1. Inner Ring: The Flexible Core of the Nuclear Pore Complex
The architecture of the inner ring, with its symmetry along the nucleocytoplasmic axis, is highly conserved
[1]. It coats the NE with eight spokes positioned around the central transport channel, each formed by three layers. Closest to the central channel, the innermost layer consists of the so-called channel nucleoporin heterotrimer (Nup49, Nup57, Nsp1), which projects intrinsically disordered FG-rich segments into the central NPC channel
[2]. The outer, membrane-binding layer is composed of the α-solenoid/β-propeller domain paralogues Nup157/Nup170, which bind the NE via an amphipathic lipid packing sensor (ALPS) motif positioned in a loop between two β-propeller blades
[3][4]. The paralogues Nup188/Nup192 have an NTR-like structure
[5][6][7] and form the central layer between the membrane binding NUPs and the channel nucleoporin heterotrimer. The rigid layers are linked by flexible connectors: the flexible N-terminus of Nic96 ties the membrane binding layer to Nup188/Nup192 and the channel nucleoporin heterotrimer, and the short linear motifs (SliMs) in the membrane-interacting Nup53/Nup59 connect most of the inner ring NUPs
[8][9][10][11][12][13]. While the NUPs within a single inner ring spoke have large interaction surfaces
[9], recent structural models of the NPC propose that the interactions between spokes are minimal and instead are mostly mediated by natively disordered and flexible connector NUPs
[8][9][10][11]. This flexibility allows the NPC to adjust its diameter depending on the physiological state of the cell
[8][14][15][16][17][18], and the resulting spaces between spokes might solve the long-standing question of how transmembrane proteins can pass through the NPC. Intriguingly, Nup188/Nup192 (hsNup188/hsNup205; hs for
Homo sapiens) not only share structural similarity with NTRs, their interaction with Nic96 (hsNup93) also resembles the interaction of the transport receptor importin-β and the importin-β binding domain (IBB) of its cargo complex
[8][10][14][19]. This points to a common evolutionary origin of NUPs and transport receptors
[5][6][7][8][20].
2. Symmetric Outer Rings: The Versatile Outer Coat of the Nuclear Pore Complex
The outer rings on the nuclear and cytoplasmic faces of the NPC are largely identical and made up of rigid subcomplexes known as Y complexes
[21][22]. These building blocks are themselves composed of six conserved constituent proteins (Seh1 is not a conserved element of the Y complex in thermophilic fungi and
Aspergillus nidulans [23][24][25]), which form a structure resembling the shape of the letter Y
[26][27][28][29], and eight Y complexes assembled in a head-to-tail manner.
S. cerevisiae has a single cytoplasmic and nucleoplasmic Y complex ring
[8][30][31], human and
Xenopus laevis NPCs carry two Y complex rings per side
[4][19][21][32][33][34], and the green algae
Chlamydomonas reinhardtii and fission yeast
Schizosaccharomyces pombe exhibit an asymmetric distribution, with two nuclear and only one cytoplasmic Y complex rings
[16][35]. Notably, the cytoplasmic Y complex ring of
S. pombe only consists of the Y triskelion, breaking the head-to-tail arrangement observed in other species
[16]. Surprisingly, the number of Y complex rings can vary even within the same cell: a subset of NPCs with two nucleoplasmic Y complex rings was recently observed in budding yeast (
[8], further discussed below).
The largely α-solenoid core of the Y complex is tethered to the NE by ALPS motifs in the β-propeller of Nup120 and Nup133
[36][37][38] and decorated by several species-specific β-propeller NUPs
[39][40][41]. The α-solenoid/β-propeller architecture of the outer and inner ring NUPs is similar to the vesicle-coating protein complexes COPI and COPII, and the β-propeller protein Sec13 is a shared component of both NPCs and COPII complexes, suggesting a common evolutionary origin (reviewed in
[42]). Although the eightfold rotational symmetry of the NPC is well established, deviations have been observed in
X. laevis NPCs
[43][44], which raises the question how the eightfold symmetry of the NPC is formed. Since the connections between inner ring spokes are flexible and the inner ring diameter can change drastically
[14][15][16][17], it seems likely that the oligomerization of the Y complex ring plays a key role in establishing the correct stoichiometry of NPC subunits. However, the Y complex itself is not a rigid structure and has multiple hinge points
[26], and additional constraints, such as, e.g., membrane interaction may thus be needed to determine the eightfold symmetry of the NPC.
The outer rings are connected to the inner ring by a set of paralogues with flexible linkers (Nup116, Nup100, Nup145N)
[10][12][31][45], and the double rings in metazoan and
C. reinhardtii NPCs are linked by an additional copy of hsNup155 or crNup155 (cr:
C. reinhardtii), respectively
[4][21][32][35]. In metazoan NPCs, the chromatin-binding NUP ELYS is associated with one short arm of the Y complex on the nucleoplasmic side
[14][19][46][47][48].
Interestingly, recent biochemical characterization and higher-resolution electron microscopy (EM) maps of the NPC revealed that the importin-β-IBB-like complex hsNup205-hsNup93 is not only a part of the inner ring, but it can also be found in the outer rings of metazoan NPCs
[11][14][19][34]. A characteristic question mark-shaped density can also be seen in EM maps from the double Y complex rings of
S. cerevisiae and
C. reinhardtii [8][35], and the presence of the hsNup205-hsNup93 heterodimer and its homologues may thus be conserved and important for the oligomerization of double Y rings.
3. Asymmetric Appendages: Functional Extensions of the Nuclear Pore Complex
The symmetry of the outer rings is broken by several subcomplexes that specifically bind to the cytoplasmic or nucleoplasmic ring. Identified by classical EM experiments, the cytoplasmic filaments and nuclear basket are the most prominent asymmetric components of the NPC
[49][50][51]. The term cytoplasmic filaments is often used as a synonym for all NUPs that preferentially localize to the cytoplasmic side of the NPC. However, the main component of these elongated filaments protruding into the cytoplasm in metazoa is the largely disordered C-terminus of hsNup358, which harbors multiple Zinc-fingers and Ran-binding domains, and plays an important role in receptor-mediated transport and protein translation
[11]. hsNup358 is specific to metazoa and stabilizes the cytoplasmic double Y ring
[11][32].
The majority of the other cytoplasmic NUPs are conserved across species and form the so-called mRNA export platform. This extends to the center of the NPC
[8][30][52][53][54] and plays a key role in mRNA export and remodeling
[55]. Intriguingly, the mRNA export platform has high similarity with the channel nucleoporin heterotrimer at the center of the NPC, with Nsp1 being a shared component between the two. Further, the positioning of the hsNup93-hsNup205 heterodimer in the cytoplasmic outer ring and its biochemical interactions suggest that hsNup93 connects the cytoplasmic mRNA export platform in a similar way as the channel nucleoporin heterotrimer in the inner ring
[11]. Interestingly, the mRNA export platforms in metazoa and yeast have different overall architectures. In yeast, the cytoplasmic coiled-coil NUPs form a single complex, whereas two parallel-orientated complexes are present in the
X. laevis NPC
[19]. This corresponds to the number of cytoplasmic Y rings in the two species. Intriguingly, the mRNA export platform is entirely absent in the more divergent eukaryote
Trypanosoma brucei [1][56]. In contrast to the conserved Y complex and inner ring, the mRNA export platform might thus have specialized to meet the needs of the respective organism during evolution.
The nuclear basket was identified in early EM studies because of its characteristic elongated structure
[49][50], but due to its flexible nature, it remains one of the least structurally characterized modules. The majority of the basket-like structure seen by classical EM analysis
[57][58] likely stems from the large coiled-coil hsTPR (
S. cerevisiae Mlp1/Mlp2)
[59][60]. Although the stoichiometry of the nuclear basket coiled-coil NUPs is not entirely clear
[31][61][62][63][64], up to eight basket-like filaments protruding into the nucleoplasm and tethering proteasomes to the NPC have been observed at single NPCs of
C. reinhardtii [65].
So far, the best-resolved fragments of the nuclear basket are coiled-coil segments that likely belong to Mlp1/2 and bind to the nuclear Y complex
[8], which is consistent with other EM and crosslinking data
[30][31]. The inventory of the
S. cerevisiae nuclear basket is completed by the mostly disordered Nup1, Nup2, and Nup60. Although these NUPs have evaded structural characterization, biochemical studies show that Nup1 and Nup60 (hsNup153) interact with the NE via an amphipathic helix (AH)
[66][67][68]. Similar to the linker NUPs in other subcomplexes, Nup60 flexibly connects the nucleoplasmic Y complex ring with the Mlps and Nup2 (hsNup50) via SLiMs
[66][69][70]. Further, Nup1, Nup2, and Nup60 contain FG repeats and, together with the Mlps, are important for export and quality control of mRNA (reviewed in
[71]).
4. The Membrane Ring: An Enigmatic Girdle
Besides the membrane interactions of the inner and outer rings mediated by ALPS motifs, the NPC is also directly anchored in the NE by transmembrane NUPs. Because of their transmembrane regions, it is difficult to purify these proteins or distinguish them from the NE in EM studies, and the structure of the membrane ring is thus poorly characterized. In
S. cerevisiae, there are four transmembrane NUPs, which are not as highly conserved as other components of the NPC
[72] (reviewed in
[73]). Only Ndc1 has a well-defined ortholog in metazoa
[74][75], and is the only essential protein of this group. Ndc1 interacts with the inner ring NUPs Nup53/59 and Nup170 (in humans: hsNdc1, hsNup35, hsNup155, and additionally ALADIN) to form a membrane interaction hub that anchors the inner ring to the NE
[8][13][14]. Pom152 and the human Gp210 are the only NUPs with structured domains in the NE lumen: both contain a series of luminal immunoglobulin repeats
[76][77][78]. Despite the low primary sequence conservation and different membrane topology, the high structural similarity could hint at a common origin for both proteins. The immunoglobulin folds of Pom152 form a belt-like chain of beads around the NPC in the NE-lumen, which is anchored near the membrane interaction hub
[8][16][31][77][78][79]. The belt-like luminal ring deforms together with changes in NPC diameter
[8][16], which raises the possibility that it regulates the diameter of the NPC. However, neither Pom152 nor Gp210 is essential
[80][81], and deletion of Gp210 does not lead to variation of the NPC diameter in cellulo
[14]. Furthermore, the expression level of Gp210 in different cell lines varies widely
[63][82], suggesting a more intricate role of the luminal ring than as just a mechanical girdle.
5. Linker Nucleoporins: An Invisible Thread Stitching the Nuclear Pore Complex Together
The NPC embodies two seemingly contradictory properties. On the one hand, it uses rigid building blocks with large interaction surfaces to form stable subcomplexes, such as the Y complex and the inner ring spokes, which confer a high degree of stability to the NPC core in post-mitotic cells
[83][84][85][86][87] (reviewed in
[88]). On the other hand, its structural flexibility allows for drastic changes in diameter
[8][15][16][17] and likely enables a fast assembly and disassembly of the NPC in open mitosis
[89]. How can these properties coexist in one structure? The emerging solution is a peculiar mode of association between the different NPC modules via intrinsically disordered NUPs. Homologues of the
S. cerevisiae FG repeat NUPs Nup100, Nup145N and Nup116, and non-FG NUPs Nup53 and Nup59 are universally capable of linking several NPC elements each via SLiMs spread throughout their intrinsically disordered domains
[2][12][90][91]. In this way, each of them can flexibly join several core subunits, akin to beads on a string. The electron densities observed next to the core NUPs in high-resolution NPC maps and chemical crosslinking data all point to SLiM-mediated connectivity of the NPC subunits
[8][10][11][14][31]. Further, flexible connections could arise from the ability of some core NUPs to directly bind FG repeats
[45]. These multivalent interactions might create a velcro-like effect that brings about both stability and structural plasticity (
Figure 1).
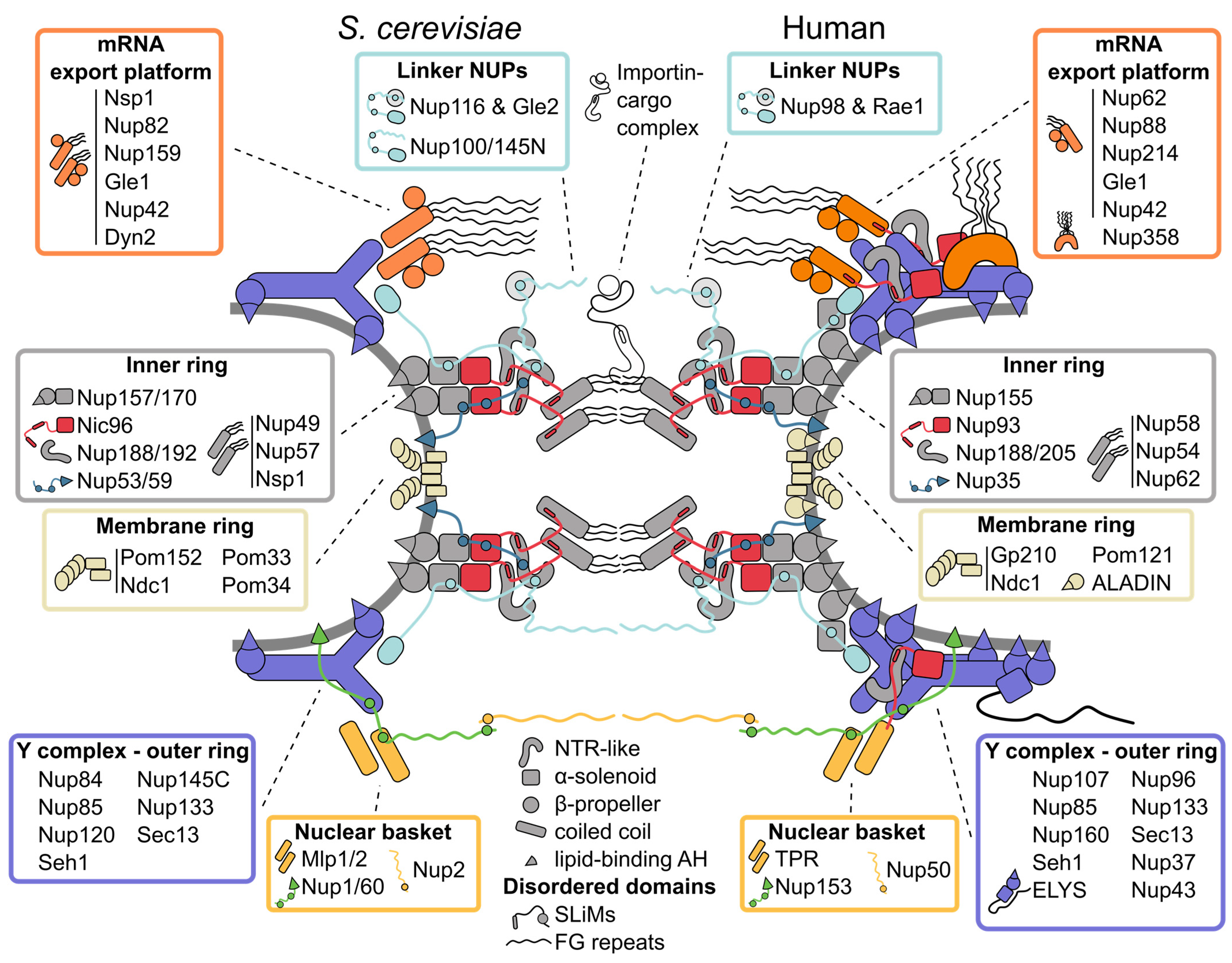
Figure 1. Inventory of the budding yeast and human nuclear pore complex. The nuclear pore complex (NPC) forms a channel connecting the nucleoplasm (bottom) with the cytoplasm (top) and is built of three concentric rings: the cytoplasmic outer ring, the inner ring, and the nucleoplasmic outer ring. The basic building blocks of the NPC are nucleoporins (NUPs), which are organized into several subcomplexes (boxes) and largely composed of only a few structural motifs (center bottom). The rigid subcomplexes are connected by disordered linkers. They contain short linear interaction motifs (SLiMs), which flexibly tie the NUPs and subcomplexes together. Multiple NUPs contain a lipid-binding amphipathic helix (AH) that helps tether the NPC to the lipid membrane. See text for details.
The interactions via short motifs are a prevailing theme also outside the NPC core. Short motif interactions contribute to the attachment of the mRNA export platform, central channel NUPs, the nuclear basket and transmembrane NUPs
[11][12][13][66][67] (
Figure 1). Interestingly, the interactions of the NPC with the NE rely on the same principle. Although transmembrane NUPs are one of the least evolutionarily conserved groups
[72] (reviewed in
[73]), short lipid-binding AHs found within multiple core and non-core NUPs are a conserved feature, often seen positioned along the lipid membrane in current NPC models
[8][14]. This multitude of binding sites could stabilize the high curvature induced in the lipid membrane and establish a tight association of the NPC spokes to the pore membrane (reviewed in
[92]).
Taken together, the short interaction motifs and intrinsically disordered domains emerge as key elements of NPC connectivity.