Glioblastoma multiforme (GBM), classified as a grade IV brain tumor, represents the most frequent brain tumor, accounting for approximately 12–15% of all intracranial neoplasms. Current therapeutic strategies for GBM rely on open surgery, chemotherapy and radiotherapy. Despite some progress in the past 30 years, the overall survival of patients with glioblastoma remains extremely poor. The average lifespan is approximately 15 months after diagnosis, with most patients experiencing tumor relapse and outgrowth within 7–10 months of initial radiation therapy.
1. Introduction
There is a pressing need to develop innovative therapy approaches for GBM, to improve clinical outcomes and reduce the rate of relapse and adverse effects intrinsic to the current treatment options.
[1] To tackle this goal, new technologies based on nanometer-sized particles (nanoparticles, NPs) have been studied in the last decade as an attractive alternative to develop innovative tools for the treatment and management of brain cancer
[2]. For this purpose, NPs allow exploration of a variety of core structures (e.g., an organic polymer or a metallic material) and different targeting moieties for specific accumulation and retention in the tumors (e.g., an antibody or a small peptide).
Such research efforts led to the development of NanoTherm, which has been approved recently by the European Medicines Agency (EMA) for thermal therapy of GBM
[3]. NanoTherm consists of an aqueous dispersion of iron oxide nanoparticles, which is injected into the tumor and, following the application of an alternating magnetic field, releases heat locally. However, the need of a dedicated magnetic field applicator (not available in most hospitals) may restrain the spread out of GBM treatments based on NanoTherm. Besides iron oxide nanoparticles, AuNPs are another class of inorganic nanoparticles that were also studied to develop new approaches to treat GBM, namely as radiosensitizers or drug-delivery carriers, because of their good biocompatibility
[4][5][6][7].
AuNPs are very well suited to deliver imaging or therapeutic radionuclides to tumor tissues, taking advantage of a variety of radiolabeling strategies for a stable incorporation of medically relevant radiohalogens or radiometals into the NPs core and/or their targeting moieties
[8][9]. Thus, AuNPs carrying therapeutic radionuclides (e.g.,
103Pd,
177Lu,
211At,
225Ac) started to be envisaged as nanometric seeds for the local delivery of radiation to solid tumors
[10][11][12]. This approach is an attractive alternative to classical millimeter-sized brachytherapy seeds, as it allows the irradiation of the tumor cells with β
− or α particles instead of the X-rays emitted by the classical seeds. These ionizing particles have a higher linear energy transfer (LET) than X-rays; therefore, radiolabeled AuNPs might offer a better chance to circumvent radiation resistance processes. Moreover, AuNPs solutions are expected to facilitate intratumoral administration procedures when compared with the insertion of classical brachytherapy devices. Therefore, nanoseed-based delivery of radionuclides to tumors might contribute to a more intimate/molecular brachytherapy approach, while allowing a versatile combination with other imaging/therapeutic modalities.
In the past few years, several authors have studied radiolabeled nanoseeds for GBM treatment, based mainly on localized intratumoral approaches, including AuNPs. Many of these studies have focused on α emitters, such as
211At,
223Ra or
225Ac, as this class of radionuclides can provide efficient therapeutic outcomes due to the inherent high LET of heavy α particles
[13][14][15][16][17]. However, the limited availability of α emitters still hinders their clinical translation and widespread use. By contrast, several β
− emitters have been in clinical use for several years. In particular, the medium-energy β
− emitter
177Lu (T
½ = 6.647 d, mean energy = 133.3 keV) is emerging as the most important theranostic radionuclide in nuclear medicine, in combination with the β
+ emitter
68Ga used for PET imaging
[8][18][19].
Despite its advantages,
177Lu has only rarely been evaluated in the labelling of nanoseeds for GBM treatment. In the first reported work,
177Lu was used to label Gd-modified fullerene nanoparticles and the resulting radiolabeled NPs demonstrated a dose-dependent increase in survival in a human GBM orthotopic tumor-bearing mouse model
[20]. These Gd-modified fullerene NPs did not contain any GBM-targeting unit to promote the accumulation and retention in the tumor, exploring, alternatively, the enhanced permeability and retention (EPR) effect, that is involved in the passive targeting of leaky tumor tissues
[21]. Later on, Ferro-Flores et al. studied
177Lu-labeled AuNPs decorated with a cyclic Arg-Gly-Asp (RGD) peptide, recognizing the α
vβ
3 integrin receptor, which is not specific for GBM but is involved in tumor angiogenesis
[22]. Following the intratumoral administration of the AuNPs in a murine C6-glioma xenograft model, the authors verified highest tumor retention and enhanced reduction in tumor growth for
177Lu-AuNP-RGD when compared with the same
177Lu-labeled AuNPs without the RGD peptide. These results pointed out that the target-specific approach can lead to improved GBM treatments based on intratumoral application of radiolabeled nanoseeds.
Unlike the α
vβ
3 integrin receptor, the neurokinin-1 receptor (NKR1) is almost exclusively expressed on the glioma cell surface with a consistent overexpression in all primary malignant gliomas
[23]. Hence, the NKR1 has emerged as a good candidate for the development of targeted GBM therapies. The main endogenous ligand of NK1R is substance P (SP) that belongs to the family of tachykinin peptide neurotransmitters
[24]. SP derivatives were labeled with therapeutic radionuclides, including β
− (e.g.,
90Y and
177Lu) and α (e.g.,
213Bi and
225Ac) emitters and using 1,4,7,10-tetraazacyclododecane-1,4,7,10-tetraacetic acid (DOTA) and 1,4,7,10-tetraazacyclododecane-1-glutaric acid-4,7,10-triacetic acid (DOTAGA) bifunctional chelators
[25][26][27]. Several of these radiolabeled SP peptides underwent clinical trials that involved intratumoral or intracavitary therapy cycles. Encouraging results were obtained in terms of median survival when compared with other therapeutic modalities.
For an intratumoral approach, radiolabeled nanoseeds are an attractive alternative to more diffusible molecular systems, such as radiolabeled SP peptides, as they should exhibit highest tumor retention with consequent improvement of therapeutic outcomes. Having this in mind, Bilewicz et al. have designed AuNPs carrying a SP derivative (SP(5–11)) and proceeded with their labeling with the α emitter
211At. The resulting
211At-labeled AuNPs showed high in vitro radiocytotoxicity in T98G glioblastoma cells, moderately larger than that showed in the same cell line by the radiolabeled AuNPs without the SP peptide
[14]. On contrary, to our knowledge, there is no study on
177Lu-labeled AuNPs functionalized with SP peptides as radionanoseeds for GBM treatment, despite the favorable nuclear properties of
177Lu for radionuclide therapy and its greater availability compared with
211At. Aiming to capitalize on these advantages, it was decided to study
177Lu-labeled AuNPs functionalized simultaneously with DOTA chelators for a stable coordination of the radiometal and with SP derivatives for the recognition of GBM cells. For this purpose, AuNP-TDOTA nanoparticles, previously reported were used.
[28]. AuNP-TDOTA are nanoparticles with a small-sized core (ca. 4 nm) containing a thiolated DOTA derivative (TDOTA = 2-[4,7-bis(carboxymethyl)-10-[2-(3-sulfanylpropanoylamino)ethyl]-1,4,7,10-tetrazacyclododec-1-yl]acetic acid), covalently linked to their surface by Au-S bonds, which confers a largely negative zeta potential value to the NPs, thus preventing their aggregation in solution. This is a crucial issue, as AuNPs for biomedical applications need to be stable in physiological environments. If needed, several strategies can be employed to improve the colloidal stability of AuNPs gold nanoparticles, such as their functionalization with different biomacromolecules and polymers or with thiol-containing small peptides
[29][30][31]. As previously shown for bombesin (BBN) derivatives, AuNP-TDOTA can be readily functionalized with bioactive peptides carrying an N-terminal thioctic acid function for their Au-S chemisorption through the terminal disulfide group
[28][32]. The same strategy was used to couple the native SP sequence (SP(1–11)) to AuNP-TDOTA, as well as to attach the congener sequence SPTyr8, where the original phenylalanine at the 8-position was replaced by a tyrosine residue. Previously, it has been shown that the introduction of the tyrosine residue in this position of the amino acid sequence does not compromise the biological activity of the peptide
[33][34]. Therefore, the tyrosyl residue was labelled with radioiodine to ascertain if the targeting vector, the SP derivative, would travel together with the AuNP-TDOTA core carrying a trivalent radiometal, such as
177Lu, in the biological milieu. This important issue has seldom been addressed in the preclinical evaluation of target-specific nanoseeds.
2. Radiolabeled Gold Nanoseeds Decorated with Substance P Peptides
The functionalization of AuNP-TDOTA with SP derivatives aimed to idea was to promote the increased accumulation of gold nanoparticles in human GBM cells by and to enhance the cellular uptake of radionuclides. For this purpose, the presence of the TDOTA coating was explored since it provides stability to the NPs and warrants a stable radiolabeling with a variety of medical radiometals. Moreover, it allows the functionalization of the NPs surface with a reasonably high payload of the targeting vectors. These favorable features prompted us to proceed with the functionalization of the AuNP-TDOTA nanoplatforms with two different thioctic acid derivatives of SP peptides, containing the native SP sequence and the modified SPTyr8 sequence, based on the reaction of the Au atoms at the NPs surface with the disulfide bridges from the incoming peptides.
The reaction of AuNP-TDOTA with thioctic acid derivatives of the SP and SPTyr8 peptides was followed by HPLC analysis to ascertain the amount of peptide attached to the NPs surface. This study showed that there are 0.54 and 0.55 mg of each peptide per mg of the recovered AuNP-SP and AuNP-SPTyr8, respectively. Considering a spherical form for the NPs gold core, with an approximate 4 nm diameter, it can be estimated that each individual AuNP contains an average of approximately ten peptide molecules (SP or SPTyr8) at its surface. Although being in the same order of magnitude, this value is inferior to that reported by Bilewicz et al. for the attachment of a HS-PEG-SP(5–11) peptide to 5 nm sized AuNPs of the citrate type (ca. 40 peptide molecules per NP)
[14]. This difference might reflect the involvement of two Au atoms in the binding of the thioctic acid derivatives used in this work, while the monothiolated sequence HS-PEG-SP(5–11) binds uniquely to one Au atom
[14]. However, the formation of chelated dithiolates in AuNP-SP and AuNP-SPTyr8, instead of monodentate thiolates, has the advantage of providing a more stable binding of the SP derivatives to the AuNPs. In this respect, the Reilly’s group has shown that AuNPs functionalized with DOTA-containing polymers through dithiol or multithiol end groups have greatest stability in vitro and the lowest liver uptake in vivo when compared with the congener AuNPs functionalized using single thiols. This trend was attributed to the greatest inertness of the chelated dithiolates and multithiolates in challenge reactions with endogenous thiolated biomolecules (e.g., dithiothreitol, cysteine or glutathione)
[35].
The designed AuNP-SP and AuNP-SPTyr8 were thought as nanoseeds for internal radiation therapy of glioblastoma, upon labeling with therapeutic radionuclides (e.g.,
125I and
177Lu) and intratumoral administration, in alternative to classical brachytherapy seeds
[36]. The decoration of these NPs with SP peptides for NK1R targeting was expected to promote the internalization in glioblastoma cells to prolong tumor retention and enhance radiobiological effects. SP derivatives are known to have a poor metabolic stability due to their rapid degradation (1 to 2 min) by serum peptidases following their entry into the systemic circulation. However, it has been shown that
90Y
_labeled SP derivatives are stable at the target site for up to 72 h, upon intratumoral administration in GBM tumors
[37][38]. Besides the possible role of the targeting vector in the intratumoral retention of AuNP-SP and AuNP-SPTyr8, the interaction with plasma proteins might also influence their retention and diffusion in tumors. Thus, it studied the in vitro interaction of these AuNPs with HSA and hTf proteins, using several analytical techniques (CD and UV-vis spectroscopy and DLS measurements). Overall, the results of these studies indicated that AuNP-SP and AuNP-SPTyr8 have a low tendency to interact with these plasma proteins. In particular, this trend is well supported by the CD results that pinpointed a low protein coverage of the AuNPs under the tested conditions. For HSA, which showed the best ability to interact with the NPs, a rough estimation indicates that such coverage corresponds approximately to 1 protein molecule per 10 NPs, based on the HSA molecular weight (66,348 Da) and amount of adsorbed protein according to the CD experiments. These findings certainly reflect the presence of a considerable payload of TDOTA chelators and SP peptides at the surface of the AuNPs that minimize their interaction with the proteins and avoid aggregation processes, as verified by CD and DLS measurements.
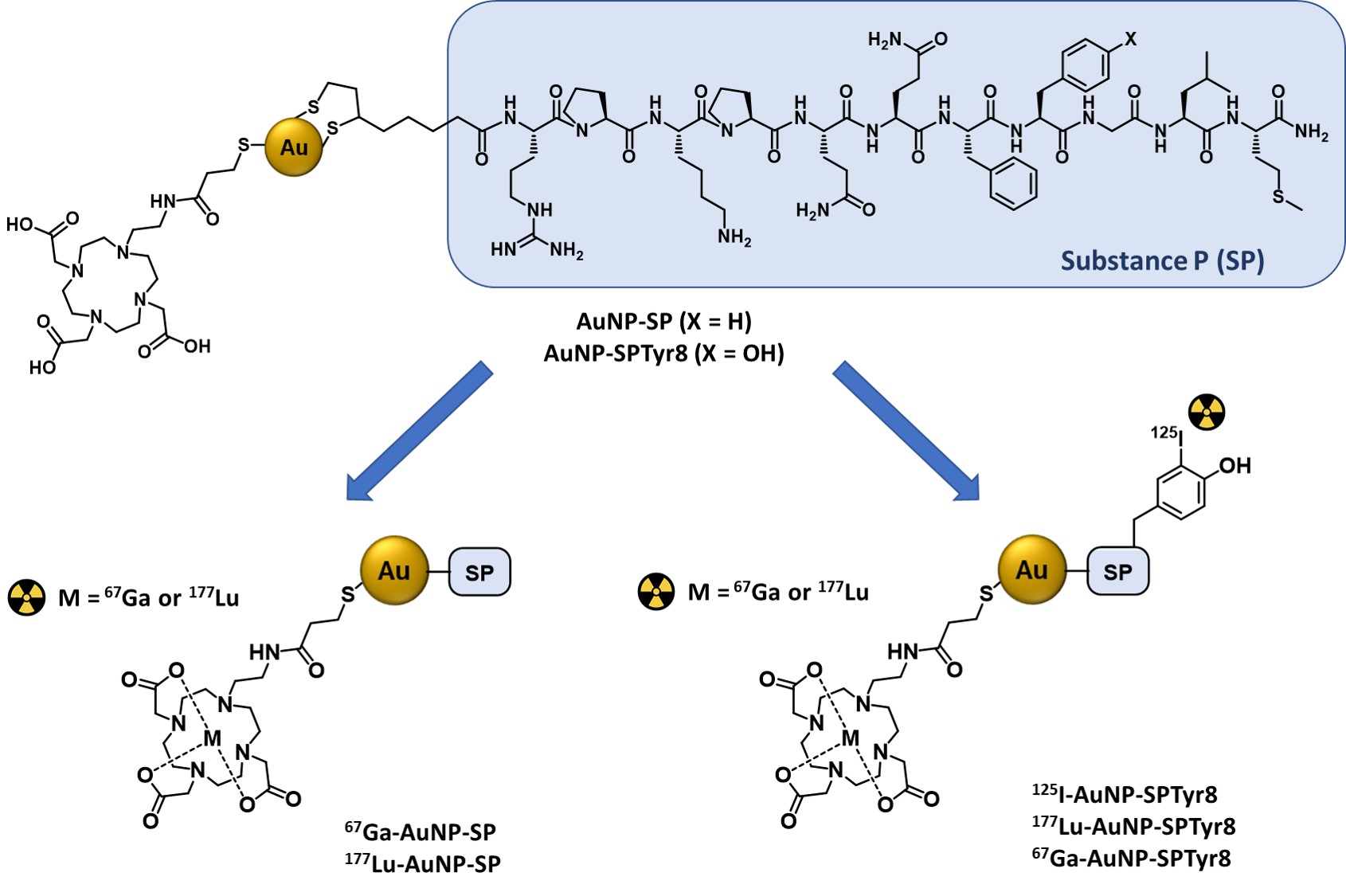
The favorable physico-chemical properties and high in vitro stability of the AuNP-SP and AuNP-SPTyr8 nanoparticles prompted us to pursue their labeling with medical radionuclides (
67Ga,
177Lu and
125I). The labeling with the trivalent radiometals
67Ga and
177Lu proceeded well with radiochemical yields >95%, reflecting the suitability of DOTA chelators to coordinate Ga
3+ and Lu
3+ ions
[39]. For the labeling with
125I, it has taken advantage of the presence of a tyrosyl residue in the SPTyr8 sequence that allowed an efficient electrophilic radioiodination of AuNP-SPTyr8 (ca. 70% radiochemical yield), using the Iodogen method as reported by other authors for the
125I-labeling of RGD-containing AuNPs
[40]. The direct reaction of AuNP-SP or AuNP-SPTyr8 with Na
125I could be an alternative to label these nanoparticles with
125I, as the I
− ion can undergo a strong chemisorption on the gold surface with formation of Au-I bonds
[41]. However, anticipating a stable radiolabeling as typically observed for radioiodinated tyrosine residue, it was preferred to label the SPTyr8 sequence at the AuNP surface. This also implies a better ability to follow the fate of the targeting vector attached to the nanoparticles. Consistently,
125I-AuNP-SPTyr8 and the congener
177Lu-AuNP-SPTyr8 have shown excellent radiochemical stability in the presence of human serum and cell culture medium, as the challenge media. Under the same conditions,
67Ga-AuNP-SP and
67Ga-AuNP-SPTyr8 had a somehow lower radiochemical stability but were still sufficient for their use in subsequent cellular studies. This difference certainly reflects the highest kinetic inertness of Lu–DOTA complexes when compared with the Ga–DOTA congeners, due to the smallest size of Ga
+3 ions
[39].
After confirming the radiochemical stability of the radiolabeled AuNPs, it has pursued with their biological evaluation in GBM cells overexpressing the NKR1. The initial studies were performed with the 67Ga-labeled AuNPs and aimed to evaluate how the presence of the two different SP peptides would influence the cellular internalization of the NPs. Thus, the cellular uptake and internalization of 67Ga-AuNP-TDOTA, 67Ga-AuNP-SP and 67Ga-AuNP-SPTyr8 were studied in T98G and U87 cells. The presence of the SP peptides revealed to be crucial to promote the uptake and internalization of the AuNPs by the GBM cells, with maximal internalization values ranging between 20 and 50% of the applied activity per million cells. By contrast, the non-targeted parental nanoplatform (67Ga-AuNP-TDOTA) presented a negligible internalization (<1%) in both cell lines. These data indicate that the interaction with the NK1R certainly plays an important role in the uptake and internalization of these SP-containing AuNPs.
Notoriously, the replacement of the phenylalanine amino acid by the tyrosine at the 8-position of the SP sequence did not influence the AuNPs ability to be efficiently internalized by the GBM cells. Therefore, the cellular studies were continued with 67Ga-, 125I- and 177Lu-labeled AuNP-SPTyr8 using the tumorigenic U373 cell line. A fast and high uptake and internalization were observed for 125I-AuNP-SPTyr8 and 177Lu-AuNP-SPTyr8, with almost equal values for the different time points, reaching plateau values of approximately 80% of the applied activity per million cells. This result seems to corroborate that there is a stable linkage between the targeting vector and the AuNPs surface under biological conditions, as well as a stable binding of the two different radioactive labels (125I or 177Lu). 67Ga-AuNP-SPTyr8 showed a relatively lower cellular internalization with a maximum internalization value close to 60% of the applied activity per million cells, which is probably due to the lowest radiochemical stability of these 67Ga-labeled AuNPs in the cell culture media.
The high cellular uptake and internalization of the radiolabeled AuNPs in the U373 cells encouraged the evaluation of the radiotherapeutic effects caused by
177Lu-AuNP-SPTyr8 in this cell line. The study was performed in comparison with
177Lu-AuNP-TDOTA that was barely taken up or internalized by the U373 cells. In this way, it intended to assess how the degree of internalization would affect the radiobiological effectiveness of the soft β
− emitter
177Lu. Remarkable differences were observed for the radiobiological effects induced by the non-targeted and targeted nanoparticles, both in the viability and clonogenic assays.
177Lu-AuNP-SPTyr8 reduced much more strongly the cell viability and survival in a dose-dependent manner, highlighting the positive influence of the cellular internalization in the radiobiological effects caused by
177Lu. For both
177Lu-AuNP and
177Lu-AuNP-SPTyr8, more notorious radiobiological effects were found in the clonogenic assay than in the MTT assay, as often observed for ionizing radiation
[42]. It is important to notice that the “cold” (non-radioactive) AuNP-TDOTA and AuNP-SPTyr8 had no effect in the survival of the U373 cells, even for the maximum gold concentration involved in the assays with the
177Lu-labeled congeners.
Finally, microdosimetry studies for the assessment of cellular absorbed doses using Monte Carlo simulations, were performed. The simulations were based on the cellular uptake results obtained for
177Lu-AuNP-TDOTA and
177Lu-AuNP-SPTyr8 and on different scenarios in terms of subcellular localization of the radioisotope and/or its efflux from the target cells. For all the considered scenarios, the calculated doses for
177Lu-AuNP-SPTyr8 were considerably higher than those calculated for the non-internalized
177Lu-AuNP-TDOTA. For the clonogenic assay, the ratios of calculated absorbed doses for
177Lu-AuNP-SPTyr8 vs.
177Lu-AuNP-TDOTA appear in the range 30–450, depending on the considered scenarios. The highest ratios correspond to the scenarios where the
177Lu is located in the nucleus without efflux. As for the experimental results, the ratios of the survival fractions in the clonogenic assays for
177Lu-AuNP-TDOTA vs.
177Lu-AuNP-SPTyr8 spanned between 2.2 and 22.5, attesting the highest radiation dose deposited in the cells by the later in agreement with the Monte Carlo simulations. It should be noticed that the cellular absorbed doses were calculated uniquely based on physical processes of interaction involving the different type of radiation emitted by
177Lu (Auger and internal conversion electrons, β
− particles, X-ray and γ photons), without considering chemical and biological processes. In this respect, radiosensitization effects of the gold nanoparticles can also play a role, as invoked by other authors for radiolabeled AuNPs
[43]. For these radiosensitization effects, the simulations performed in this work showed that the dose enhancement (DE) factors are more relevant in the case of the X-ray photons emitted by
177Lu.
In summary, 177Lu-AuNP-SPTyr8 has emerged in this study as a very promising radiolabeled nanoseed for the treatment of localized GBM upon intratumoral administration. Thus, it deserves a further preclinical evaluation that should include radiotherapeutic assays in GBM xenografts, which should be performed in comparison with the non-targeted congener 177Lu-AuNP. These studies should confirm if the excellent cellular internalization of 177Lu-AuNP-SPTyr8 will translate into augmented tumoral retention and improved therapeutic outcomes. Interestingly, the congener 125I-AuNP-SPTyr8 is expected to have a similar tumor retention and biodistribution profile, based on the tracer principle. Thus, AuNP-SPTyr8 can be seen as a versatile nanoseed for the delivery of therapeutic radionuclides with different emission properties to GBM tumors, including classical brachytherapy radioisotopes, such as 125I. Compared with traditional brachytherapy seeds, AuNP-SPTyr8 is expected to undergo significant local diffusion from the site of injection with a more uniform dose distribution in the tumor. In addition, it allows to obtain enhanced radiobiological effects from all the particles emitted by the radionuclides, due to the intimate molecular contact of the nanoparticles with the tumor tissues. On the contrary, classical seeds fully attenuate short-range particles, such as Auger and internal conversion electrons, capitalizing mainly on penetrating radiation, such as that in X-rays.