Retinoic acid (RA) is an important biological metabolite synthesized from the retinol content (known as “vitamin A”) via a sequential cellular process in the retinoid signaling pathway (RSP). RSP-mediated RA biosynthesis is a vital physiological process in chordates, since RA interacts with the nuclear receptor superfamily, namely nuclear RA receptors (RARs) and retinoid X receptors (RXRs), bound to the RA response elements (RAREs) in the promoter region of RA target genes. Retinaldehyde dehydrogenases (RALDH) belongs to the oxidoreductase family and plays a critical role in RA synthesis from the retinaldehyde content; therefore, this enzyme is considered to be one of the key regulators of RA-related retinol metabolism and embryonic development.
1. Pleiotropic Roles of Retinoic acid (RA) and RALDH Inhibitors
The RALDH may be categorized into different classes of aldehyde dehydrogenase (ALDH) superfamily, including class 1 (cytosolic proteins), class 2 (mitochondrial proteins), class 3 (tumor-related proteins, inducible, or found in the endoplasmic reticulum), and microsomal aldehyde dehydrogenase
[1][2][3]. The structures of RALDHs had been determined in previous studies
[3][4][5]. RA biosynthesis and signaling seem to be limited to chordates, due to the existence of RALDH
[6]. The
ALDH1
A1,
ALDH1
A2, and
ALDH1
A3 genes code cytosolic RALDH enzyme (RALDH1, 2, 3) that catalyzes the synthesis of retinoic acid (RA) from retinaldehyde. The RNA and protein expression patterns of RALDH in human tissues can be verified in the transcriptomic and proteomic database of THE HUMAN PROTEIN ATLAS
[7]. According to data, differentially expressed RNA and RALDH levels were determined in human tissue cell lines including brain, eye, endocrine tissues, lung, proximal digestive tract, gastrointestinal tract, liver and gallbladder, pancreas, kidney and urinary bladder, female and male tissues, muscle tissues, adipose and soft tissue, skin, bone marrow and lymphoid tissues, and blood. Among these, male tissues including ductus deferens, testis, epididymis, seminal vesicle, prostate, and female tissues such as the vagina, ovary fallopian tube, endometrium, cervix, uterine, placenta, breast showed relatively higher expressions of RNA and protein expression than the other tissues. Furthermore, endocrine tissues, lungs, and pancreas also exhibited relatively higher enzyme expression levels than those of other human tissues [
https://www.proteinatlas.org/ENSG00000128918-ALDH1A2/tissue (accessed on 14 October 2021)]. Meanwhile, in the embryonic cells, three types of cytosolic RALDHs (RALDH1, 2, 3)-coding genes differentially expressed during the early embryogenesis
[8]. According to a previous study by Niederreither et al.
[8],
ALDH1
A1 expression was increased in developing lung, and stage-specifically expressed in stomach and intestine epithelial and mesenchymal layers of embryonic cells. On the other hand,
ALDH1
A2 was expressed in the kidney nephrogenic zone, and
ALDH1
A3 was specifically expressed in the intestinal lamina propria.
Due to the physiological importance, RALDH inhibition by diverse chemicals during the early embryogenesis potentially causes serious disease such as fetal alcohol spectrum disorder (FASD), congenital diaphragmatic hernia (CDH), and Moyamoya disease (MMD)
[9][10][11]. Kot-Leibovich and Fainsod
[10] reported that the alcohol (ethanol) compete with available RALDH activity during embryonic development, thereby inducing abnormally lowered RA level, and finally FASD. Mey et al.
[9] also previously reported that RALDH2 inhibitors including nitrofen, 4-biphenyl carboxylic acid, bisdiamine, and SB-210661 induced posterolateral defects in the rat diaphragm. Furthermore, abnormal organ developments such as eye, kidney, and brain also can be induced by RALDH-inhibition, and thus potentially cause population decline of chordate in the ecosystem. Therefore, the regulation of RALDH inhibitors needs to be re-considered for the improvement of public health.
The physiological importance of RA is explained by pleiotropic modulation, including early embryogenesis, cell differentiation, and innate and adaptive immune homeostasis as shown in
Table 1. Among the diverse roles of RA, morphogenesis and organogenesis during vertebrate development have been widely recognized over the past few decades
[12][13][14][15][16][17][18]. Wilson et al.
[19] had reported that retinoic acid-free embryos exhibited abnormal development of neural tube with alteration in the actin filaments and junctional complexes of the cell layer. Wang et al.
[20] had previously shown that RALDH2-deficient mouse embryo exhibited abnormal development of posterior foregut derivatives (stomach and duodenum) along with liver growth. The relationship between mammalian craniofacial morphogenesis and RA signaling had also been reported in a previous review
[21]. Furthermore, the RA-mediated
Hox genes and adhesion molecule-induced skin morphogenesis via determination of axial orientation had been reported in an embryonic chicken skin explant culture
[22]. RA-mediated epidermal morphogenesis had been reported by Asselineau et al.
[22]. They had shown the delipidized serum-exposed abnormal human keratinocytes to be restored by RA addition, whereas higher RA concentration (>10
−7 M) reduced epidermal maturation and produced parakeratosis
[22]. Multiple roles of RA signaling for mammalian eye development had been reported in previous reviews
[23][24]. RA signaling was shown to not only be required for interactions between the invaginating lens placode and optic vesicle, but also to promote the development of ventral retina and optic nerve, thereby modulating eye and photoreceptor development
[25][23][24]. Dupé and Lumsden
[26] had reported that the RA-mediated transforming signal is involved in the posterior hindbrain structure expansion, and that RA signaling is related to rhombomere boundaries, which are transverse developmental units of the embryonic hindbrain. Morphogenesis during embryonic development is regulated by diverse RA target genes. The enhanced key target genes expressed by RA signaling during early organogenesis include
Hoxa1,
Hoxb1,
Hoxa3,
Hoxd4,
vHnf1,
Pax6,
Olig2,
Cdx1,
Pdx1,
Hoxa5,
Pitx2,
Ret, and
Stra8, whereas the repressed target genes include
Fgf8 and
TGF-ß1 [6]. These genes are highly related to the vertebrate developmental process. Specifically, over-expression of
Hoxa1,
Hoxb1,
Hoxa3,
Hoxd4, and
vHnf1 genes is highly relevant to hindbrain anterior-posterior patterning developmental process, whereas that of
Pax6 and
Olig2 induce spinal cord motor neuron differentiation
[6][27][28][29][30]. Repressed
Fgf8 and overexpressed
Cdx1 genes induce early somite formation, and heart anteroposterior patterning during embryonic development
[6][31]. While the induction of meiosis is related to the
Stra8 gene, anterior eye formation and kidney formation are relevant to the overexpression of
Pitx2 and
Ret genes, respectively
[6][32].
Table 1. Physiological roles of retinoic acid (RA) in chordate.
|
Role of Retinoic Acid (RA) |
References |
Embryogenesis |
Neural tube development |
[19] |
Posterior foregut derivatives development and liver growth |
[20] |
craniofacial morphogenesis |
[21] |
Skin morphogenesis |
[22] |
Eye development |
[23][24] |
posterior hindbrain structure expansion |
[26] |
Early somite formation |
[6][31] |
Heart anteroposterior patterning |
[6][31] |
Kidney formation |
[6][32] |
Others (immunity, cell differentiation) |
Neuron differentiation |
[6][14][27] |
Anti-inflammatory naïve T cells differentiation |
[33] |
HL-60 cells differentiation |
[34] |
2. RA Signaling-Associated Eye Development and RALDH-Relevant AOP (AOP297)
The reciprocal relationship between RA signaling and mammalian eye development has been well-described over the past few decades
[23][35][36][37][38][39].
Eye development in vertebrates occurs in the neural ectoderm, surface ectoderm, and neural crest-derived periocular mesenchyme of the embryonic cell
[23]. As shown in the cross-section of neural ectoderm, pre-placodal ectoderm, and epidermis (
Figure 1), mammalian eye development starts with the formation of a wall of the forebrain at either side of the optic grooves. The optic grooves gradually deepen as the neural folds become elevated, and the neural tube is closed by the surface ectoderm. The optic grooves extend to the near ectoderm to form early lens placode by thickening of the adjacent area. During the interaction of optic vesicles with the ectoderm, the generated lens placode invaginates to form the lens pit, and optic vesicles invaginate to form the double-layered optic cup. The lens vesicles are generated from the lens pit, are surrounded by the double-layered optic cup (
Figure 2), and finally, become the fetal lens and mature lens after the development of primary lens fibers, secondary lens fibers, lens suture, and lens nucleus. A detailed explanation of vertebrate eye development has been well-described in previous reviews
[40][41].
Figure 1. Schematic diagram of mammalian eye development. Eye development in vertebrates occurs in the neural ectoderm origin, surface ectoderm, and neural crest-derived periocular mesenchyme of embryonic cell. Mammalian eye development starts with formation of the wall of the forebrain on either side of the optic grooves. The optic grooves gradually deepen as the neural folds become elevated, and the neural tube is closed by surface ectoderm. The optic grooves extend to the near ectoderm to form early lens placode by thickening of the adjacent area. During the interaction of optic vesicles with the ectoderm, the generated lens placode invaginates to form the lens pit, and optic vesicles invaginate to form the double-layered optic cup. Lens vesicles are generated from the lens pit and are surrounded by the double-layered optic cup; finally, the generated lens vesicle becomes the fetal lens and mature lens after the development of primary lens fibers, secondary lens fibers, lens suture, and lens nucleus.
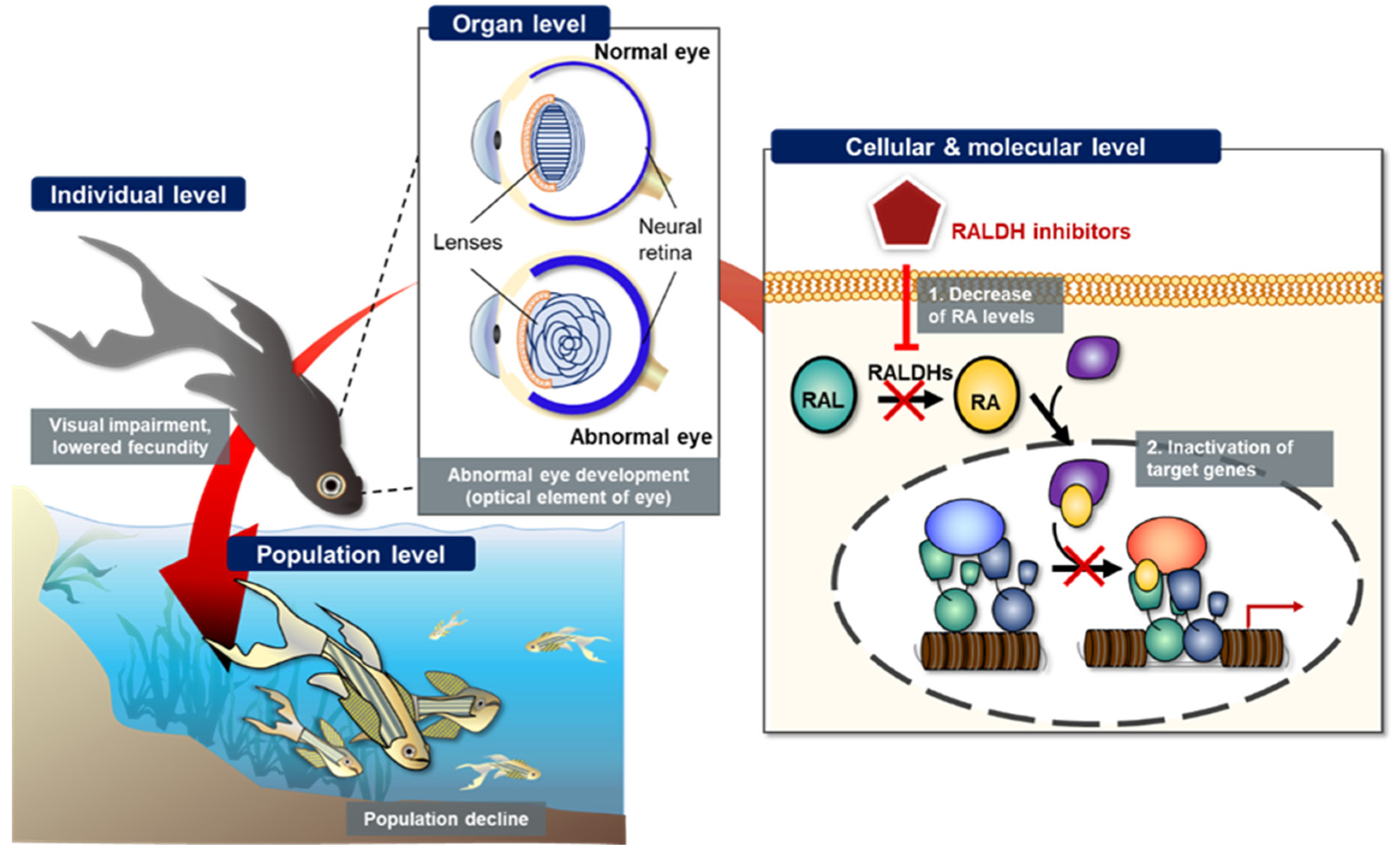
Figure 2. Schematic representation of potential retinoic acid (RA) inhibition-related adverse outcome pathway (AOP). Inhibition of retinaldehyde dehydrogenase (RALDH) by known or unknown inhibitors potentially induces decreased cytosolic retinoic acid (RA) levels, which in turn, changes target gene expression, leads to abnormal embryonic eye development at individual level, and finally results in population decline of teleosts.
Many previous studies have reported that RA signaling plays a vital role in vertebrate eye development
[23][24][35]. RA synthesis is mediated by three types of RALDHs, including RALDH1, RALDH2, and RALDH3. These enzyme-coding genes contribute to RA synthesis in non-overlapping locations during eye development
[35]. For instance, when the optic cup forms, the RALDH2-coding gene is first expressed in the perioptic mesenchyme. Subsequently, RALDH1- and RALDH3-coding genes are expressed in the dorsal and ventral retina, respectively
[35]. The synthesized RA then participates in eye development to form the optic cup, ventral retina, cornea, and eyelids
[35]. Mouse knockout model-mediated genetic studies demonstrated the role of RA in embryonic eye development
[35][42]. Raldh1-knockout mice had previously presented no noticeable adverse outcome and only showed slight RA activity in the dorsal retina owing to the compensation of Raldh3 during eye development
[35][42]. However, Raldh1 and Raldh3 double knock out mutants induced overgrowth of mesenchyme in the cornea and eyelids due to defective apoptosis
[35][43][44]. In another study, the Raldh3 knock out embryo exhibited eye and nasal defects, and thus could not survive due to blockage of the nasal passages
[45]. Raldh3 knock out mutant showed abnormal embryonic development by shortening of the ventral retina. Furthermore, the study of Raldh2 knock out mutant and Raldh1/Raldh2 double knock out mutants showed the stoppage of optic cup development, which was subsequently rescued by RA addition
[46]. These findings suggested that RALDH is an important molecule for embryonic eye development. A previous study had shown that citral competitively inhibits ventral RALDH of zebrafish embryo, thereby leading to reduced RA synthesis
[25]. Citral administration under neurula-stage of the zebrafish embryo led to the deformity of a ventral retina in zebrafish eyes. Conversely, exogenous addition of RA induced a duplication of the zebrafish retina in a concentration-dependent manner during the initial formation of the optic primordia
[24][47]. The results together indicated that the RALDH inhibition-associated undermined RA synthesis potentially causes abnormal eye development in chordates, including teleosts.
The potential AO can be sequentially explained from the molecular level to population level via the AOP framework. As shown in
Figure 2, we schematized the potential AO by RALDH inhibition. At both cellular and molecular levels, RALDH inhibitors decreased the conversion of retinal to RA, thereby inhibiting the activation of target gene transcription. At the organ level, inhibited RA synthesis led to abnormal eye development, caused by inhibited optical elements. Finally, the abnormal eye development led to visual impairment at the individual level. Previous studies had reported that ocular coloboma, caused by incomplete closure of the choroid fissure, was potentially related to the ventral RA levels due to several genes; for example,
Lrp6 (a bottleneck coreceptor in the canonical Wnt signaling pathway) knock out mutation resulted in ocular coloboma in mice
[35][48]. The Lrp6 null mutant showed a dramatically inhibited level of RALDH in mouse eye
[48]. The visual impairment potentially affected reproduction and survival of teleosts, with lowered fecundity and hence decline in the population level (
Figure 2). However, the population decline caused by RALDH inhibition-mediated abnormal eye development still remains poorly understood. Therefore, the weak weight of evidence needs to be redeemed to completely construct the AOP framework.
3. Conclusions
RALDH is a key enzymatic component in cellular RA synthesis from retinaldehyde. The synthesized RA systematically regulates gene transcription via conversion of RAR or RXR receptors, thereby conducting morphogenesis and other functions during the embryonic development. In the current review, we comprehensively presented the importance of RA-mediated eye development, and potential AO derived from RALDH inhibition alongside the AOP (AOP297) framework, based on the existing knowledge.
RALDH-relevant gene deletion and RALDH-inhibitor administration caused abnormal eye development, and could potentially lead to population decline. Despite the weak weight of evidence regarding the relationship between RA inhibition and population decline, the AOP suggested the need for regulation of RALDH inhibitors to prevent potential risk of reproductive toxicity. However, further studies are required to support WoEs to construct a more complete AOP.