurethane |
Impregnation/nd |
nd |
3M/KCI, St. Paul, MN, USA |
nd–Not disclosed by the manufacturer. 1 Contains PHMB complexed with an undisclosed surfactant. 2 Contains PHMB and an aqueous extract of Triticum vulgare. BKC–benzalkonium chloride; ECM–extracellular matrix.