This work proposes an open-hardware low-cost battery maintenance tool architecture that can be used with common laboratory instruments. The tool is based on a relay matrix and a battery monitor integrated circuit. It is able to completely characterize and optimize the state of a battery independently of the battery management system and also gives a figure of the individual aging of the battery cells. The work shows the architecture and the experimental validation of a 16-cells battery maintenance tool prototype. The results demonstrate that utilizing the tool brings the battery to the best possible state and identifies the degradation of the cells in terms of capacity and resistance.
1. Introduction
The vehicle electrification process is becoming a reality. Li-ion batteries are the leading actors of this change, thanks to their high energy and power density. The market of electric mobility is showing a huge increase in recent years. The number of Light-Electric-Vehicles (LEVs), such as e-scooters, e-bikes, and e-motorcycles, will show a large increment as described in the study presented in [
1]. Thus, the increase of the energy demand, the lack of widely spread charging infrastructures, the battery diagnostic and maintenance services, and the scarcity of assistance centers dedicated to e-mobility are issues that need to be addressed. Generally, the lithium batteries are provided with a Battery Management System (BMS) that manages the battery and controls its state and safety. Since the battery pack cost might reach up to 45% of the entire LEV cost [
2], the battery manufacturers tend to minimize the BMS hardware and software complexity as much as possible by implementing only the basic features that guarantee the system safety. Therefore, advantageous functionalities such as the balancing system and sophisticated algorithms to optimize the battery state and LEV performance might be very reduced or even not implemented. However, the manufacturing process can lead to electrical characteristics that differ from cell to cell. Furthermore, the cells are subjected to different temperatures that accelerate asymmetrical degradation with aging. The battery may lose performance if these aspects are not addressed by the BMS. For example, the usable battery capacity decreases if the BMS does not implement a charge equalization or balancing system. In this scenario, maintenance centers are required to check and keep the LEV battery to an optimal state extending its lifespan. The diagnostic tests should include both the check of the BMS functionalities and the characterization of the lithium-ion cells that compose the battery. Instead, the development of low-cost instrumentation to diagnose the aging of the lithium-ion cells is still lacking. Specifically, these instruments should be able to identify faulty or weak cells and suggest the substitution of the damaged cells or the entire battery. Nowadays, the LEV maintenance services are still limited to the producer web-page or to call centers, and the faulty objects are usually sent to the manufacturer or its partner workshops. This maintenance procedure may require a long time and determines long stops of the vehicle. Hence, assistance centers independent of the e-mobility device producers and spread into the cities could simplify the process and improve its timing response. They would support the mobility electrification process. However, the cost of professional battery characterization instruments that can simultaneously test all the battery cells still limits the assistance centers escalation. Scientific works present low-cost single Li-ion cell characterization instruments composed of commercial laboratory instruments and a personal computer to reduce the maintenance system costs [
4]. However, they can characterize only one cell at a time, so the diagnostic process may be very long and requires continuous manual operations to connect each cell to the tool. A maintenance tool that uses low-cost instrumentation was introduced in [
5]. The basic idea to speed up and automatize the characterization procedure of an LEV battery pack is only described in that work. This work aims to extend the idea reported in [
5] by developing a real prototype of the tool and experimentally verifying its diagnostic capabilities.
2. Maintenance Tool
Before describing in details the maintenance tool proposed in this work, it is worth saying that we suppose that the battery is provided with a standard connector to simplify the access to the cell terminals. In this way, a maintenance service operator can verify the battery state and optimize it independently of the BMS integrated in the pack. This assumption is not difficult to satisfy, because any BMS has electrical connections to each cell, at least to measure the cell voltages for safety reasons. Figure 1 shows the general architecture of the low-cost maintenance system tool for a battery with N series-connected cells. It consists of some blocks, among which are the control and measurement unit, the diverter relay-matrix and the management unit.

Figure 1. General architecture of the proposed maintenance tool for a battery composed of N series-connected cells.
The diverter relay-matrix is composed of diverter relays organized in a matrix that allows us to select one specific cell
CX among the
N battery cells. The block has
N differential inputs, one for each cell, and one differential output.
The choice of a relay-matrix instead of a MOS-based one prevents unwanted cell short-circuits due to programming mistakes or device faults. In fact, the mechanical switch of the relay will never contact the two diverter terminals simultaneously. Two other relay groups are used to connect the battery and the selected cell CX to the external lab instruments. The charging and discharging paths in both groups are split. In this way, standalone low-cost laboratory instruments with different power capabilities can easily be used to separately charge/discharge the battery and the selected cell.
Figure 2. (a) Photograph of the tool relay-matrix board; (b) Relay-matrix architecture for a battery composed of 16 series-connected cells.
3. Maintenance Procedure
A battery composed of 16 series-connected second-hand cells was assembled and characterized by using the proposed maintenance tool to verify its functionalities. The cells are LGDBHE21865A from LG Chem. They are cylindrical with a nominal capacity of 2.5 Ah, a voltage range from 2.75 V to 4.2 V and a rated series resistance lower than 20 mΩ. These cells were used in the first life in laboratory investigations characterized by different electrical and environmental conditions. Furthermore, some of them were also abused in some cases. Their different history and aging level make them a perfect example for testing the maintenance tool, and in particular to verify if the tool is able to reveal the most degraded cells in the pack. It is worth reminding that the battery is not equipped with a BMS, because it was assembled and exclusively used for the validation of the maintenance tool. Figure 3a shows the block scheme of the experimental setup used to carry-out the battery maintenance tests. It consists of the maintenance system, the assembled battery, two Tektronix Keihtley 2420 used as cell charger and load, respectively, a RIGOL DL3031A as battery load, and a TTi QL355TP power supply used as battery charger, as the photograph in Figure 3b shows. For the sake of clarity, the two 35 V output channels of the QL355TP were series-connected to reach the maximum battery voltage of 67.2 V.
Figure 3. Experimental set-up used to test a battery pack with 16 series-connected cells. (a) Block scheme; (b) Prototype photograph.
Specifically, Figure 4 shows the flowchart of the maintenance procedure adopted, which starts with the battery charging and equalization step. To this end, the tool charges the battery by connecting the power supply set with a constant current of 1.25 A. The charging process continues until one cell reaches the upper cut-off voltage of 4.2 V. Then, the battery charger is disconnected and the cell C0 is selected by the relay-matrix. C0 is fully charged by connecting to it the Keihtley 2420, which applies the classical Constant Current-Constant Voltage (CC-CV) profile with values of 1.25 A and 4.2 V, respectively. The CC-CV profile continues until the cell charging current falls below 125 mA. Then, the maintenance tool disconnects the cell C0 and connects the cell charger to the cell C1. The procedure is repeated for all the cells of the battery pack. Every battery cell is fully charged and balanced with the others at the end of the initialization procedure.
Figure 4. Flowchart of the battery maintenance procedure.
The battery characterization proceeds by using a procedure similar to a Pulsed Current Test (PCT) [
6]. Specifically, the resistance is identified by evaluating the voltage drop due to the current step. The amplitude and duration of the current pulse were set to 1.25 A and 360 s, respectively. The current pulses are directly applied to the battery terminals by the electronic load, while the stack monitor simultaneously acquires the voltage of each cell during the test. The PCT continues until one cell reaches the minimum cut-off voltage value of 2.75 V. Since the PCT starts from the fully charged condition of each cell and ends when the cell with the lowest capacity reaches the fully discharged state, the time integration of the current gives the actual residual capacity of the battery, which is the same of the most aged cell. Finally, the cells are individually discharged, one by one, to identify their residual capacity. Here, the relay-matrix sequentially selects and connects one cell to the cell load. This final discharging phase occurs with a constant current of 1.25 A and 2.75 V as cell cut-off voltage. The actual cell capacity is assessed by integrating the cell current and adding the measured charge to the residual capacity of the most degraded cell. The outstanding result obtained with the maintenance tool is the complete characterization of every cell of the battery in terms of the residual capacity and the internal resistance at different values state of charge.
4. Tool Validation
The battery characterization procedure described above was applied to the assembled battery. Figure 5 shows the cell voltages together with the currents of the battery and the selected cell, as measured by the stack monitor IC and the calibrated sensors. We note that the battery is first charged with a constant current of 1.25 A (orange track) in Figure 5b. The charge continues until C11 reaches the upper cut-off voltage of 4.2 V [see Figure 5a]. Then, the maintenance tool disconnects the battery charger and connects the cell charger to C0. The charger applies the CC-CV profile to fully charge the cell (blue track) in Figure 5b. As soon as the cell current reaches the stop value of 125 mA, the maintenance tool disconnects C0 and connects C1 to the charger. The procedure is repeated until all the cells are fully charged in sequence. It should be noted that the cells, initially strongly unbalanced, are all progressively brought to the maximum voltage achieving the complete cell balancing at 100% state of charge. Indeed, the maximum mismatch among the cell voltages is reduced from 247 mV to 35 mV at the end of the balancing phase, as is visible in Figure 5a.
Figure 5. Cell voltages. (a) battery and cell currents; (b) during the charge balancing phase. The cells are sequentially charged up to the maximum voltage level.
Starting from the battery fully charged and balanced, the resistance and capacity identification is carried-out by exploiting the pulsed current test.
Figure 6 reports the battery current, the cell voltages, and the series-resistances in the diagrams from top to the bottom, respectively. It should be noted that
C6 is the most aged cell as it reaches the minimum cut-off voltage before the others during the ninth pulse. At this point, the maintenance tool stops the PCT to avoid undervoltage on this cell. The time integration of the current allows us to calculate the
C6 residual capacity that results to be 1.14 Ah, a value below 50% of the nominal capacity. As expected,
C6 also shows the highest series-resistance, as seen in
Figure 6c. Thus, the results of the maintenance procedure confirm the capability of the proposed tool to determine the most aged cell, which limits both the maximum battery usable capacity and the power performance.
Figure 7 shows the residual capacity of the cells and their series-resistance calculated as the average of the values shown in
Figure 6. The cells with the lowest residual capacities show the highest series-resistance.
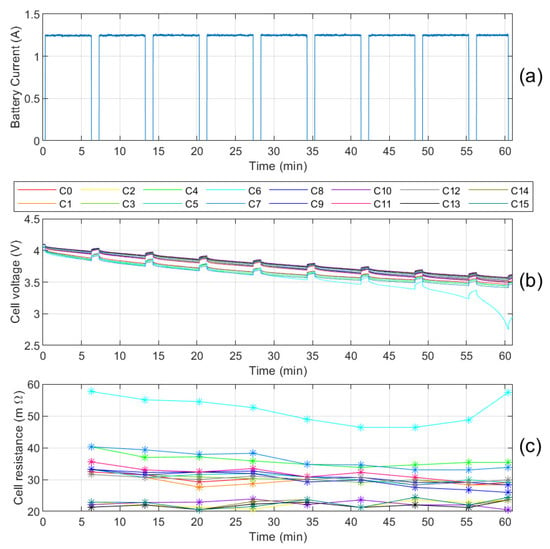
Figure 6. Cell voltages. (a), battery current; (b), and cell series-resistances; (c) measured during the PCT.
Figure 7. Average series-resistance value and residual capacity of each battery cell.
5. Conclusion
The idea of a low-cost battery maintenance tool conceptually described in [
5] has found a practical realization in this work. Specifically, the experimental results validate the proposed tool for a battery composed of 16 series-connected lithium-ion cells. In fact, the tool is able to balance the battery pack restoring the optimal condition. This fact will increase the LEV performance without interactions with the BMS that usually is a closed system. Moreover, the tool can completely characterize the battery pack by measuring the actual residual capacity and the series-resistance of each cell. These important features allow a maintenance operator to map the battery state and to identify the most degraded and aged cells that limit the overall battery performance in terms of usable capacity and power capability.
The maintenance tool can selectively charge and discharge either the battery or a specific cell. Thus, it can bring the battery in a desired state and simultaneously apply to all the cells a given current profile. Otherwise, it can separately operate on each battery cell by properly setting the relay-matrix. These capabilities combined with the possibility of simultaneously acquiring all the cell voltages speed-up the battery maintenance procedure if compared to the instruments proposed in [
4].
Moreover, flexibility is a strength of the proposed tool. It can employ different low-cost laboratory instruments with characteristics suited to the battery under test, and it can easily be managed by a simple PC as shown in
Figure 3. All these features make the proposed maintenance tool appealing in terms of overall cost, particularly if compared with professional characterization tools. Therefore, it simplifies the maintenance procedure supporting and accelerating the LEVdiffusion. Finally, we also remind that the tool hardware and software source files are available as supplementary material and can be downloaded from the link
https://github.com/batterylabunipi/MaintenanceToolForLightElectricVehicles.git (accessed on 6 August 2021), according to the open-source philosophy [
6].
References
- Sachs, C.; Burandt, S.; Mandelj, S.; Mutter, R. Assessing the market of light electric vehicles as a potential application for electric in-wheel drives. In Proceedings of the 2016 6th International Electric Drives Production Conference (EDPC), Nuremberg, Germany, 30 November–1 December 2016. [Google Scholar] [CrossRef]
- Carloni, A.; Baronti, F.; Di Rienzo, R.; Roncella, R.; Saletti, R. Open and Flexible Li-ion Battery Tester Based on Python Language and Raspberry Pi. Electronics 2018, 7, 454. [Google Scholar] [CrossRef]
- Babin, A.; Rizoug, N.; Mesbahi, T.; Boscher, D.; Hamdoun, Z.; Larouci, C. Total Cost of Ownership Improvement of Commercial Electric Vehicles Using Battery Sizing and Intelligent Charge Method. IEEE Trans. Ind. Appl. 2018, 54, 1691–1700. [Google Scholar] [CrossRef]
- Vergori, E.; Mocera, F.; Somà, A. Battery Modelling and Simulation Using a Programmable Testing Equipment. Computers 2018, 7, 20. [Google Scholar] [CrossRef]
- Carloni, A.; Baronti, F.; Di Rienzo, R.; Roncella, R.; Saletti, R. Preliminary Study of a Novel Lithium-Ion Low-Cost Battery Maintenance system. In Lecture Notes in Electrical Engineering; Springer International Publishing: Basel, Switzerland, 2021; pp. 241–245. [Google Scholar] [CrossRef]
- Lee, S.; Kim, J. Power Capability Analysis of Lithium Battery and Supercapacitor by Pulse Duration. Electronics 2019, 8, 1395. [Google Scholar] [CrossRef]