The gut microbiota is a crucial factor in maintaining homeostasis. The presence of commensal microorganisms leads to the stimulation of the immune system and its maturation. In turn, dysbiosis with an impaired intestinal barrier leads to accelerated contact of microbiota with the host’s immune cells. Microbial structural parts, i.e., pathogen-associated molecular patterns (PAMPs), such as flagellin (FLG), peptidoglycan (PGN), lipoteichoic acid (LTA), and lipopolysaccharide (LPS), induce inflammation via activation of pattern recognition receptors. Microbial metabolites can also develop chronic low-grade inflammation, which is the cause of many metabolic diseases.
1. Introduction
Berg’s publication was intended to systematize the definition of the microbiome. Based on many definitions, the authors proposed an extended one, in which the microbiome is presented as a combination of microbiota and their theater of activity. Microbiota are living organisms and include bacteria, archaea, fungi, protists, and algae. Viruses, phages, plasmids, viroids, prions, and free DNA or RNA are, by definition, not living organisms, so they are not members of the microbiota, but they belong to the microbiome. The term theater of activity refers to the structural elements of microorganisms, their metabolites, and molecules produced by the host and modified by environmental conditions. The microbiome also includes the given environmental conditions. All of these factors create a complex micro-ecosystem on which the health and well-being of the host largely depend [1].
The gastrointestinal tract is inhabited by more than 10^14 microorganisms, most of which have not been identified [2]. The bacteria are classified into 12 different types, of which 93.5% belong to 4 types: Firmicutes, Bacteroidetes, Proteobacteria, and Actinobacteria [3]. The gut microbiota plays a major role in digestion, regulation of the immune system, and production of compounds that might alter human metabolism. The microbiota also performs many other functions, i.e., synthesizing vitamins, creating appropriate environmental conditions influencing the oxygen level and pH in the intestines, competing with pathogens (thus reducing their number), and stabilizing the intestinal barrier [4].
The scientific literature shows that the intestinal microbiota can stimulate the immune system and, in the case of excessive stimuli, affect the formation of inflammation.
2. Microbiota-Derived Inflammation
Inflammation is a natural response of the body that is triggered by harmful stimuli, and its evolutionary purpose is to restore homeostasis. Inflammation can be triggered by external and internal inducers. The external inducers are divided into microbial or non-microbial factors. Non-microbial factors include allergens, irritants, and toxic compounds. Two main microbial factors that trigger inflammation are pathogen-associated molecular patterns (PAMPs) and virulence factors. Virulence factors are molecules that occur in pathogens
[5]. PAMPs, however, are small molecules with conserved patterns that occur among various microorganisms. PAMPs include components that build cell walls, including bacterial peptidoglycan, lipopolysaccharide (LPS), lipoteichoic acid (LTA), and flagellin
[6]. Other factors classified as PAMPs include intracellular pathogens like viral RNA or DNA. PAMPs are recognized by pattern recognition receptors (PRRs), which occur on the surface and in the cytosol of immune and epithelial cells
[7]. The families of receptors belonging to PRRs are TLR, NLR, C-type lectin receptor (CLR), retinoic acid-inducible gene (RIG)-I-like receptor (RLR), and the absent in melanoma 2 (AIM2)-like receptor (ALR). The bond of PAMPs with their respective specific receptors activates the innate immune system, which plays a crucial role in first-line defense
[8].
2.1. Pathogen-Associated Molecular Patterns
LPS is a component of the outer cell wall of Gram-negative bacteria and is the best-known bacterial endotoxin. It protects bacteria against harmful factors, such as antibiotics or immune cells, of the host from the external environment. The membrane receptor CD14 is on the surface of cells of the immune system and epithelial cells. It is also available in a soluble form in serum. Lipopolysaccharide binds to a lipopolysaccharide-binding protein (LBP) to increase the affinity for CD14 receptors. The LPS/LBP/CD14 complex, in turn, binds to the myeloid differentiation factor 2 (MD-2) and, in this form, is recognized by the toll-like receptor 4 (TLR4). Activation of this receptor leads to the release of mediators including MyD88, which stimulates nuclear factor κB (NF-κB) to produce proinflammatory cytokines, including tumor necrosis factor α (TNF-α) and interleukin 1β (IL-1β)
[9]. Paradoxically, inflammation enhances intestinal barrier disruption and causes increased gut permeability, which exacerbates chronic low-grade inflammation
[10]. This process is shown in a simplified way in
Figure 1. The increased plasma LPS levels associated with chronic inflammation are called metabolic endotoxemia. This condition leads to the development of cardiometabolic diseases. Endotoxemia is characterized by 2–3-fold higher serum LPS levels (10–15-fold lower serum LPS levels than in sepsis), which is a life-threatening condition
[11]. Endotoxemia is associated with a variety of diseases, including obesity, type 2 diabetes, non-alcoholic fatty liver disease, chronic kidney disease, and cardiovascular disease
[12].
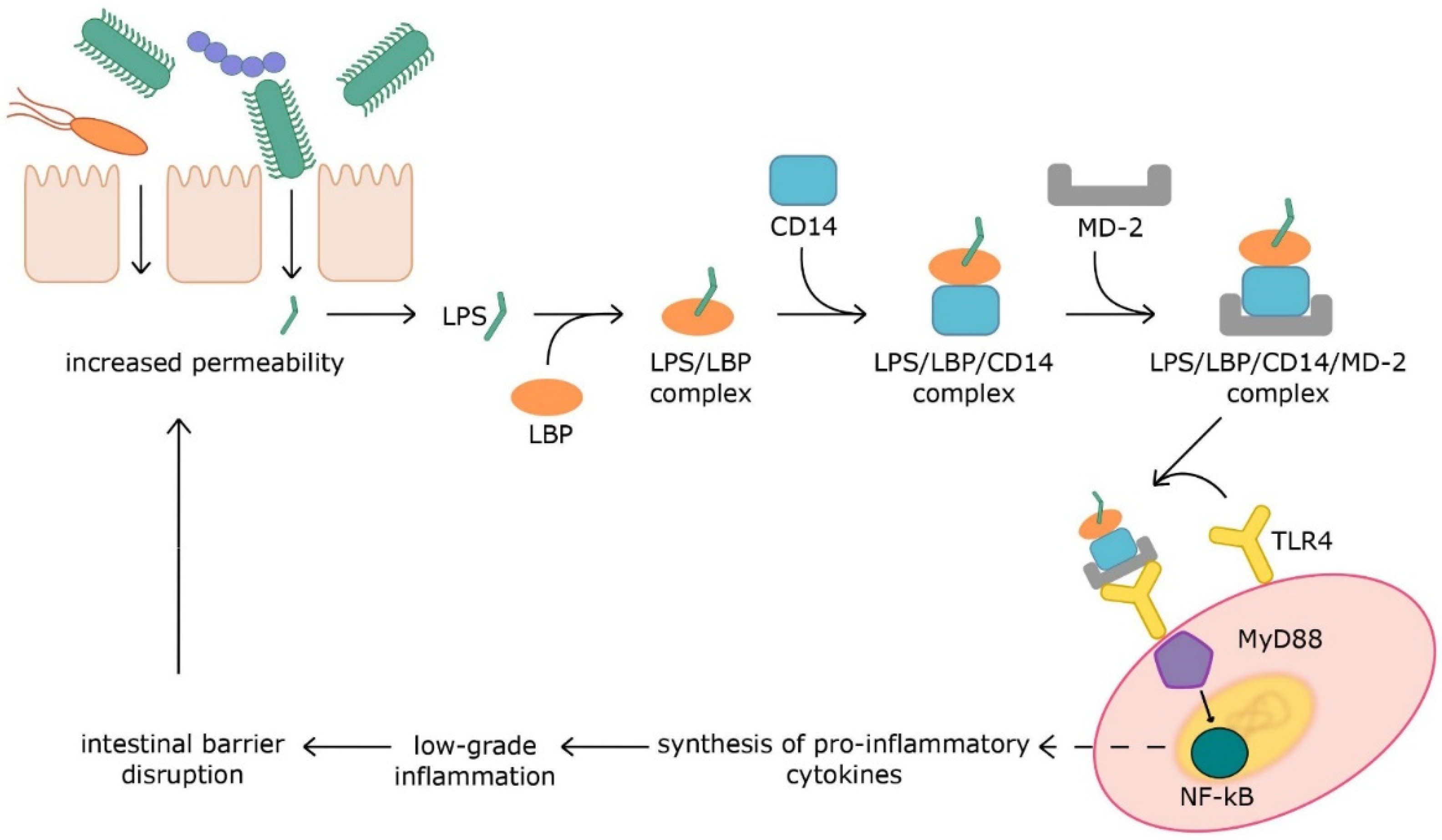
Figure 1. Mechanism of LPS’ influence on inflammation and intestinal permeability. Aberrations: LPS—lipopolysaccharide; LBP—LPS-binding protein; CD14—cluster of differentiation 14; MD-2—myeloid differential factor 2; TLR4—toll-like receptor 4; MyD88—myeloid differentiation factor 88; NF-κB—nuclear factor kappa-light-chain-enhancer of activated B cells.
The receptor that recognizes the bacterial protein of flagella (flagellin) is toll-like receptor 5 (TLR5). Flagellum, a microbial component for locomotion, is mainly found in Gram-negative bacteria
[13]. TLR5 is produced in immune cells and epithelial cells of the gastrointestinal tract, respiratory tract, and liver. It is mainly expressed on the basolateral side of gut epithelial cells, and its role is to detect the translocation of bacteria across the endothelial barrier
[14]. The activation of TLR5 by flagellin results in the induction of the synthesis of chemokines, nitric oxide (NO), hydrogen peroxide (H
2O
2), and proinflammatory cytokines
[13]. Overactivation of TLR5 may contribute to impairment of the intestinal barrier integrity and cause chronic inflammation
[14]. The composition of the microbiota associated with inflammation is characterized by an increased number of motile bacteria, including bacteria with flagella, which more easily penetrate the mucosa and initiate an inflammatory response. A study using a mouse model showed that administration of anti-flagellin antibodies prevents interleukin 10 (IL-10) deficiency-induced colitis and reduces diet-induced obesity
[15]. Lodes et al. found that bacterial flagellin was a dominant antigen in mice with Crohn’s disease, a disease characterized by chronic intestinal inflammation
[16].
Peptidoglycan (PGN) is a significant cellular structure that protects bacteria from environmental factors. It is also necessary for bacterial growth. Peptidoglycan is a complex polymer consisting of a network of glycan strands that gives the cell mechanical strength. PGN also consists of other compounds, such as lipoproteins, polysaccharides, and glycolipids, that give bacteria various physical and chemical properties. The Gram-negative and Gram-positive bacteria have a differently organized peptidoglycan structure. In the first group, peptidoglycan is thinner and is located between the outer and cytoplasmic membranes. However, in Gram-positive bacteria, peptidoglycan is significantly thicker and occurs on the outer side of the cytoplasmic membrane, creating the outermost barrier separating the cell from the environment
[17]. Mammals produce four peptidoglycan recognition proteins (PGLYRPs), which are secreted by immune and epithelial cells and lead to bacterial cell wall lysis
[18]. The peptidoglycans found in the intestinal lumen activate Paneth cells to synthesize defensins, which allows the regulation of the microbiota and protection against pathogens, such as
Salmonella enterica. The recognition of peptidoglycan by NOD1 and NOD2 receptors leads to activation of NF-κB and the mitogen-activated protein kinase (MAPK). Both pathways contribute to the transcription of pro-inflammatory genes, leading to the synthesis of cytokines, adhesion molecules, and inflammatory mediator enzymes. Another signaling pathway involving the activation of cryopyrin, a NOD family protein, also leads to the activation of NF-κB as well as caspase-1-dependent maturation and secretion of the cytokines IL-1β and interleukin 18 (IL-18)
[19]. In 2019, the journal
Nature published an article in which researchers inhibited the development of autoimmune encephalomyelitis and autoimmune arthritis in mice by neutralizing peptidoglycans in the circulatory system. This suggests that peptidoglycans may participate in the pathogenesis of autoimmune diseases
[20].
LTA, a component of a Gram-positive bacteria’s cell wall, is recognized by TRL2 and leads to immune system activation and the development of adaptive immunity. Similar to the other PAMPs, in the case of an excessive immune response, inflammation also develops
[21]. LTA is recognized by TLR-2 and, thus, activates the signaling pathway, leading to the expression of cyclooxygenase, which contributes to prostaglandin E2 (PGE2) synthesis
[22]. PGE2, via activation of mast cells, elicits edema formation and vascular permeability, two main symptoms of inflammation. It is also involved in gene regulation leading to cytokine signaling
[23]. In macrophages, LTA has been established to stimulate the release of interleukin 1 (IL-1), interleukin 6 (IL-6), and TNF-α
[22]. The origin of PAMPs and their recognizing receptors are presented graphically in
Figure 2.
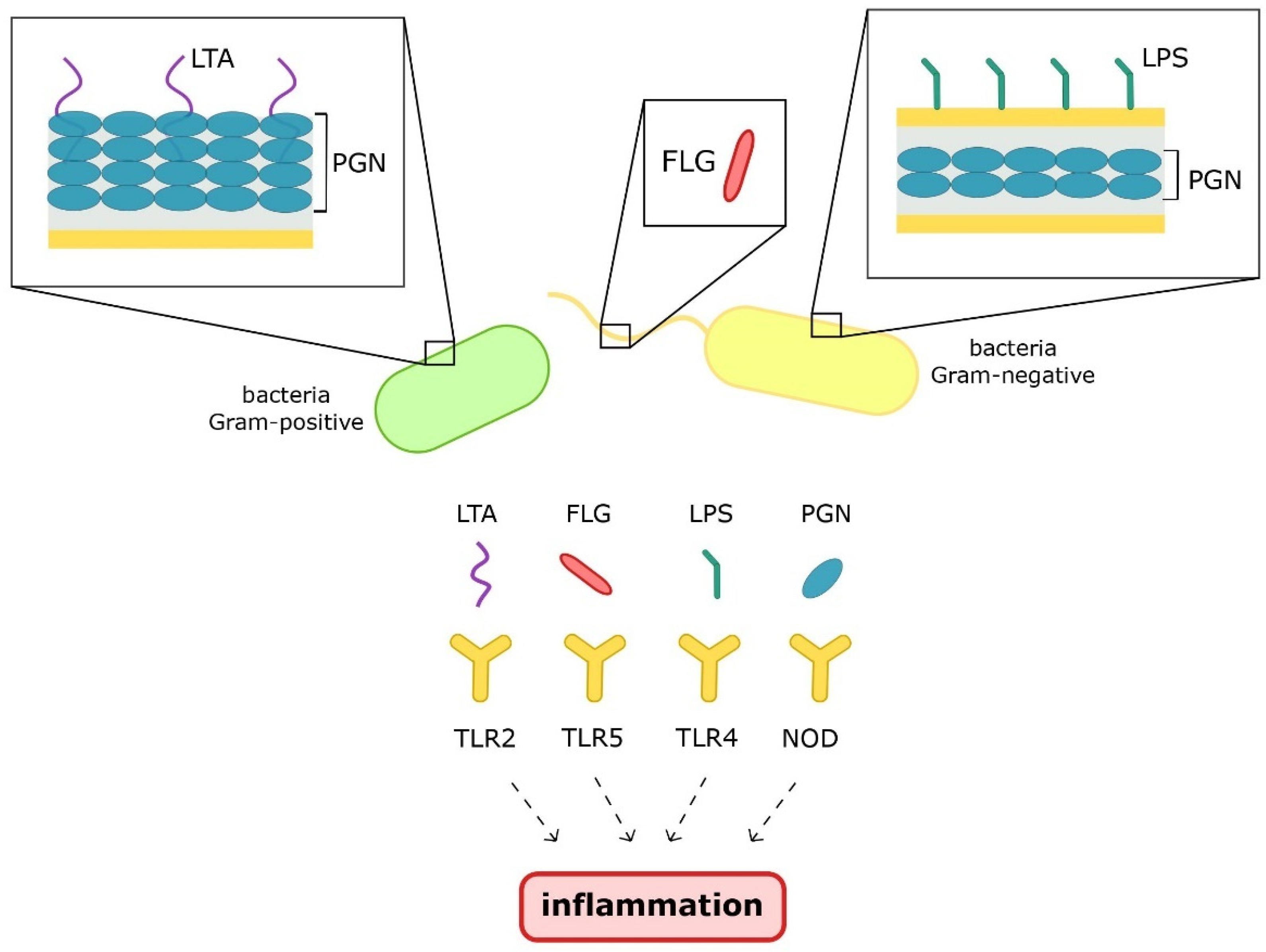
Figure 2. Origin and receptors of pathogen-associated molecular patterns. Aberrations: LTA—lipoteichoic acid; PGN—peptidoglycan; FLG—flagellin; LPS—lipopolysaccharide; TLR2/4/5 –toll-like receptors2/4/5; NOD—nucleotide-binding oligomerization domain.
2.2. Metabolites
Microorganisms can interfere with the host’s immune system and contribute to inflammation not only through their structural elements but also through the products of their metabolism. Short-chain fatty acids are related to several processes that positively influence the metabolism of the host. Butyrate nourishes intestinal endothelial cells and increases the thickness of mucin, which improves the tightness of the intestinal barrier
[4]. Propionate stimulates L-enteroendocrine cells to release glucagon-like peptide 1 (GLP-1) and peptide tyrosine-tyrosine (PYY), which leads to inhibition of appetite. In the liver, it inhibits the synthesis of cholesterol and fatty acids, which reduces the chances of developing obesity and associated diseases. However, not all SCFAs have a beneficial effect on the host. Acetate is attributed to properties that worsen the metabolic state and contribute to the formation of obesity. In the liver, acetate contributes to the synthesis of lipids, which can lead to dyslipidemia. This compound also increases the appetite by increasing the production of gastric ghrelin
[24].
Trimethylamine N-oxide (TMAO), a bacterial metabolite produced during choline, betaine, and L-carnitine refinement, is associated with an increased risk of cardiovascular disease. It has been established that elevated levels of TMAO induce NLR family pyrin domain-containing 3 (NLRP3) activation. NLRP3 belongs to the inflammasomes, which are intracellular protein complexes responsible for initiating inflammatory processes. Their function is to regulate the maturation and secretion of the proinflammatory cytokines IL-1β and IL-18 through the activation of caspase-1. In addition, TMAO, by activating NF-κB, causes the synthesis of proinflammatory proteins with pro-atherosclerotic properties, such as cyclooxygenase 2 (COX2), E-selectin, IL-6, and intracellular adhesion molecule 1
[25].
The intestinal microbiota causes the deconjugation of bile acids in the intestine, resulting in the formation of secondary bile acids, including deoxycholic acid (DCA). It has been shown that a high-fat diet can increase DCA levels up to 10 times. Increased DCA levels are associated with impaired intestinal epithelial integrity along with gut inflammation. It has been indicated that the development of intestinal inflammation is due to the activation of the NLRP3 inflammasome
[26]. In addition, high levels of secondary bile acids (BAs) contribute to the formation of reactive oxygen and nitrogen species. BAs also damage cell membranes, mitochondria, and DNA, which may result in an increased risk of colon cancer. Bacterial endotoxins and metabolites can synergistically increase their harmful properties. The accumulation of DCA and LTA amplifies signals caused by TLR2 activation, leading to overproduction of COX2, which is associated with the suppression of natural killer T and dendritic cells and may lead to cancer and inflammatory diseases
[27].
The preferred energy source for most gut microbiota is carbohydrates, but if carbohydrates are insufficient in the colon, proteins are fermented. The products of these transformations are numerous toxic bacterial metabolites, including p-cresol sulfate (pCS) and indoxyl sulfate (IS)
[28]. An increase in IS and pCS levels in serum is characteristic of patients with chronic kidney disease. In these patients, a positive correlation has been found between circulating IS and pCS and vascular stiffness and calcification, which means a greater risk of cardiovascular disease and the progression of chronic kidney disease. In turn, TMAO, IS, and pCS (as compounds with uremic toxicity) lead to dysbiosis and increased intestinal permeability, which intensifies the inflammation that is already taking place in the host organism
[29].
3. Conclusions
An unhealthy diet, diseases, lack of exercise, sleep disorders, exposure to nicotine, drugs, and many other factors can lead to an imbalance in the gut microbiota
[30]. Dysbiosis and increased intestinal permeability may contribute to inflammation and lead to metabolic disorders or exacerbate existing conditions. Inflammation can be caused by the presence of microorganisms and their structural elements and the products of their metabolism. Low-grade chronic inflammation and dysbiosis intensify each other, creating a vicious circle. Modifying the microbiota by changing the diet and using prebiotics and probiotics might restore microbiome balance and reduce inflammation along with improving metabolic status. However, the composition of the intestinal microbiota is unique for every human being. As a result, there is no universal method of microbiota modification that would bring health benefits. Attempts to modify the microbiota should be based on the type of dysbiosis
[31]. However, the current state of knowledge on the subject is not sufficient and more research is required on many levels to understand how to modify the microbiota to improve the inflammatory profile.