2. Modulation of NRF2/KEAP1 Signaling Pathway by α,β-Unsaturated Moiety-Bearing Compounds
Although carbonyl and sulfonyl groups are both electron-withdrawing, the sulfonyl group tends to exhibit more of an electron-withdrawing effect than the carbonyl group. It is therefore preferred to the carbonyl group as a leaving group in nucleophilic substitution reactions
[15]. However, there is a more efficient delocalization with carbonyl groups than with sulfonyl groups
[15]. The beta-carbon of the α,β-unsaturated carbonyl, sulfonyl and sulfinyl groups is the most reactive electrophilic atom of these groups
[16][17]. There is electron deficiency at the beta-carbon of the α,β-unsaturated carbonyl, sulfonyl and sulfinyl groups due to the electron-attracting and delocalizing activity of these moieties, and this property accounts for their electrophilicity
[18][19][20]. The electrophilic character is transmitted to the beta-carbon of the double bond following the conjugation of a double bond to a carbonyl, sulfonyl and sulfinyl group in α,β-unsaturated systems. This phenomenon favors 1,4-addition reaction
[21]. The resonance description of the transmission of electrophilicity to the beta-carbon (
Scheme 1)
[21] confirms that the beta-carbon represents the electrophilic atom at which nucleophilic thiols of cysteines are most likely to attack. Thus, the beta-carbon of α,β-unsaturated carbonyl, sulfonyl, sulfinyl groups and that of NRF2 activators containing them (
4–
7) are indicated in
Scheme 2. The nucleophilic attack of the α,β-unsaturated structural systems by thiols of the KEAP1 cysteine residues occurs via the reaction mechanism represented in
Scheme 3.
Scheme 1. A resonance description of the transmission of electrophilic character to the beta-carbon of α,β-unsaturated carbonyl system (1).
Scheme 2. A schematic view of the electrophilic beta-carbon (indicated with asterisks) of α,β-unsaturated carbonyl (1), sulfonyl (2), sulfinyl (3) and some NRF2-activating compounds containing these α,β-unsaturated moieties (4–7). The asterisks represents the point at which thiols of cysteines are most likely to attack.
Scheme 3. Reaction mechanisms of α,β-unsaturated (A) carbonyl, (B) sulfonyl and (C) sulfinyl moieties. The nucleophilic attack of the thiol of the KEAP1 cysteine residues on the β carbon of the carbonyl group is followed by 1,4-addition reaction in which the thiol bonds to carbon in position 1 and hydrogen bonds to oxygen in position 4. It undergoes tautomerization to form adducts which facilitates the nuclear translocation of NRF2 (A). The reaction of α,β-unsaturated sulfonyl (B) and α,β-Unsaturated sulfinyl (C) with thiols of the KEAP1 cysteine residue also enable NRF2 translocation.
The electrophilic modification of the cysteine residues of cytosolic proteins by α,β-unsaturated carbonyl, sulfonyl and sulfinyl groups has been found to affect transcriptional regulation of the NRF2 signaling pathway
[4][7][16]. The NRF2 pathway is likely the most sensitive pathway for electrophilic thiol-modifying molecules due to the presence of several highly reactive cysteine residues in KEAP1
[22]. Under homeostatic conditions, there is a continuous degradation of NRF2 protein in the cytoplasm by a complex of E3 ubiquitin ligase containing the regulatory cysteine-rich KEAP1 protein
[23][24]. However, under oxidative stress, electrophilic α,β-unsaturated carbonyl, sulfonyl and sulfinyl compounds modify Keap1
[9][17][25]. They react with some cysteine residues of KEAP1 to form adducts that create a non-functional KEAP1 complex, thus favoring the nuclear translocation of newly translated NRF2 and facilitating transcriptional induction of NRF2–dependent genes
[26][27][28][29]. Many cysteines of KEAP1 are modified by different electrophiles
[26][27][30][31][32][33]. KEAP1 is a cysteine-rich protein possessing 27 and 25 cysteine residues in the human and mouse proteins, respectively. This “cysteine-code” controls KEAP1 activity. Cysteines Cys-151, Cys-273 and Cys-288
[34][35] appear to be the most susceptible to electrophilic reaction
[33][36]. Based on the functional necessity of these three cysteine residues in the maintenance of KEAP1 ability to inhibit NRF2 accumulation, chemical inducers of NRF2 were categorized into four classes in relation to the cysteine on which they act
[33], namely, class I (Cys151preferring), class II (Cys288 preferring), class III (Cys151/Cys273/Cys288 collaboration preferring) and class IV (Cys151/Cys273/Cys288 independent). Other sensitive cysteines are Cys-226, Cys-434 and Cys-613. Thus, considering the distinct patterns of adduct formation for each chemical inducers of NRF2, the set of optimal acceptor thiols that are functional and convert KEAP1 from the active to the inactive state should be determined.
The NRF2 activation mechanism of α,β-unsaturated moieties is represented in
Scheme 4. The α,β-unsaturated sulfonyl group (
2) acts as a 2 donor and a Michael acceptor in addition reactions
[37]. The stability of the α,β-unsaturated sulfonyl and sulfinyl systems needs to be understood. The equilibrium of these functionalities can be attributed to factors such as the interaction of the α,β-double bond with the d-orbitals of sulfur in addition to the inductive effects of the sulfonyl and sulfinyl groups. In the α,β-unsaturated sulfonyl and sulfinyl systems, the double bond stabilizes by interacting with sulfur’s d-orbitals. Inductive effects on the other hand, accounts for the electron withdrawing ability of the α,β-unsaturated sulfonyl and sulfinyl groups at equilibrium in the order sulfinyl < sulfonyl. The stability of the sulfonyl group, especially sulfones, has been linked to the strength of its carbon-sulfur bond. The observed minimal role of resonance effects and the major role of inductive effects suggest that the latter is very important in the stability of these systems. The α,β-unsaturated carbonyl systems are thermodynamically more favored than α,β-unsaturated sulfonyl and sulfinyl systems, while the α,β-unsaturated sulfonyl group is more stable than the α,β-unsaturated carbonyl system
[38][39][40]. Sulfonyl functional group confers dienophilic activity to the double bond attached to it
[41]. The double bond in α,β-unsaturated sulfonyl-containing compounds is activated by the sulfonyl group
[42]. In parallel, Choi et al.
[18] reported that the α,β-unsaturated sulfonyl system is a highly active Michael acceptor for NRF2 activation. The addition of hard nucleophiles to α,β-unsaturated sulfonyl system poses some difficulties due to metalation and conjugate additions occurring as competing reactions
[43]. However, the addition of soft nucleophiles, especially thiols, to the α,β-unsaturated sulfonyl group via an addition reaction is an easy and effective process
[44][45].
Scheme 4. Mechanism of activation of KEAP1-NRF2-ARE pathway by α,β-unsaturated moieties. In pro-oxidant condition, the exposure to electrophilic α,β-unsaturated moieties alters the structure of NRF2/KEAP1 complex, thus inhibiting NRF2 ubiquitination and creating a non-functional KEAP1 complex. As NRF2 is not released by KEAP1, it saturates all binding sites of KEAP1, allowing newly translated NRF2 to bypass KEAP1 and translocate to the nucleus.
3. α,β-Unsaturated Carbonyls
α,β-Unsaturated carbonyl (
1) compounds can be described as organic compounds with the general structure (O=CR)-C=C-R, in which carbonyl functional group is conjugated with an alkene
[46]. For example, enones and enals exhibit vinylogues reactivity pattern which makes them prone to attack by nucleophiles at the beta-carbon
[46]. In α,β-unsaturated carbonyl-based compound, one C-C bond separates the C=C and C=O bonds. The α,β-unsaturated carbonyl functionality is the most reactive substructure of synthetic and natural molecules
[47][48]. The reactivity of this group explains its various pharmacological activities
[48]. α,β-unsaturated carbonyls scavenge free radicals via covalent ligand binding to target proteins. They exhibit significant antioxidant and anti-inflammatory activities by thiol trapping
[48][49][50]. Data have shown that α,β-unsaturated carbonyls react with a wide range of Cys-containing amino acids, proteins and peptides
[20][51]. They exhibit different molecular actions due to localization and concentration in the different targeting of certain Cysteine residues on specific proteins. Experiments performed utilizing KEAP1 mutants have demonstrated that Cys-151, Cys-273 and Cys-288 are most sensitive to electrophilic reactions with the α,β-unsaturated carbonyl group and are essential for KEAP1 to inhibit Nrf2 activity
[52][53][54]. Although few α,β-unsaturated carbonyl compounds such as acrolein and its derivatives are toxic, a good number of them induce adaptive or protective responses, exhibit remarkable NRF2 activity and play important signaling functions
[55][56][57][58]. Several NRF2 activators strongly depend on the presence of the α,β-unsaturated carbonyl moiety for efficacy. The α,β-unsaturated carbonyl functionality is responsible for the reactivity of several NRF2 activators, including flavones and flavonols, and when this structural feature is disrupted, the ability of these compounds to activate NRF2 is completely suppressed. Moreover, the α,β-unsaturated carbonyl group is required by polyphenols to play the role of antioxidant via NRF2 activation. Wu et al.
[59] reported that α,β-unsaturated carbonyl compounds activate NRF2 pathway, and the loss of the α,β-unsaturated carbonyl moiety abrogates the NRF2 activation by these compounds. Molecules containing α-β unsaturated carbonyl groups have been shown to activate NRF2 in a reporter system and normal peripheral blood mononuclear cells
[60].
3.1. Sesquiterpene Lactones
Sesquiterpene lactones are sesquiterpenoids with a lactone ring, commonly obtained from Asteraceae plant family. They are lipophilic solids that serve as a rich source of drugs because of their wide range of biological activities including antioxidant and anti-inflammatory properties
[60][61][62]. Sesquiterpene lactones such as parthenolide (
8), helenalin (
9), alantolactone (
10) and costunolide (
11) have been found to significantly activate the NRF2/KEAP1 signaling pathway in different in vitro cell culture systems
[63][64][65][66]. Experiments performed in rat neuronal cells demonstrated that treatment with sesquiterpene lactones promoted nuclear NRF2 translocation and ARE target genes expression, and that ARE activation was dependent on the number of α,β-unsaturated carbonyl groups present in each compound
[64]. These observations strongly suggest that the bioactivities of sesquiterpene lactones, especially their ability to activate the NRF2 pathway, can be attributed to the presence of the α,β-unsaturated carbonyl unit
[64][67].
3.1.1. Parthenolide
Parthenolide (
8) is an α,β-unsaturated carbonyl-containing sesquiterpene lactone, the most abundant and active electrophilic compound obtained from feverfew plant (
Tanacetum parthenium)
[68][69]. The α,β-unsaturated lactone is reported to be the reactive part of parthenolide, not the epoxide
[58]. The α,β-unsaturated carbonyl group is responsible for the electrophilic nature of parthenolide (
8), which accounts for its ability to undergo Michael addition reaction with biochemical nucleophiles, to covalently modify proteins, and to activate the NRF2 pathway
[70][71]. Kim et al.
[71] reported that the antioxidant and anti-adipogenic effects of parthenolide are associated with NRF2 activation. Parthenolide (
8) inhibits the early stage of adipogenesis, reduces the production of intracellular reactive oxygen species (ROS) and increases the expression of heme oxygenase-1 (HO-1) and NADPH dehydrogenase 1(NQO1) via the activation of the NRF2/KEAP1 signaling pathway
[71]. In a similar study, Kim and co-workers
[72] attributed the anti-obese effects of parthenolide (
8) to its ability to activate NRF2. They reported that parthenolide (
8) suppresses adiposity-induced inflammatory responses, controls the dysregulation of adiponectin and resistin, upregulates HO-1 and promotes nuclear translocation of NRF2 in obesity and related diseases. In summary, parthenolide inhibits obesity and obesity-related inflammatory responses through the activation of the NRF2/Keap1 signaling pathway. Mao and Zhu
[73] reported that parthenolide (
8) increases the expression of NRF2, HO-1 and NQO1 in hydrogen peroxide-induced osteoblasts, thereby preventing apoptosis by the reduction in oxidative stress. Parthenolide (
8) exhibits significant anti-tumor and anti-inflammatory activities, it inhibits inflammatory mediators and the expression of pro-inflammatory cytokines
[74][75]. Additionally, the anticancer activities of parthenolide are linked to its NRF2 activity, in particular it increases the level of glutathione via the activation of the NRF2-ARE signaling pathway
[76][77]. The antioxidant activity of parthenolide is dose-dependent, at low dose (<5 µM), it neutralizes hydrogen peroxide and protects against CD3-induced apoptosis in Jurkat T cells, while at high dose (10 µM) it induces oxidative stress
[78]. Of note, in recent studies aimed at identifying new strategies to overcome chemoresistance and to increase the effectiveness of chemotherapy in cancer, parthenolide was found to suppress mammosphere formation and overexpression of NRF2 and its dependent genes in triple-negative breast cancer cell lines, thereby preventing resistance to doxorubicin and mitoxantrone based on ROS modulation
[79][80]. It was also reported that parthenolide (
8) activates NRF2 and it is selectively cytotoxic to chronic lymphocytic leukemia (CLL)
[59].
3.1.2. Helenalin
Helenalin (
9) is a sesquiterpene lactone obtained from Arnica montana and Arnica chamissonis foliosa containing an α,β-unsaturated carbonyl group that accounts for its anti-inflammatory, antioxidant, anti-cancer and NRF2 activities
[81][82][83][84]. Lin et al.
[85] reported that helenalin (
9) inhibits oxidative stress, enhances ethanol metabolism and therefore attenuates alcohol-induced hepatic fibrosis. Li et al.
[84] demonstrated that helenalin (
9) isolated from Centipede minima (the family Asteraceae) exhibits significant antioxidant activity and anti-inflammatory effects by inhibiting NF-ĸB activation. It ameliorates acute hepatic injury, alleviates hepatocyte apoptosis, restores mitochondrial function and inhibits hepatic inflammatory cytokines. Helenalin (
9) also alleviates lipid peroxidation, reduces ROS and NO production, increases antioxidant enzyme activity and HO-1 activity via activation of the NRF2 signaling pathway
[84].
3.1.3. Alantolactone
Alantolactone (
10) is a sesquiterpene lactone commonly obtained from lnula helenium
L. It contains α,β-unsaturated carbonyl moiety. It exhibits anti-inflammatory, antioxidant, anticancer and antibacterial activities
[86][87][88]. According to Liu et al.
[89], alantolactone (
10) increases the expression and nuclear translocation of NRF2. This implies that the ability of alantolactone (
10) to promote apoptosis and suppress migration in human breast cancer cell line may depend on NRF2 signaling in addition to other pathways such as p38 and NF-ĸB. Soe et al.
[90] reported that the induction of detoxifying enzymes by alantolactone (
10) is mediated by NRF2. Alantolactone (
10) enhances the activity of glutathione and increases the induction of phase II and antioxidant enzymes such as glutathione reductase, heme oxygenase-1 and γ-glutamylcysteine synthase via the NRF2-ARE signaling pathway. It increases the nuclear translocation and activation of NRF2 in murine hepatoma (Hepa1c1c7) cells
[90]. In vitro experiments conducted on human bronchial epithelial Beas-2B and NHBE cells demonstrated that alantolactone is able to prevent cigarette smoke extract (CSE)-induced pro-inflammatory cytokine production, caspase-3 activation and the increased levels of the oxidative stress markers malondialdehyde, ROS and superoxide dismutase. The same study also demonstrated that alantolactone promotes NRF2 nuclear aggregation and HO-1 expression, thus suggesting that this compound inhibits CSE-induced inflammation, apoptosis and oxidative stress by promoting NRF2 activation
[91].
3.1.4. Costunolide
Costunolide (
11) is a sesquiterpene lactone usually obtained from Inula helenium and Vladimiria souliel
[92]. It has been extensively studied due to its numerous biological functions such as anti-inflammatory, antioxidant and neuroprotective activities
[92][93]. Pae et al.
[94] reported that costunolide (
11) reduces inflammation by the up-regulation of HO-1 expression. Furthermore, costunolide (
11) has been reported to improve the level of GSH in tissues and to ameliorate ethanol-induced gastric ulcer through its antioxidant anti-inflammatory activities
[95][96]. Peng et al.
[97] demonstrated that costunolide (
11) prevents oxidative injuries and hinders apoptosis by promoting the nuclear translocation of NRF2, and up-regulating the expression of NRF2 downstream molecules in the neuron-like rat pheochromocytoma cell line (PC12). It upregulates antioxidant genes and reduces cellular ROS levels thus maintaining redox balance in PC12 cells. However, the knockdown of NRF2 reportedly abrogated the cytoprotective activity of costunolide (
11), thus suggesting that its ability to promote neuroprotection is dependent on NRF2 pathway activation. In another study, costunolide (
11) was found to induce HO-1 expression and NRF2 nuclear accumulation, to inhibit pro-inflammatory cytokines and to activate NRF2 in RAW 264.7 macrophages
[94]. Similarly, Mao et al.
[93] reported that costunolide (
11) inhibits lipopolysaccharide and D-galactosamine-induced acute liver injury via NRF2 activation. It also down-regulates KEAP1 gene expression and up-regulates HO-1 and NQO1 gene expressions. Taken together, these results indicate that costunolide (
11) exerts protective effects against acute liver injuries via its antioxidant activity by promoting the NRF2 signaling pathway.
3.2. Curcumin
Curcumin (
12) is a phytochemical usually obtained from rhizomes of Curcuma longa that exhibits significant antioxidant and anti-inflammatory activities
[98][99]. It contains an α,β-unsaturated carbonyl group that accounts for its neuroprotective effect via NRF2 activation. It has been found to promote the nuclear expression levels and biological effects of NRF2 through the interaction of the α,β-unsaturated carbonyl moiety with Cys151 in KEAP1
[100][101]. According to a recent report by Park and co-workers
[102], curcumin (
12) induces the expression of NRF2-dependent genes such as NQO1, GST-mu1 and HO-1 and increases the level of NRF2 protein in neuronal cells. The activation of NRF2 by curcumin (
12) is reportedly accomplished via PKCα- mediated P62 phosphorylation at Ser351
[102]. Similarly, Ashrafizadeh et al.
[103] reported that curcumin activates the NRF2 signaling pathway by inhibiting KEAP1, up-regulating the expression of NRF2 and its dependent genes and promoting nuclear translocation of NRF2. The pre-treatment with curcumin (
12) prevents hemin-induced neuronal death by inducing NRF2 and antioxidant response in cultures of cerebellar neurons of rats
[104]. Curcumin (
12) also inhibits the upregulation of inflammatory signaling-mediated KEAP1 synthesis and reduces NRF2 degradation in HepG2 cells
[105]. Furthermore, curcumin (
12) hinders oxidative stress in human nasal fibroblasts that have been exposed to urban particulate matter via the activation of the NRF2/HO-1 signaling pathway
[106]. Of note, although curcumin (
12) has been found to alleviate oxidative stress, the co-administration of curcumin and vitamin E gives a better result
[107]. Co-treatment with vitamin E and curcumin of hypo- and hyper- thyroid rats resulted more efficient in down-regulating oxidative stress evaluated as lipid peroxidation and glutathione levels, and in promoting activities and protein expression of antioxidant enzymes such as superoxide dismutase, catalase, glutathione peroxidase and glutathione reductase, when compared to individual treatment. In the same study, a modeled active portion of the protein NRF2 indicated its interaction with both vitamin E and curcumin. Furthermore, in silico experiments showed the interaction of curcumin and vitamin E complex with KEAP1, suggesting that the more effective attenuation of oxidative stress by the concomitant administration of these two antioxidants might be the result of NRF2/KEAP1 pathway modulation
[107].
3.3. J-Series Cyclopentenone Prostaglandin
15-Deoxy-D-prostaglandin J
2 (15d- PGJ2) (
13) is a peroxisome proliferator-activated receptor γ ligand. It represents the J-series cyclopentenone prostaglandin and exerts cytoprotection via NRF2-mediated induction of antioxidant enzymes due to the presence of α,β-unsaturated carbonyl moiety
[108][109]. Song et al.
[110] corroborated the importance of the α,β-unsaturated carbonyl group in NRF2 activation by demonstrating that 9,10-dihydro-15d-PGJ2 (H
2-15d-PGJ
2), an analogue of 15d-PGJ2 that lacks α,β-unsaturated carbonyl moiety as a Michael acceptor, is not able to induce the NRF2 signaling pathway. 15d-PGJ2 (
13) induces the up-regulation of multidrug resistance associated proteins through the activation of the NRF2-ARE signaling pathway
[111]. It has been found to regulate the expression of NRF2-dependent genes and enzymes
[112]. However, NADPH-dependent alkenal/one oxidoreductase reportedly attenuated the ability of 15d-PGJ2 (
13) to affect NRF2-mediated induction of cytoprotective enzymes
[111].
3.4. Chalcone and Its Derivatives
Chalcone and its derivatives exhibit significant antioxidant, anti-inflammatory and anticancer activities
[113][114][115][116]. Their ability to activate the NRF2 signaling pathway has been attributed to the presence of an α,β-unsaturated carbonyl moiety
[7]. Miranda-Sapla and co-workers
[117] reported that
trans-chalcone (
14) modulates inflammatory response and enhances the total bound iron capacity via the activation of NRF2 and expression of HO-1 and ferritin. It also down-regulates ROS and NO levels in leishmania amazonensis-infected macrophages. Licochalcone A (
15) induces nuclear translocation and activation of NRF2 through which it elevates the expression of the anti-inflammatory enzymes and determines licorice extract-induced lowered cutaneous oxidative stress in vivo
[118]. Isoliquiritigen (ISL) (
16), a natural chalcone compound, attenuates oxidative stress and inflammatory injuries via the activation of NRF2 signaling, as demonstrated in a mouse model of severe acute pancreatitis in which ISL determined a reduction in malondialdehyde, interleukin-6, tumor necrosis factor-α and cleaved-caspase-3 and an increase in NRF2, HO-1, NQO1 and superoxide dismutase (SOD)
[119]. Chalcone flavokawain A (
17) is a chalcone derivative that suppresses lipopolysaccharide-induced inflammation through activating the NRF2/ARE-mediated genes and inhibiting the ROS/NF-ĸB signaling in primary splenocytes
[120].
3.5. Dimethyl Fumarate
Dimethyl fumarate (DMF) (
18) is an α,β-unsaturated carboxylic acid ester, approved for the treatment of relapsing multiple sclerosis
[8][121][122]. It exhibits significant antioxidant, anti-inflammatory and NRF2 activities due to the presence of α,β-unsaturated carbonyl moiety
[59][123][124]. Akin et al.
[124] reported that oral administration of DMF (
18) alleviates oxidative stress via activation of NRF2/KEAP1 pathway in mouse ovary. Gopal et al.
[125] reported evidence of NRF2 pathway activation in multiple sclerosis patients that were treated with DMF in Phase 3 studies. Ahuja et al.
[126] observed that DMF (
18) activates the NRF2 pathway, depletes glutathione level, decreases the viability of cells and inhibits mitochondrial oxygen consumption in a dose-dependent manner. Based on these observations, they recommended the development of monomethyl fumarate (MMF) a bioactive metabolite of DMF, which does not exhibit similar adverse effects, as a novel Parkinson’s disease drug
[126]. In summary, the reactivity of α,β-unsaturated carbonyl system with thiols of the KEAPl cysteine residues is responsible for the activation of the NRF2 signaling pathway and accounts for the antioxidant and anti-inflammatory activities of α,β-unsaturated carbonyl-containing compounds. DMF is a notable multi-target compound that modulates NRF2, nuclear factor kappa-light-chain-enhancer of activated B cells (NF-ĸB), hydrocarboxylic acid receptor (HCAR2) pathways and regulates glutathione and iron metabolism which is utilized for the treatment of neurodegenerative diseases
[127].
4. α,β-Unsaturated Sulfonyls
The sulfonyl group is an electron-withdrawing moiety found in several organosulfur compounds such as sulfones, sulfonamides and sulfonates
[15][128]. The strong electron-withdrawing effect of the sulfonyl group accounts for the tendency of α,β-unsaturated sulfonyls to add to nucleophiles in order to form Michael-type adducts. This property also makes α,β-unsaturated sulfonyls to act as powerful dienophiles
[129]. Several sulfonyl-containing compounds exhibit significant antioxidant and anti-inflammatory activities
[130][131][132][133]. α,β-unsaturated sulfonyls are notable building blocks in the synthesis of organic compounds
[134]. They exhibit notable biomedical significance
[135]. They inhibit several enzymatic processes making them essential moieties in drug design and medicinal chemistry
[37]. The first α,β-unsaturated sulfonyls were reported as potent inhibitors of cysteine proteases in 1995
[136]. They are inhibitors of cruzain, HIV-1 integrase,
Staphylococcus aureus sortase, among others
[137][138][139]. α,β-unsaturated sulfonyls reversibly inhibit diverse enzymes via conjugate addition reaction with the thiols of cysteine residue
[136][139][140]. They are effective for intracellular inhibition of dipeptidyl peptidase1
[141][142]. α,β-unsaturated sulfonyls are reportedly activators of the NRF2 signaling pathway
[2][9][16][143].
4.1. Vinyl Sulfones
Vinyl sulfones have been reported as modulators of NRF2 activity due to the presence of the α,β-unsaturated sulfonyl system that accounts for their effectiveness as Michael acceptors
[2][9][18]. Carlstrom et al.
[2] reported that vinyl sulfone (
19) activates the NRF2 signaling pathway with limited off-target effects on hypoxia-inducible factor 1 and NF-ĸB in PTRAF-transfected HEK293 cells. Lee and co-workers
[16] also reported that compound
19 activates NRF2 signaling and induces the up-regulation of the expression of NRF2-dependent antioxidant enzymes in microglia. It inhibits the expression of proinflammatory enzymes and proinflammatory cytokines production in activated microglia. Woo et al.
[143] reported that compound
19 in dopaminergic (DAergic) neuronal cells activates NRF2 and up-regulates the expression of NRF2-regulated antioxidant enzymes at mRNA and protein levels. It exerts neuroprotection and attenuates Parkinson’s disease (PD)-related deficits in PD mouse models
[144]. Choi and co-workers
[9][18] corroborated that compound
19 activates NRF2 and induces the expression of NRF2-regulated antioxidant mediators in PD mice. Although extensive researches have proven that compound
19 exhibits the highest NRF2 activity amongst its vinyl sulfone analogues, however, its poor drug-like properties remain a concern. In view of this, Choi et al.
[18] designed a vinyl sulfone derivative (
20) with improved NRF2 activation potency and drug-likeness. Compound
20 significantly induces NRF2 activation, up-regulation of NRF2-dependent genes, improves the movement ability in acute 1-methyl-4-phenyl-1,2,3,6-tetrahydropyridine-induced PD mice and reduces microglial activation and loss of DAergic neurons
[18]. Vinyl sulfone derivative (
21) is reportedly more potent than chalcone and vinyl sulfoxide analogues in activating the NRF2 signaling pathway and up-regulating the expression of HO-1 gene
[143]. Vinyl sulfone compounds
22 and
23 induce the relief of H
2O
2-induced lesions, neutralize ROS, activate antioxidant response and promote neuroprotection via the activation of NRF2 pathway in PC12 cells. However, the neuroprotective activity of compound
22 is higher than that of compound
23 [145]. The electrophilicity and steric hindrance of α,β-unsaturated sulfones have been tuned to generate several potent NRF2 activators
[145].
4.2. Vinyl Sulfonamides
Sulfonamides exhibit antioxidant and anti-inflammatory activities
[146][147][148][149][150][151][152]. The presence of the α,β-unsaturated sulfonyl system in vinyl sulfonamides enable them to act as Michael acceptors and activate the NRF2 signaling pathway
[18]. Choi and co-workers
[18] synthesized several vinyl sulfonamides by substituting the sulfone moiety of compound
19 with sulfonamide moiety to improve NRF2 activation ability. The analysis of antioxidant enzymes and inflammatory cytokines expression in BV-2 microglial cells and SH-SY5Y human neuroblastoma cells, and of in vivo therapeutic effects on Parkinsonism in a mouse model of Parkinson’s disease showed that compounds
24,
25,
26,
27,
28 exhibit NRF2 activity and compound
26 is the most potent NRF2 activator. However, compound
26 is not as potent as the vinyl sulfonate analogues
[18].
4.3. Vinyl Sulfonates
Sulfonates exhibit antioxidant and anti-inflammatory activities
[153][154]. Vinyl sulfonate are highly activated Michael acceptors due to the α,β-unsaturated sulfonyl moiety they contain
[18]. Vinyl sulfonate compounds
29,
30 and
31 have been reported as potent activators of the NRF2 signaling pathway
[18]. They exert therapeutic effects against Parkinson’s disease via their antioxidant, anti-inflammatory and neuroprotective activities
[18]. Compound
29 exhibits about seven times NRF2 activity higher than its vinyl sulfone analogue (
19). Compound
29 increases NRF2-related protein levels attenuates inflammation and decreases the production of NO in BV-2 cells. It also up-regulates the expression of NRF2-regulated antioxidant enzymes and inhibits motor deficits in Parkinson’s disease
[18].
5. α,β-Unsaturated Sulfinyls
The sulfinyl group is available in several organosulfur compounds. It is a strong electron-withdrawing moiety and exhibits high configurational stability and several biological functions such as antioxidant, anti-inflammatory and NRF2 up-regulation activities
[155][156][157][158]. Recently, sulfinyl group has been utilized in controlling the enantioselectivity of 1,4-additions involving carbon nucleophiles to α,β-unsaturated sulfoxides
[159]. Similarly, α,β-unsaturated sulfinyl group is a very essential partner in Michael addition reaction involving thiols of the KEAP1 cysteine residues in NRF2 activation
[160][161]. α,β-unsaturated sulfinyl compounds activate the NRF2 signaling pathway.
Vinyl Sulfoxide
Sulfoxides exhibit antioxidant and anti-inflammatory activities
[160][162]. The ability of vinyl sulfoxide to activate NRF2 and to induce HO-1 has been linked to the presence of an α,β-unsaturated sulfinyl system
[143]. Woo and co-workers
[143] synthesized vinyl sulfoxide (
32) based on chalcone structure. In an attempt to determine the NRF2-activating potency of compound
32. Woo et al.
[143] assessed its ability to induce the expression of a NRF2-dependent genes in BV2 cells. Compound
32 was found to exhibit significant HO-1 inducing activity and confirmed to be a potent as its vinyl sulfone and chalcone analogues
[143]. Shim et al.
[3] designed and synthesized vinyl sulfoxide derivatives (
33 and
34) using sulforaphane and gallic acid as structural templates and tested their HO-1 inducing ability as the measure of NRF2 activation in BV2 microglial cells. However, compounds
33 and
34 exhibit moderate HO-1 inducing activity and no inhibitory effect on NO production
[3], thus suggesting that a more efficient electrophile is needed to get more effective NRF2 activator. α,β-unsaturated sulfinyl compounds activate the NRF2 signaling pathway as shown in
Table 1.
Table 1. α,β-Unsaturated moiety-bearing compounds as NRF2 activators/KEAP1 inhibitors.
S/N |
Compound |
Disease Studied |
Model |
NRF2 Activating Conc/Activity |
Mechanism of Action |
Biological Activity |
Reference |
8 |
Sesquiterpene lactones 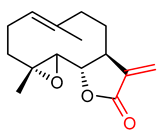
Parthenolide |
Obesity |
Mice, Adipocytes (3T3-L1), RAW264.7 |
1–8 µM |
electrophilic modification of KEAP1 cysteine residues |
NRF2 activation, Antioxidant, Anti-adipogenesis |
[124] |
Obesity |
3T3-L1 Cell |
1–8 µM |
NRF2 activation, Antioxidant, Anti-inflammatory |
[125] |
Osteoporosis |
Human |
5–20 µM |
NRF2 activation, Antioxidant, Anti-apoptosis |
[126] |
Breast cancer |
Human breast cancer cell line MDA-MB 231 |
2.0 µM |
NRF2 regulation, chemoresistance |
[133] |
Chronic lymphocytic leukemia |
Human peripheral blood mononuclear cells (PBMCs) |
1.46 µM |
NRF2 activation, Antioxidant, cytotoxicity |
[111] |
9 |
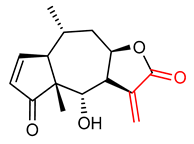 Helenalin |
Acute hepatic injury |
Male C57BL/6 Mice |
0.75–3.00 mg/kg |
electrophilic modification of KEAP1 cysteine residues |
NRF2 activation, Antioxidant, Anti-inflammatory |
[137] |
10 |
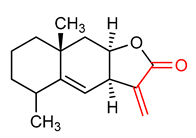 Alantolactone |
Breast cancer |
MCF-7 human breast cancer cells |
10–30 µM |
electrophilic modification of KEAP1 cysteine residues |
NRF2 activation, anticancer |
[142] |
Cancer |
Heps1c1c7 cells |
1–10 µM |
NRF2 activation, Antioxidant, anticancer |
[143] |
Chronic obstructive pulmonary disease (COPD) |
Cigarette smoke-induced human bronchial epithelial cells |
1–10 µM |
NRF2 activation, Antioxidant, Anti-inflammatory |
[144] |
11 |
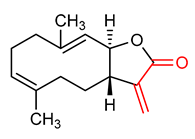 Costunolide |
Acute liver injury |
Mice |
20–40 mg/kg |
electrophilic modification of KEAP1 cysteine residues |
NRF2 activation, Antioxidant, Anti-inflammatory |
[146] |
Oxidative damage |
PC12 Cells |
5 µM |
NRF2 activation, Antioxidant, neuroprotection |
[150] |
Tumor |
RAW264.7 Macrophages |
0.1–1.0 µM |
NRF2 activation, Anti-inflammatory |
[147] |
12 |
Curcumin 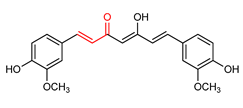 |
Neurodegenerative diseases |
Neuronal cells |
10 µM |
electrophilic modification of KEAP1 cysteine residues |
NRF2 activation, Antioxidant |
[155] |
Oxidative stress, inflammation |
HepG2 Cells |
50 mg/kg |
NRF2 activation, Antioxidant, Anti-inflammatory |
[158] |
Nasal diseases |
Human nasal fibroblast |
0–5 µM |
NRF2 activation, Antioxidant |
[159] |
Oxidative stress |
Rats |
30 mg/kg |
NRF2 activation, Antioxidant |
[160] |
13 |
Prostaglandin 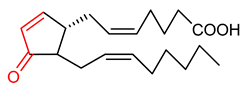 15-Deoxy-∆12,14-prostaglandin J2 |
Breast cancer |
Human breast cancer cells |
10 µmol/L |
electrophilic modification of KEAP1 cysteine residues |
NRF2 activation, Antioxidant |
[163] |
Cancer |
Mouse embryonic fibroblast (MEF) 293 cells |
0.5–10 µM |
NRF2 activation, Antioxidant, Anticancer |
[164] |
14 |
Chalcones 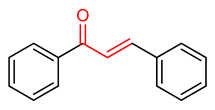 Trans-chalcone |
Leishmannia amazonensis |
L. amazonensis-infected macrophages |
2–12 µM |
electrophilic modification of KEAP1 cysteine residues |
NRF2 activation, Antioxidant, |
[169] |
15 |
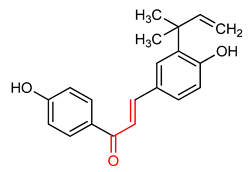 Licochalcone A |
Cutaneous oxidative stress |
UVA-irradiated human dermal fibroblast |
9 µM |
electrophilic modification of KEAP1 cysteine residues |
NRF2 activation, Antioxidant, Anti-inflammatory |
[170] |
16 |
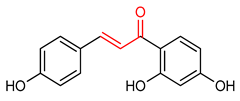 Isoliquiritigenin |
Pancreatic injury |
Mice |
>3% |
electrophilic modification of KEAP1 cysteine residues |
NRF2 activation, Antioxidant, Anti-inflammatory |
[171] |
17 |
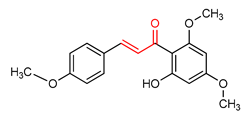 Chalcone flavokawain A |
inflammation |
Primary splenocytes |
2–30 µM |
electrophilic modification of KEAP1 cysteine residues |
NRF2 activation, Antioxidant, Anti-inflammatory |
[172] |
18 |
DMF 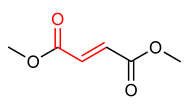 Dimethyl fumarate |
Oxidative stress |
Mouse ovary |
20 mg/kg |
electrophilic modification of KEAP1 cysteine residues |
NRF2 activation, Antioxidant, |
[175] |
Multiple sclerosis |
Multiple sclerosis patient |
0–400 |
NRF2 activation, Antioxidant, |
[173] |
Parkinson’s disease |
Mice |
0.05–80 µM |
NRF2 activation, Antioxidant, Anti-inflammatory |
[177] |
19 |
Vinyl Sulfones 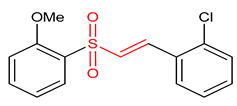 (E)-1-chloro-2-(2-((2-methoxyphenyl)sulfonyl)vinyl)benzene |
Multiple sclerosis |
HEK293 |
10 µM |
electrophilic modification of KEAP1 cysteine residues |
NRF2 activation, Antioxidant, |
[2] |
Parkinson’s disease |
PD animal model |
1–20 µM |
NRF2 activation, Antioxidant, Anti-inflammatory |
[23] |
Parkinson’s disease |
PD animal model |
1–10 µM |
NRF2 activation, Antioxidant, Neuroprotection |
[32] |
20 |
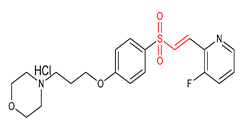 (E)-4-(3-(4-((2-(3-fluoropyridin-2-yl)vinyl)sulfonyl)phenoxy)propyl)morpholine hydrochloride |
Parkinson’s disease |
PD mice |
0.3–10 µM |
electrophilic modification of KEAP1 cysteine residues |
NRF2 activation, Antioxidant, Neuroprotection |
[9] |
21 |
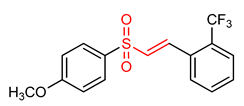 (E)-1-(2-((4-methoxyphenyl)sulfonyl)vinyl)2-(trifluoromethyl)benzene |
Parkinson’s disease |
PD mice |
20 µM |
electrophilic modification of KEAP1 cysteine residues |
NRF2 activation, Antioxidant, Neuroprotection |
[32] |
22 |
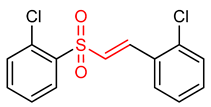 (E)-1-chloro-2-(2-((2-chlorophenyl)sulfonyl)vinyl)benzene |
Oxidative stress |
PC12 Cells |
2.5–1.0 µM |
electrophilic modification of KEAP1 cysteine residues |
NRF2 activation, Antioxidant, Neuroprotection |
[194] |
23 |
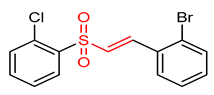 (E)-1-bromo-2-(2-((2-chlorophenyl)sulfonyl)vinyl)benzene |
Oxidative stress |
PC12 Cells |
0.5–1.0 µM |
electrophilic modification of KEAP1 cysteine residues |
NRF2 activation, Antioxidant, Neuroprotection |
[194] |
24 |
Vinyl Sulfonamides 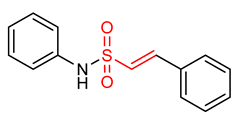 (E)-N,2-diphenylethenesulfonamide |
Parkinson’s disease |
PD mouse |
>10 µM |
electrophilic modification of KEAP1 cysteine residues |
NRF2 activation, Antioxidant, Anti-inflammatory |
[17] |
25 |
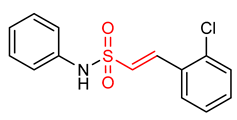 (E)-2-(2-chlorophenyl)-N-phenylethesulfonamide |
Parkinson’s disease |
PD mouse |
>10 µM |
electrophilic modification of KEAP1 cysteine residues |
NRF2 activation, Antioxidant, Anti-inflammatory |
[17] |
26 |
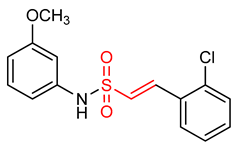 (E)-2-(2-chlorophenyl)-N-(2-methoxyphenyl)ethenesulfonamide |
Parkinson’s disease |
PD mouse |
6.35 µM |
electrophilic modification of KEAP1 cysteine residues |
NRF2 activation, Antioxidant, Anti-inflammatory |
[17] |
27 |
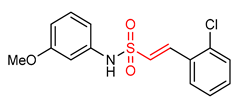 (E)-2-(2-chlorophenyl)-N-(3-methoxyphenyl)ethane sulfonamide |
Parkinson’s disease |
PD mouse |
>10 µM |
electrophilic modification of KEAP1 cysteine residues |
NRF2 activation, Antioxidant, Anti-inflammatory |
[17] |
28 |
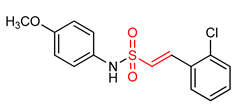 (E)-2-(2-chlorophenyl)-N-(4-methoxyphenyl)ethane sulfonamide |
Parkinson’s disease |
PD mouse |
>10 µM |
electrophilic modification of KEAP1 cysteine residues |
NRF2 activation, Antioxidant, Anti-inflammatory |
[17] |
29 |
Vinyl Sulfonates 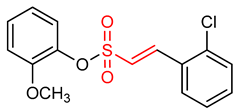 (E)-2-methoxyphenyl 2-(2-chlorophenyl)ethenesulfonate |
Parkinson’s disease |
PD mouse |
0.076 µM |
electrophilic modification of KEAP1 cysteine residues |
NRF2 activation, Antioxidant, Anti-inflammatory |
[17] |
30 |
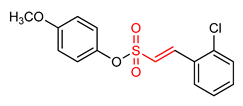 (E)-4-methoxyphenyl 2-(2-chlorophenyl)ethenesulfonate |
Parkinson’s disease |
PD animal model |
0.237 µM |
electrophilic modification of KEAP1 cysteine residues |
NRF2 activation, Antioxidant, Anti-inflammatory |
[17] |
31 |
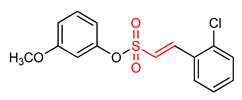 (E)-3-methoxyphenyl 2-(2-chlorophenyl)ethenesulfonate |
Parkinson’s disease |
PD mouse |
0.165 µM |
electrophilic modification of KEAP1 cysteine residues |
NRF2 activation, Antioxidant, Anti-inflammatory |
[17] |
32 |
Vinyl Sulfoxides 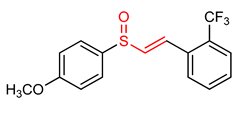 (E)-1-(2-((4-methoxyphenyl)sulfinyl)vinyl)-2-(trifluoromethyl)benzene |
Parkinson’s disease |
BV-2 Cells |
20 µM |
electrophilic modification of KEAP1 cysteine residues |
NRF2 activation, Neuroprotection |
[32] |
33 |
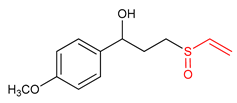 1-(4-methoxyphenyl)-3-(vinylsulfinyl)propan-1-ol |
Parkinson’s disease |
BV-2 Cells |
20 µM |
electrophilic modification of KEAP1 cysteine residues |
NRF2 activation, Antioxidant, |
[3] |
34 |
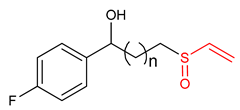 1-(4-fluorophenyl)-3-(vinylsulfinyl)propan-1-ol |
Parkinson’s disease |
BV-2 Cells |
20 µM |
electrophilic modification of KEAP1 cysteine residues |
NRF2 activation, Antioxidant, |
[3] |