Polyethylene glycol-modified titanium dioxide (PEG-modified TiO2) nanopowders were prepared using a fast solvothermal method under microwave irradiation, and without any further calcination processes. These nanopowders were further impregnated on porous polymeric platforms by drop-casting. The effect of adding iron with different molar ratios (1, 2, and 5%) of iron precursor was investigated. The approach developed in this work originated novel functionalized photocatalytic platforms, which were revealed to be promising for the removal of organic dyes from wastewater.
1. Brief introduction
As a by-product of the society’s rapid development, environmental contaminants, such as dyes, drugs, and pesticides, are widely being released into effluents and represent, not only a risk to human health, but also to the environment, due to their complex structure and high recalcitrance. Spillage of dyes, widely used in various industries, such as water-soluble Rhodamine B (RhB) and Methylene blue (MB), endangers animals, plants, and human beings
[1][2][3]. In this regard, a growing interest in the removal of pollution effluents from wastewater through environmentally friendly routes has spurred intensive research over the last few years
[4][5][6].
Among the various techniques for decomposition and detoxification of organic dye effluents, photocatalysis is considered an appealing and inexpensive technology, which has been successfully employed for the treatment of pollutants and remediation of water. One of its advantages is the use of solar energy, which makes the process economically viable for large scale applications, while acquiring additional environmental value
[2][3][7][8][9].
Concerning photocatalysts, TiO
2 is in the spotlight and the subject of intense research, due to its remarkable properties, which include a strong oxidation potential to decompose organic pollutants, physical and chemical stabilities, low cost and non-toxicity
[10][11][12][13][14][15]. TiO
2 is widely used in photocatalysis for the degradation of contaminants, in both aqueous and gaseous mediums
[16].
TiO
2 is a semiconductor that presents a wide band gap (~3.2 eV for anatase, ~3.0 eV for rutile and 3.4 eV for brookite
[17], at room temperature) and is usually excited with high-energy UV photons, above the semiconductor’s band gap
[18][19]. As a result, pure TiO
2 absorbs in the UV region (4–5% of the solar spectrum
[18]), which makes its photocatalytic efficiency low under visible light (visible region comprehends ~45% of the solar spectrum
[20]). To overcome this issue and enhance the photocatalytic activity of TiO
2-based materials, several approaches have been tested to extend their use under sunlight, including doping with metal and non-metal elements, through surface modification and by coupling with other semiconductor materials
[12][16][20][21][22][23].
Doping TiO
2 with transition metal ions has been reported to reduce the rate of electron-hole recombination, due to the formation of a Schottky barrier with TiO
2. Simultaneously, it is responsible for narrowing the TiO
2 band gap energy; thus, extending the absorption to the visible spectral range (red-shift), and leading to an improvement in the photocatalytic activity
[24][25][26]. Doping could also potentially increase TiO
2 crystallinity and enlarge the surface area
[27]. Several metal ions have been successfully incorporated into a TiO
2 lattice, including nickel
[28], copper
[29][30], chromium
[31], iron
[1], vanadium
[32], and zinc
[33]. Amongst the different metal ions, Fe appears an excellent candidate for doping TiO
2, due to its half-filled d-electronic configuration and the similar atomic radius of Fe3+ ion (0.69 Å) and Ti4+ ion (0.75 Å). The titanium positions in the TiO
2 lattice can be easily substituted by the cation. Fe3+ is reported to provide trap centers for photogenerated electrons and holes, since the energy level of Fe2+/Fe3+ is located near Ti3+/Ti4+; thus, enhancing the charge separation.
Different substrates have been used for growing Fe-doped TiO2 nanostructures, including glass substrates, polyamide fabric, and Si substrates, but to the best of researchers' knowledge, this has never been reported for porous water filters. The impregnation of these nanostructures on commercial water filters avoids the limitations and costs associated with the recovery of nanometer-sized particles.
In this research, pure TiO2 and 1, 2, and 5 mol% Fe-TiO2 nanostructures were synthesized by a fast surfactant-assisted microwave method (1 h), without any further calcination of the nanostructures, and impregnated using a simple drop-casting technique on polymeric substrates, used as water filters. Enhanced visible light absorption of TiO2-based materials on flexible porous water filter substrates was, thus, produced. The surface modification of TiO2-based materials with PEG was investigated. The materials produced were systematically characterized by XRD, Raman spectroscopy, SEM coupled with EDS, and XPS. Optical characterization was also carried out for the nanostructures, via UV–VIS absorption and room temperature diffuse reflectance measurements, to determine the optical band gap values. Finally, their efficiency as photocatalysts on water filters was investigated for the degradation of the organic model pollutant, RhB, under solar radiation.
2. Conclusions
In summary, pure TiO2 and Fe-TiO2 nanostructures were successfully synthesized by a fast surfactant-assisted microwave irradiation (1 h), without a calcination step, and impregnated by drop-casting on porous polymeric substrates. The approach used in this study enabled the total covering of the porous substrates and the evaluation of their photocatalytic activity in the degradation of RhB under solar radiation. SEM confirmed the formation of fine particles with a sphere-like appearance and films that uniformly coated the substrates. XRD revealed the presence of pure TiO2 anatase in all nanostructures, which was further confirmed by Raman spectroscopy on the impregnated substrates. The platforms impregnated with 5 mol% of Fe-TiO2 nanostructures exhibited an enhanced RhB photodegradation, when compared to pure TiO2 and the pristine substrates. The highest photocatalytic activity for the Fe-TiO2 material under solar radiation reached 85% after 3.5 h, compared to 74% with pure TiO2. The photodegradation rate of RhB dye with the Fe-TiO2 substrate was 1.5-times faster than pure TiO2. The XPS and UV-VIS results support that the presence of Fe ions led to the introduction of new energy levels, as well as defects, such as oxygen vacancies, that played an important role as traps for the photogenerated carriers, leading to a reduction in the recombination rate, followed by a visible enhancement in the photocatalytic activity. In summary, this study demonstrated that the synergy between the micro-porosity of the substrates and the surface-modified Fe-TiO2 nanostructures enhanced the substrates’ photocatalytic properties. Flexible and eco-friendly photocatalytic functionalized substrates were produced with potential for wastewater removal.
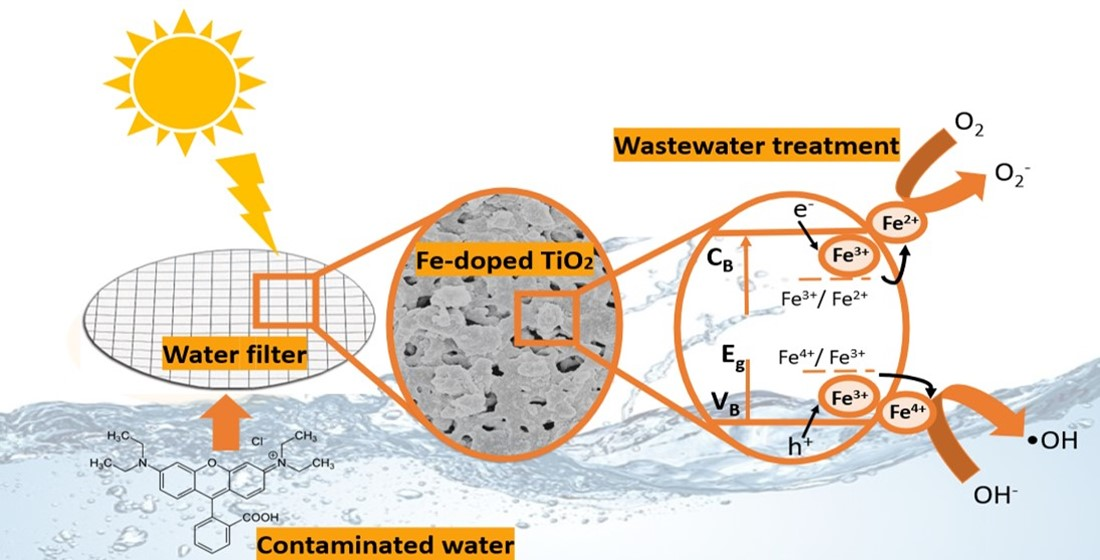
Graphical Abstract