Saporin is a ribosome-inactivating protein that can cause inhibition of protein synthesis and causes cell death when delivered inside a cell. Development of commercial Saporin results in a technology termed ‘molecular surgery’, with Saporin as the scalpel. Its low toxicity (it has no efficient method of cell entry) and sturdy structure make Saporin a safe and simple molecule for many purposes. The most popular applications use experimental molecules that deliver Saporin via an add-on targeting molecule. These add-ons come in several forms: peptides, protein ligands, antibodies, even DNA fragments that mimic cell-binding ligands. Cells that do not express the targeted cell surface marker will not be affected.
1. Introduction
Ribosome-inactivating proteins (RIPs) have long been thought of as potentially perfect payloads for the treatment of human tumors; they could be tools to make Ehrlich’s Magic Bullet to remove harmful cells without harming healthy ones
[1]. A common property shared among RIPs is their highly specific RNA N-glycosidase activity that cleaves the glycosidic bond of a single adenine base (A
4324 in rat) from the ribosomal RNA of the large subunit 28 s
[2]. RIPs are very potent, often active in the picomolar range. They can be targeted, as their corresponding bacterial toxins are, by attachment to molecules that bind to the cell surface and cause internalization. Some of the RIPs have targeting agents already attached and are per se toxins (e.g., ricin, abrin); some do not (e.g., Saporin, gelonin) and are as toxic as a cucumber, that is, not at all. In these latter cases, if the proper targeting agent is used, a specific antibody for instance, they can be very specific and very toxic to many different cell types that bind the targeting moiety, especially cancer cells.
That’s the upside. As RIPs had been utilized, some problems were found, and many of them have been resolved over the years. The presence of attached sugars created issues for pharmacokinetics because of binding to various membrane sugar receptors
[3]. Sometimes the RIP was surprisingly inactive against the target cell type without artificial ‘enhancement’
[4]. Worst of all was the immunogenicity of the plant protein when injected systemically into a human. This also occurs with the bacterial toxins.
One of the things that is reliable with Saporin is that if it is part of a complex that binds to a cell surface target, it will be internalized. This is not always the case with some of the toxin enzymatic chains. With Pseudomonas exotoxin, quite a bit of work went into the addition of sequence pieces outside of the enzymatic chain that were necessary for causing internalization and cell death
[5][6][7][8][9]. This was accomplished primarily by the group of Ira Pastan over many years and the result is an anti-tumor drug, Lumoxiti. In addition to producing a cancer drug, the work of the Pastan group to diminish immunogenicity by the toxic moiety is a model for plant toxin workers.
There are, after all, a number of steps on the way: binding of the targeted toxin to the cell surface target, internalization, intracellular processing and trafficking to reach the cytosol, and inhibition of protein synthesis through ribosome-inactivation or one of the other players in protein synthesis, elongation factor 2
[10]. Saporin per se, and its related brother gelonin, are able to enter the cell along with its targeting agent with no extra additions to their sequences.
1.1. RIPs in Cancer Research
Saporin was first characterized from
Saponaria officinalis by a group at the University of Bologna under the direction of Fiorenzo Stirpe
[11]. It was identified as a good-sized chromatography peak of the soluble extract of the plant and was termed peak six, or SO-6, with high activity and high stability. Stirpe collaborated with Phillip Thorpe’s group in England to publish activity of a Saporin immunotoxin in a lymphoma model
[12]. In a telling comment, the authors stated that, “Ricin A-chain coupled to OX7 antibody was one hundredfold to one thousandfold less effective than OX7-Saporin as an antitumor agent in vivo”. Over the years, a Saporin-targeted toxin has been used many times for the treatment of human disease
[13][14][15], but has not yet been approved as an anti-cancer drug component. Efforts to produce an approved Saporin as a cancer drug continue, as seen recently
[16] and in other articles contained in this issue of Toxins.
It is appropriate to mention that systemic administration of Saporin conjugates would dictate nearly immediate contact with tumors from systemic sources, as opposed to solid tumors. In addition, solid tumors, by definition, have tumor cells that are not immediately accessible; they are hidden in the three-dimensional structure of the tumor. This is one reason that much of the experimental success with Saporin conjugates is in cells in the systemic fluid. As to the development of a Saporin conjugate as a cancer drug, the immunogenicity of Saporin has left cancer drug development companies looking to non-immunogenic payloads.
It is important to point out that Saporin research has evolved from isolating the protein directly from the plant into recombinant expression. This is demonstrated in the cancer research area, where a chimeric fusion protein of the amino-terminal fragment (ATF) of the urokinase-type plasminogen activator (uPA) and Saporin create highly active therapeutic compounds
[17].
1.2. RIPs in Neuroscience Research
The use of targeted toxins in the nervous systems has advantages over cancer systems. For one, the nervous system doesn’t replicate such as tumors do. Trying to target tumor cells that are constantly expanding and spreading to other areas of the body is quite difficult; reaching all these cells requires systemic delivery which can result in an immune response. In the nervous systems, binding by the targeting molecule—whether it is a ligand, antibody, aptamer, or lectin—will cause internalization in nearly all cases, allowing Saporin to enter the cell. Once inside the cell, Saporin will inhibit protein synthesis and the cell will die. Neighboring, non-target bearing cells will remain. Unlike targeting individual tumor cells, the depletion of one neuronal cell type will also affect its pathway. For example, with the use of SP-SAP (Substance P-Saporin), the elimination of the perception of chronic pain is accomplished with a single dose delivered, thereby not causing an immune response. Another advantage to targeting nervous systems is that once cells are removed by a targeted toxin, they are usually not replaced. This allows possibilities for experimental studies on the various cell types with removal and examination of the effect.
2. Targeting Alzheimer’s Disease
Alzheimer’s disease (AD) is the most common form of dementia and has many symptoms that often present themselves gradually. The most talked about are loss of memory, confusion, repetitiveness, and mood and personality changes, and loss of initiative. Signs of severe AD include seizures, increased sleeping and difficulty swallowing, which often leads to aspiration pneumonia, and inevitable death
[18].
Over the years, several animal models of AD have been established to reproduce behavioral changes mimicking those seen in AD. Different types of lesions were produced by employing electrolytes, excitotoxins, or other alkaloid substances that led to non-specific and incomplete killing in several brain areas
[19][20]. Cognitive dysfunction was also induced by alcohol injection
[21] and β-amyloid peptides, which led to inflammation and cholinergic hypofunction
[22]. Verkhratsky et al. stated that when compared to those lesions, the injection of 192-IgG-SAP results in depletion of specific brain areas relevant to AD, which makes it a reliable tool to investigate and understand the etiology of AD and its effect on cognitive performance
[23].
2.1. 192-IgG-SAP
192-IgG-SAP uses the mouse monoclonal antibody 192-IgG, which recognizes the rat low-affinity neurotrophin receptor (p75). This is conjugated to the ribosome-inactivating protein, Saporin. This anti-neuronal immunotoxin permanently eliminates cholinergic neurons of the basal forebrain (CBF), medial septum, and diagonal band of Broca, which provide critical inputs to the hippocampus
[24]. 192-IgG-SAP also targets neurons of the nucleus basalis of Meynert, and Purkinje neurons of the cerebellum, but not projections to the amygdala. The majority of the cholinergic neurons that project to the amygdala do not express p75 and are therefore not affected by 192-IgG-SAP
[25][26]. These choline acetyltransferase (ChAT)-positive/p75-negative neurons can be eliminated with a different Saporin antibody conjugate called Anti-ChAT-SAP. It is important to emphasize that the amount of cell death due to targeted Saporin toxins directly correlates with the number of cells that express the p75 receptor.
2.2. 192-IgG-SAP Species Specific Alternatives
There are two conjugates in addition to 192-IgG-SAP that specifically kill p75 neurotrophin receptor-expressing cells. ME20.4-SAP kills p75NTR-positive neurons in primate
[27], rabbit
[28], and sheep
[29]. In mice, mu p75-SAP eliminates these neurons
[30]. These additional immunotoxins allow for a wider scope, as research can occur in different species.
3. Behavior, Disease and Animal Models
Conjugates of Saporin have been used to create several models that are helpful in academic and pharmaceutical research (Figure 1).
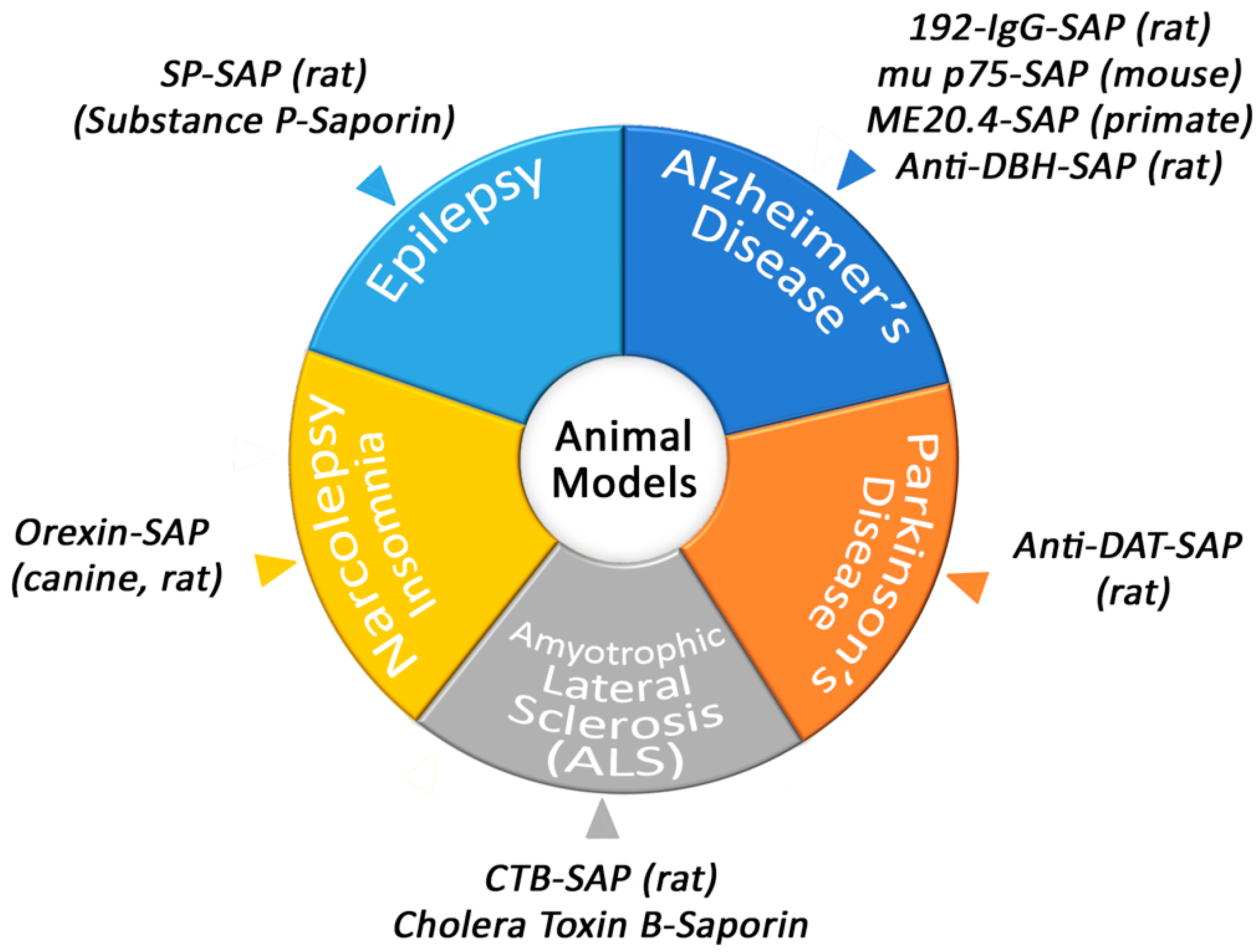
3.1. Narcolepsy/Insomnia
Orexin-B-SAP, a conjugate of the Orexin-B (Hypocretin-2) peptide and Saporin, has been used extensively to study sleep and sleep disorders
[31]. The generation of this tool began when researchers had determined the classic Doberman demonstration of narcolepsy was probably related to the human malady and perhaps genetically closely related. In 1999, these molecules had only recently been recognized by the Sakurai group at the University of Texas Southwestern Medical Center
[32] and the group of J.G. Sutcliffe at the Scripps Research Clinic
[33], and were shown to be involved in feeding behaviors.
3.2. Amyotrophic Lateral Sclerosis
Amyotrophic lateral sclerosis (ALS), also called Lou Gehrig’s disease, is centered around motor neuron deterioration. When these neurons die, muscles can’t function. Cholera toxin subunit B conjugated to Saporin (CTB-SAP) has a specificity towards motor neurons via GM1 ganglioside and has been used in different varieties to mimic complications in ALS. For example, tongue weakness and dysphagia are major complications in ALS patients and a model using CTB-SAP has been employed to induce hypoglossal motor neuron death to mimic the swallowing difficulties
[34].
3.3. Parkinson’s Disease
One of the main ways to research Parkinson’s Disease (PD) is to use the neurotoxin 6-hydroxydopamine (6-OHDA), which selectively destroys dopaminergic and noradrenergic neurons in the brain and can induce Parkinsonism in laboratory animals. Although a valuable tool, there are issues with using this model such as not producing extra-nigral pathology or Lewy body-like inclusions
[35]. A Saporin conjugate, such as Anti-DAT-SAP, that utilizes a monoclonal antibody to the dopamine transporter conjugated to Saporin, can also lesion dopaminergic neurons
[36]. It’s important to note the that the lesioning becomes more specific than 6-OHDA because the lesioning only occurs in places that express the dopamine transporter. Saporin conjugates are as specific as the targeting moiety of the conjugate and therefore there are constantly new targeting agents being attached to Saporin in order to create different, unique, robust, or better targeted toxins.
3.4. Itch
Various Saporin conjugates have been vital in discovering the mechanism behind itch. Understanding the pathway that itch is correlated to is important because one in five people experience chronic itch at least once in their life
[37]. Chronic itch is also poorly treated, and treatments are often ineffective.
3.5. Epilepsy
One of the animal models developed to produce temporal lobe epilepsy in rats has been via systemic injection of pilocarpine. This model, developed by the Turski group in 1983
[38], has certainly been a valuable tool in the field of researching epilepsy. However, the mortality rates of rats are around 30–40% when injecting with the necessary amount of pilocarpine to induce the syndrome
[39]. The high mortality rate of this model and the number of antiepileptic drugs (AEDs) that exist means that there is room for improvement of a model for temporal lobe epilepsy and treating the disease itself.
4. Secondary Conjugates and Streptavidin-ZAP
Immunotoxins made with Saporin have provided robust tools, especially in the fields of neuroscience
[16][19][40] and cancer
[16][41]. As studies in these fields continue to expand, finding a modular way of creating targeted toxins becomes more desirable. Not all laboratories are created equal and having the scientific background, time, and funds needed to produce these tools are often unavailable.
For the synthesis of an immunotoxin, choosing the right antibody is key. Particularly in the area of drug development, large numbers of antibodies need to be screened for suitability. In the year 2000, a tool was developed for evaluating antibody efficacy for use as an immunotoxin. The idea was first suggested by Till et al.
[42] but was not commercially available.
A similar molecule, Mab-ZAP, was prepared at Advanced Targeting Systems (ATS) for commercial use
[43]. Mab-ZAP consists of a polyclonal goat anti-mouse IgG antibody chemically conjugated to Saporin. ATS created the moniker ‘ZAP’ in place of Saporin for all its non-targeted secondary conjugates and has continued to use it in its commercial reagents. Its mechanism of action is simple: Mab-ZAP is mixed with a candidate antibody, the complex binds to a cell-surface antigen, and is internalized. Once internalized, Saporin inactivates the ribosomes, protein synthesis ceases, and this results in cell death. Mab-ZAP doesn’t have a way to enter a cell on its own and so only the cells expressing the specific antigen recognized by the primary antibody candidate is affected.
5. Clinical Trial for Cancer Pain
5.1. The Road to Human Clinical Trials
In 1997, Patrick Mantyh published a paper in the journal
Science entitled “Inhibition of hyperalgesia by ablation of lamina I spinal neurons expressing the substance P receptor”
[44]. This publication made a huge impact in the field of pain and was followed by a series of television, radio, and news reports about this one-shot, targeted drug, a ‘magic bullet’, Substance P–Saporin (SP-SAP), that eliminates chronic pain while leaving acute pain intact (
Figure 2).
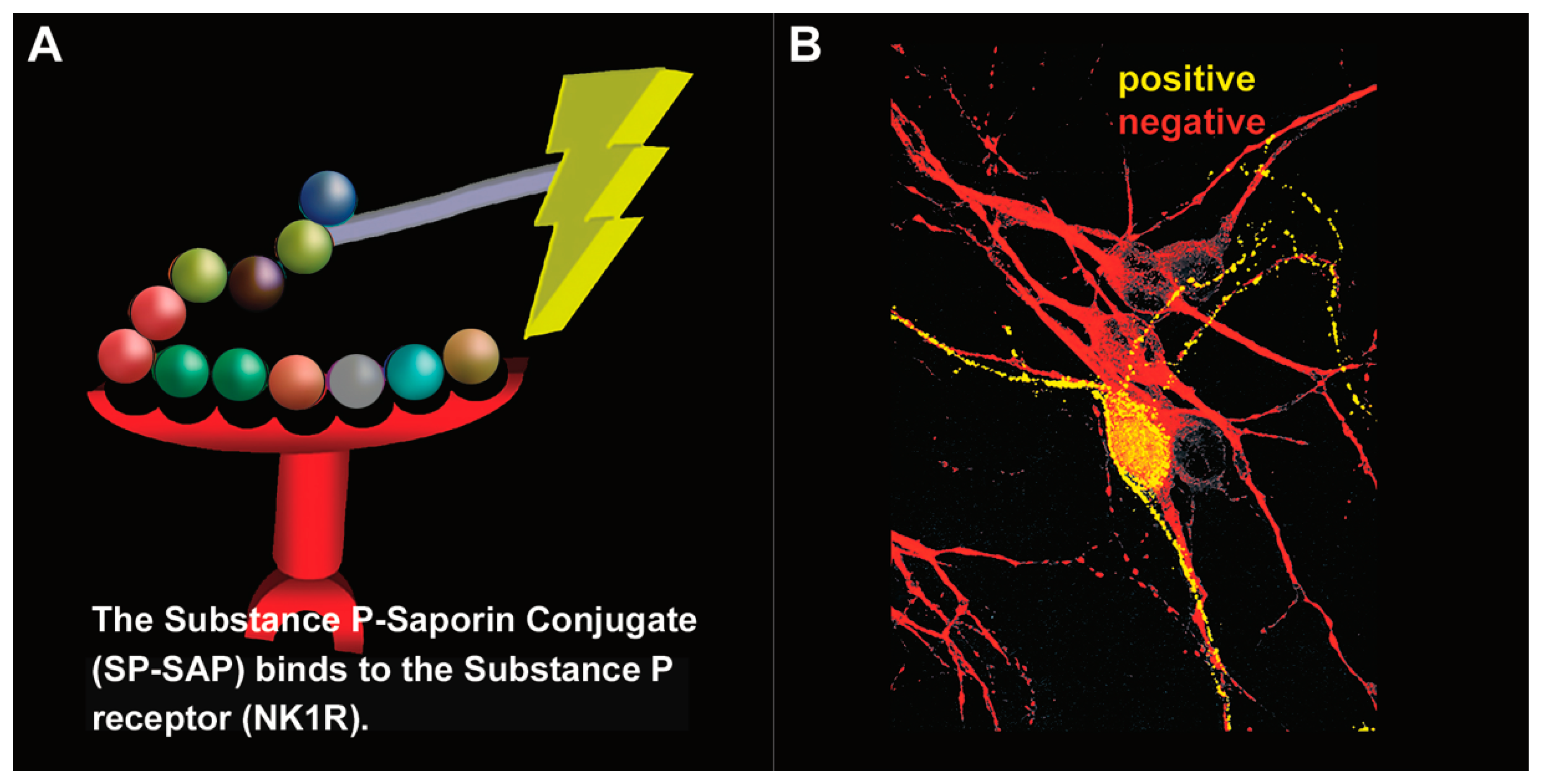
5.2. Human Clinical Trial
In 2008, a pre-IND (Investigational New Drug) meeting was held with the United States Federal Drug Administration (FDA), and the Division of Anesthesia, Analgesia, and Rheumatology Products at the Center for Drug Evaluation (CDER). The meeting was positive and provided clear guidance for the next steps in the areas of chemistry, manufacturing, and controls (CMC), as well as the pre-clinical toxicology requirements for the IND. The CDER review panel indicated that the patient population was appropriate and noted that there was a clear unmet clinical need for drugs addressing a terminal cancer patients’ intractable pain. They determined that a terminal population was appropriate due to the irreversible elimination by SP-SAP of <5% of NK1R+ neurons in the spinal cord
[45].
5.3. Preclinical Work Using Stable Substance P-Saporin (SSP-SAP)
SSP-SAP is a conjugation of Saporin and stable Substance P, the Sar9, Met(O2)11 analog of Substance P. The two amino acid replacements are at two sites of digestion by tissue proteases which allows this peptide to resist peptidase digestion, allowing a greater diffusion from the injection site before it is metabolized. It is considered a ‘new and improved’ version of SP-SAP for research purposes where the targeting and elimination of NK1R+ cells in a larger area is desired; it has not yet been studied sufficiently to progress into veterinary or human trials.