Diabetes mellitus (DM), one of the metabolic diseases which is characterized by sustained hyperglycemia, is a life-threatening disease. The global prevalence of DM is on the rise, mainly in low- and middle-income countries. Diabetes is a major cause of blindness, heart attacks, kidney failure, stroke, and lower limb amputation. Type 2 diabetes mellitus (T2DM) is a form of diabetes that is characterized by high blood sugar and insulin resistance. T2DM can be prevented or delayed by a healthy diet, regular physical activity, maintaining normal body weight, and avoiding alcohol and tobacco use. Ethanol and its metabolites can cause differentiation defects in stem cells and promote inflammatory injury and carcinogenesis in several tissues. Studies have suggested that diabetes can be treated, and its consequences can be avoided or delayed with proper management. DM has a greater risk for several cancers, such as breast, colorectal, endometrial, pancreatic, gallbladder, renal, and liver cancer. The incidence of cancer is significantly higher in patients with DM than in those without DM. In addition to DM, alcohol abuse is also a risk factor for many cancers.
1. Introduction
The possible biological links between diabetes mellitus or impaired glucose tolerance and cancer comprise hyperinsulinemia, hyperglycemia, and fat-induced chronic inflammation. DM is a known risk factor for several cancers [1], resulting from insulin resistance induced by a paraneoplastic syndrome [2] or pancreatic β-cell dysfunction [3]. Mechanistically, hyperglycemia may cause hyperinsulinemia, providing growth signals to positively stimulate the expansion of cancer [4][5][6]. In addition, it has been demonstrated that moderate alcohol intake had no significant impact, whereas high alcohol intake was associated with an increased risk of breast and gastrointestinal cancer [7][8][9][10].
According to the National Diabetes Statistics Report, a periodical publication by the Centers for Disease Control and Prevention (CDC), during 1999–2016, the age-adjusted prevalence of total diabetes significantly increased among adults aged 18 years or older. Prevalence estimates were 9.5% in 1999–2002 and 12.0% in 2013–2016. Among the overall US population, the crude estimates for 2018 were that 34.2 million people of all ages or 10.5% of the US population had diabetes. Furthermore, 34.1 million adults aged 18 years or older, or 13.0% of all US adults, had diabetes. Age-adjusted data for 2017–2018 indicated that non-Hispanic blacks (8.2 per 1000 persons) and people of Hispanic origin (9.7 per 1000 persons) had a higher incidence of diabetes compared to non-Hispanic whites (5.0 per 1000 persons). According to the National Institute of Diabetes and Digestive and Kidney Diseases, diabetes is the seventh leading cause of death in the United States.
In 2017, the International Agency for Research on Cancer (IARC) concluded that obesity is a risk factor of cancer of 13 anatomic sites [11]. The direct association of diabetes mellitus with pancreatic, liver, breast, endometrium, bladder, and kidney cancer has been demonstrated. In addition to obesity and diabetes, other risk factors of cancer are alcohol abuse, genetics (family history), smoking, and exposure to toxic chemicals ( Figure 1 ). Recent studies have shown an association between the incidence of cancer and anti-diabetic medications. Furthermore, the use of metformin (a drug for type 2 diabetes mellitus) is associated with a reduced risk of cancer [12][13][14][15][16] or cancer mortality [17].
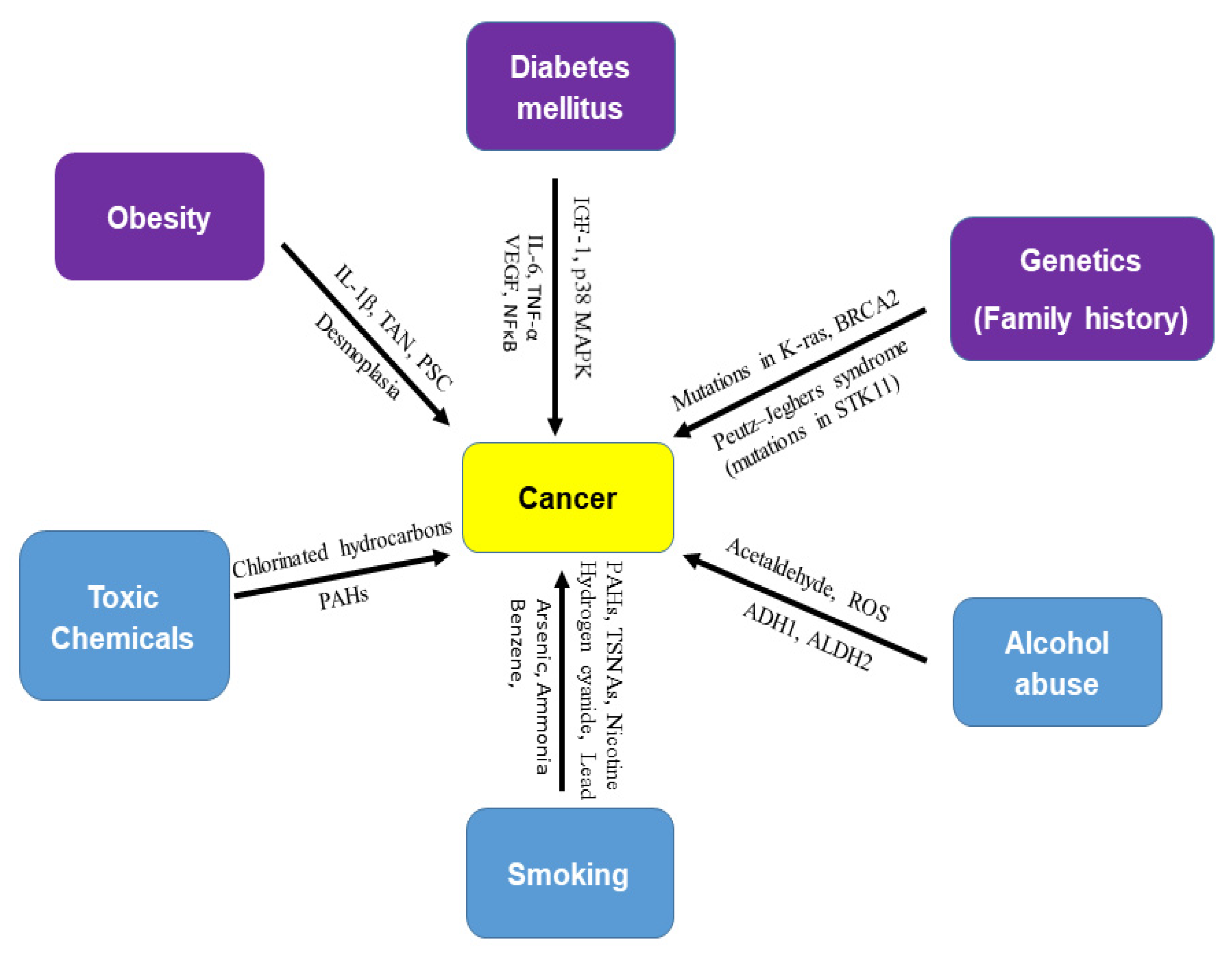
Figure 1. Risk factors of cancer. There are several risk factors for cancer. Obesity (IL-1β, TAN, PSC, desmoplasia), diabetes mellitus (IGF-1, p38 MAPK, IL-6, TNF-β, VEGF, and NF-κB), and genetics (mutations in K-ras, BRCA2, and STK11) are biological risk factors for cancer. Toxic chemicals (chlorinated hydrocarbons and polycyclic aromatic hydrocarbons), alcohol abuse (acetaldehyde, ROS, ADH1, and ALDH2), and smoking (nicotine, hydrogen cyanide, formaldehyde, lead, arsenic, ammonia, benzene, carbon monoxide, nitrosamines, and polycyclic aromatic hydrocarbons), are external or environmental risk factors of cancer. Smoking is known as a strong carcinogen in many cancers. Most cancer cases are attributed to environmental factors but a small percentage are involved in gene mutations and hereditary traces. Peutz–Jeghers syndrome (PJS) is caused by mutations in the tumor suppressor STK11 gene.
2. Diabetes, Alcohol, and Cancer
2.1. Diabetes, Alcohol, and Breast Cancer
Obesity, diabetes mellitus, and unhealthy lifestyle behaviors (alcohol dependence, smoking, low physical activity) are risk factors for breast cancer
[18]. The Mediterranean diet, which consists of fish, monounsaturated fats from olive oil, a high omega-3-to-omega-6 ratio, fruits, vegetables, whole grains, legumes/nuts, and moderate alcohol consumption, has been shown to reduce the risk and prevent the development of depression, diabetes, obesity, and breast cancer
[19][20]. Obese or overweight women at the time of breast cancer diagnosis are at increased risk of cancer recurrence and mortality compared with leaner women
[21][22]. Obesity is associated with adverse outcomes in both pre- and postmenopausal women with breast cancer
[21]. Hyperinsulinemia may promote mammary carcinogenesis. Insulin resistance has been linked to an increased risk of breast cancer and is also characteristic of T2DM
[21]. The Nurses’ Health Study showed that women with T2DM had a modestly elevated incidence of breast cancer (hazard ratio (HR) = 1.17; 95% CI 1.01–1.35) compared with women without diabetes
[22]. Metformin compared to the use of other oral antidiabetic drugs is associated with a lower risk of cancer in patients with T2DM
[23]. Metformin may induce ferroptosis by inhibiting autophagy via lncRNA-H19
[24]. Metformin induced the activation of AMP-induced protein kinase (AMPK), suppressed the phosphorylated-eukaryotic translation initiation factor 4E-binding protein 1 (p-4E-BP1), decreased cyclin D1 levels, and inhibited COX-2 expression
[25]. Metformin inhibits mTORC1 directly or through the inhibition of AMPK. The inhibition of AMPK by metformin results in the induction of p53 and the inhibition of Mdm2. Interestingly, metformin can also downregulate cyclin D1 and upregulate p53 expression through an AMPKα-independent mechanism in breast cancer
[26]. Furthermore, metformin suppresses tumor growth by inhibiting the activation of Akt, MEK/ERK, NFkB, and Stat3.
Drinking within recommended limits is socially acceptable and prevalent in most of the world. It is believed that consuming no more than one alcoholic drink per day, preferably red wine, is beneficial for health. Strong epidemiological data exist showing associations between moderate alcohol consumption and the risk of diabetes and breast cancer. Alcohol intake has been associated with an increased risk of breast cancer and decreased risk of coronary heart disease
[27]. CYP2E1 exerts a significant role in mammary carcinogenesis, and thus provides a potential link between ethanol metabolism and advanced stages of breast cancer
[28]. Factors that are differently distributed among ethnic groups, such as obesity, diabetes, metabolic syndrome, alcohol consumption, and smoking, predict survival after breast cancer diagnosis and therefore might mediate part of the observed social disparity in survival
[29].
2.2. Diabetes, Alcohol, and Pancreatic Cancer
Pancreatic cancer (PC) is one of the ten most common cancers in humans. Most of these cases are pancreatic exocrine cancer; only 1–2% of cases of PC are neuroendocrine tumors. It causes 7% of all cancer deaths. It is the fourth highest cause of cancer-related death in both men and women in the United States each year
[30]. In the United States, the number of new cases of PC was 12.4 per 100,000 men and women per year based on 2009–2013 cases. The five-year survival rate for PC is 7.7% (2006–2012)
[31]. The common risk factors for developing PC are tobacco products, obesity, diabetes, chronic pancreatitis, hereditary conditions, and a family history of PC
[30].
DM, or impaired glucose tolerance, is concurrently present in 50–80% of patients with PC. DM is a known risk factor for PC
[32][33][34], and in another aspect, new-onset DM could be an early manifestation of PC
[1], resulting from insulin resistance induced by a paraneoplastic syndrome
[2] or pancreatic β-cell dysfunction
[3]. In addition, it has been demonstrated that moderate alcohol intake had no significant impact, whereas high alcohol intake was associated with an increased risk of PC
[7][8][9].
There was a three-fold risk of pancreatic malignancy in patients with diabetes
[35]. Consistently, the risk of PC increased with a longer duration of diabetes in a prospective study with a hazard risk of 2.0 in both men and women
[36][37]. In a Taiwanese cohort, diabetes mellitus was associated with a relative risk of 2.75 in relation to developing PC and other gastrointestinal tumors
[38]. It was established that HbA1C is a predictor and prognostic factor in PC
[39], requiring further studies to clarify the function of HbA1C in the pathogenesis of PC. Under-treated DM patients had a higher risk for PC than all DM populations
[40]. New-onset DM is significantly associated with reduced survival, whereas long-standing DM does not affect overall survival significantly
[41]. The new-onset DM could be attributed to the paraneoplastic phenomenon mediated by tumor-secreted products, whereas long-standing DM is induced by non-paraneoplastic factors.
2.3. Diabetes, Alcohol, and Liver Cancer
The major risk factors for hepatocellular carcinoma (HCC), the most frequent histological type of primary liver cancer, are persistent infection with hepatitis B virus (HBV) and hepatitis C virus (HCV), both of which increase the risk of liver cancer to 20-fold
[42]. Other established risk factors include non-alcoholic fatty liver disease (NAFLD), tobacco smoking, alcohol abuse, exposure to aflatoxin-contaminated food, and some rare inherited disorders, including hereditary hemochromatosis
[43][44][45][46][47][48].
Emerging evidence supports a positive association between diabetes and liver cancer
[49][50]. The impact of obesity, genetic factors, and a sedentary lifestyle on liver diseases is shown in
Figure 2.
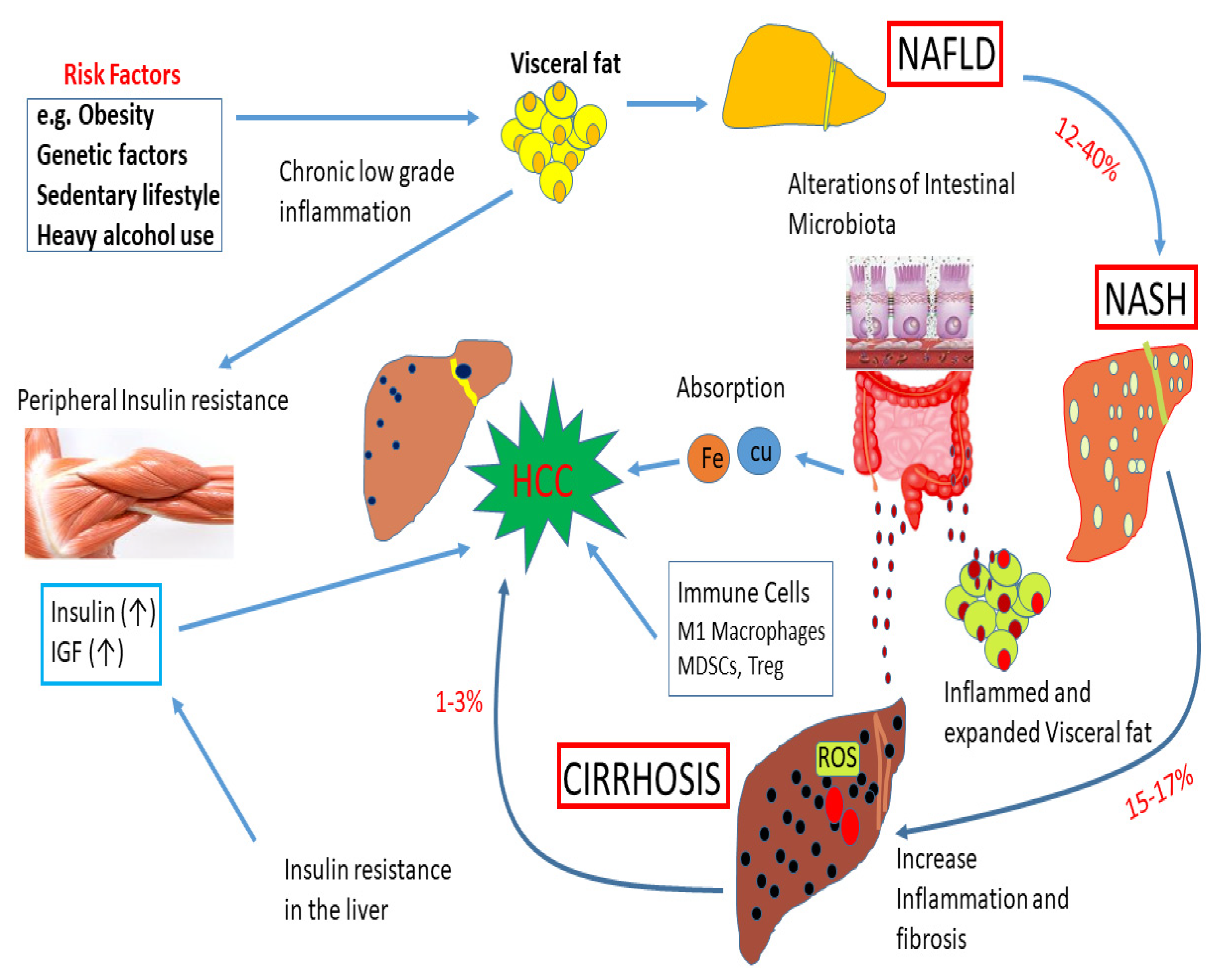
Figure 2. Impact of obesity, genetic factors, and a sedentary lifestyle on liver diseases. Obesity, genetic factors, and a sedentary lifestyle enhance the deposition of visceral fat. These events can play a significant role in the development of non-alcoholic fatty liver disease (NAFLD). About 12–40% of cases of NAFLD develop into NASH. About 15–17% of NASH cases may advance to cirrhosis due to an increase in inflammation and fibrosis. A subset of cirrhosis (1–3%) will finally advance to hepatocellular carcinoma (HCC). High levels of insulin and IGF-1 during insulin resistance can accelerate the development of HCC. Alterations of intestinal microbiota can cause inflammation and visceral fat deposition. The absorption of Fe and Cu in the gut, and immune cells, M1 macrophages, myeloid-derived suppressor cells (MDSCs), and Treg can regulate the development of HCC. In addition, ROS and fatty acid ethyl esters induce cellular damage.
2.4. Diabetes, Alcohol, and Gastric Cancer
The morbidity and mortality rate of gastric cancer has declined rapidly over the past few decades, probably due to the recognition of certain risk factors such as
Helicobacter pylori and dietary and environmental risks factors
[51]. Gastric cancer is more common in men and people aged 50 years or older. Obesity, smoking, and
Helicobacter pylori (
H. pylori) infection are important risk factors
[42][52]. Few studies have focused on the relationship between DM and the development of gastric cancer. Some systematic meta-analysis reviews have shown the higher risk and mortality of gastric cancer in DM patients
[53][54]. However, others have shown that there is no clear association between DM and the risk of gastric cancer
[55][56]. Recently, it was found that DM increases the risk of early gastric cancer development within an average of 70 months of follow-up
[57]. All gastric cancers in this study were identified in patients with gastric atrophy, and no cancer was identified in patients without gastric atrophy, which is a typical presentation of
H. pylori infection. However, a small percentage of gastric atrophy patients will develop gastric cancer. It was also reported that hyperglycemia and HbA1c increased the risk of gastric cancer induced by
H. pylori infection
[58]. The production of ROS, which causes DNA damage
[59], is increased in hyperglycemia, and a high glucose level itself has been shown to contribute to DNA damage not only in vitro but also in patients with DM
[60]. Hyperinsulin in DM has a mitogenic effect by activating the mitogen-activated protein (MAP) or phosphoinositide 3 (PI3) kinase pathway via insulin receptors
[61][62] and the signaling of insulin-like growth factor receptors (IGF-Rs)
[63].
2.5. Diabetes, Alcohol, and Bladder Cancer
Most studies have suggested a positive association between DM and a greater risk of bladder cancer morbidity and mortality, particularly in men
[64][65][66]. A recent cumulative meta-analysis showed that DM was positively associated with bladder cancer mortality in both men and women
[64]. Some studies have provided further evidence of a potential risk of bladder cancer associated with insulin. Insulin enhances bladder cancer cell growth by activating epidermal growth factor and PI3K pathways
[67][68]. Chronic exposure to hyperinsulinemia or hyperglycemia induces tumor cell proliferation and metastasis, which increases insulin-like growth factor (IGF)-1 in diabetic patients, stimulates cellular proliferation, and inhibits apoptosis
[69]. In DM patients with bladder cancer, the differential regulation of cadherin expression and the degradation of glycosaminoglycans were observed. Furthermore, reduced expression of E-cadherin was associated with poor outcomes in bladder cancer patients, which demonstrated an increase in metastasis.
The association between elevated plasma-free fatty acid (FFA) concentrations and insulin resistance has been demonstrated. Although the relationship between FFAs and insulin resistance is complex, a study demonstrated negative correlations between plasma FFA levels and the expression of peroxisome proliferator-activated receptor-gamma cofactor-1 (PGC-1) and nuclear-encoded mitochondrial genes. It was concluded that an increase in FFAs decreases the expression of PGC-1 and nuclear-encoded mitochondrial genes and also enhances the expression of extracellular matrix genes in a manner similar to those of inflammatory diseases
[70].
3. Conclusions
Diabetes, obesity, alcoholism, smoking, chemical exposure, and dietary patterns are closely associated with cancer risk. Although DM and cancer share many common risk factors, several population-based retrospective cohort studies have demonstrated that DM may potentiate gastroenterological carcinogenesis. Thus, avoiding these risks will attenuate the morbidity and mortality of cancers. Furthermore, the discovery of new cancer-related genes is very important for cancer screening strategies and prevention. DM, alcohol misuse, and cancer attract public concern because they are not only medical and health issues but also represent social and financial burdens globally. Therefore, health authorities, social workers, and government agencies should manage DM, alcohol consumption, and cancer in such a way so that these diseases can be prevented and treated simultaneously. Eventually, a better comprehension of pathogenesis and novel therapies should be extensively studied to lower or eliminate DM, alcoholism, and cancer and save human lives.