Banana is a tropical fruit grown in more than 130 countries. It is the second most produced fruit after citrus, contributing around 16% of world fruit production and the fourth most important food crop after rice, wheat and corn. Banana is very nutritious and digests better than many other fruits. After harvest, almost 60% of banana biomass is left as waste. Worldwide, about 114.08 million metric tons of banana waste-loss are produced, leading to environmental problems such as the excessive emission of greenhouse gases. These wastes contain a high content of paramount industrial importance, such as cellulose, hemicellulose and natural fibers that various processes can modify, such as bacterial fermentation and anaerobic degradation, to obtain bioplastics, organic fertilizers and biofuels such as ethanol, biogas, hydrogen and biodiesel. In addition, they can be used in wastewater treatment methods by producing low-cost biofilters and obtaining activated carbon from rachis and banana peel. Furthermore, nanometric fibers commonly used in nanotechnology applications and silver nanoparticles useful in therapeutic cancer treatments, can be produced from banana pseudostems.
1. Introduction
Banana is a large-scale, evergreen herbaceous plant belonging to the Zingiberales order, Musaceae family, and Musa genus, with high calcium, magnesium, and high assimilation of nitrogen emissions
[1]. The banana is a fruit with variable qualities, based on size, color, and firmness, generally curved and fleshy, covered with green skin, yellow after maturation, and brown when ripe. The fruit grows in cones from the plant’s top, up to 15 m, between 80 to 180 days
[2].
In general, bananas have more than 50 species and dozens of hybrids, large and rhizomatous underground stems, from which their large leaves with powerfully spirally arranged pods start, giving the shape of a false stem (pseudostem, Figure 1).
It belongs to the Musaceae family, order Scitamineae. This family is made up of the
Musa and
Ensete gender, monocotyledonous plants, of intraspecific and interspecific crosses between
Musa acuminata Colla (genome A) and
Musa balbisiana Colla (genome B)
[3]. Currently, banana classification is based on various morphological variations that help in the differentiation of landrace varieties of dessert bananas (AA, AAA, AAB), cooking bananas (AAA, AAB, ABB)
[4]. To illustrate this classification more clearly,
Table 1 groups together varieties of bananas such as Cavendish or Gros Michel, which usually are produced in countries with a good production index, such as India, the Philippines, and Ecuador.
Figure 1. Parts of the banana plant. Adapted from [5].
Table 1. Classification of banana varieties based on morphological differences.
Producing Country |
Variety |
Genotype |
Subgroup |
References |
Ecuador |
Williams |
AAA |
Gros Michel |
[6][7] |
Dwarf Cavendish |
AAA |
Cavendish |
Maqueño |
AAB |
Red |
Gros Michel Highgate |
AAA |
Gros Michel |
Philippines |
Saba |
ABB |
Saba |
[8][9] |
Cavendish |
AAA |
Cavendish |
Lakatan |
AAA |
Lakatan |
Colombia |
Banana Valery |
AAA |
Cavendish |
[10] |
Hartón Enano |
AAB |
Plantain |
Bocadillo del Quindío |
AA |
Sucrier |
Guineo Común |
AAAae |
Lujujira |
India |
Robusta |
AAA |
Cavendish |
[11][12] |
Palayankodan |
AAB |
Mysore |
Nendran |
AAB |
Cavendish |
Red banana |
AAA |
Red |
Perú |
Williams |
AAA |
Cavendish |
[13] |
FHIA-17 |
AAAA |
Non-defined |
Cavendish Valery |
AAA |
Cavendish |
Chemical Composition of Banana
Banana contains sugars, acids, vitamin C, amino acids, and pectin that form the fruit’s soluble solids content and vary according to the variety grown and the degree of maturity
[14]. During the ripening process, ethylene production is induced, which stimulates the production of the enzyme amylase, and breaks down starch into sugar. At the same time, it initiates the synthesis of the enzyme pectinase to act on the pectin between the cells of the pulp, allowing the softening of the plant tissues
[15].
The fruit contains various chemical components (
Table 2), especially dietary fiber and sugars. During the early part of ripening, sucrose is the predominant sugar, but in the later stages, glucose and fructose predominate
[16]. The conversion of starch to sucrose is catalyzed by sucrose phosphate synthase activity, while hydrolysis causes the conversion of starch to non-reducing sugars from sucrose
[16]. Bananas are considered a good source of potassium and magnesium, essential components in nutrition, and their functional roles involve structural, physiological, and metabolic processes in the body
[17]. Bananas can provide macro and micro minerals in the diet such as P, K, Ca, Mg, Na, which, together with the carotenoids in the plant, play an essential role in the functioning and nutritional value
[18].
The peel and pulp are good sources of certain biogenic amines (catecholamines) produced by decarboxylation of amino acids or amination of aldehydes and ketones. Catecholamines include dopamine, serotonin, epinephrine, and norepinephrine
[19]. Serotonin is crucial for the correct functioning of the nervous system and control of blood pressure in humans. On the other hand, putrescine contributes to physiological processes such as flowering, fruit development, and cell division. Putrescine is also used as a quality indicator in various foods
[20]. In addition, bananas contain phenolic compounds that help prevent many human disorders such as cardiovascular diseases, obesity, and diabetes
[21].
Table 2. Chemical composition of Banana fruit.
Compound |
Content |
Unit |
References |
Carbohydrates |
22–88 |
g/100g DW |
[15][22][23] |
Dietary fiber |
2–5 |
g/100g DW |
[17][23][24] |
Protein |
1–2 |
g/100g DW |
[15][25][26] |
Grease |
0.3–1.78 |
g/100g DW |
[24][26][27] |
AC |
5 |
mg/100g FW |
[17][23][25] |
P |
350–485 |
mg/100g FW |
[17][18][23] |
Mg |
26–27 |
mg/100g FW |
[15][17][23] |
Vitamin C |
12.7 |
mg/100g FW |
[15][23][25] |
Vitamin A |
12.4 |
mg/100g *RAE |
[23][25] |
Folate |
20 |
µg/100g FW |
[15][23][25] |
Cellulose |
5.47 |
g/100g DW |
[24][26] |
Hemicellulose |
18.83 |
g/100g DW |
[16][26] |
Serotonin |
28 |
µg/g DW |
[28][18] |
Phenols |
1–8 |
g/100g DW |
[18][22][26] |
Dopamine |
7.9–9.9 |
µg/g DW |
[28][29] |
Putrescine |
25–50 |
mg/kg DW |
[20][30] |
Norepinephrine |
1.9 |
µg/g DW |
[28] |
Bananas, like most fruits, are acidic, with a pulp pH below 4.5. The primary organic acids in bananas are ascorbic, citric, malic, and oxalic acid, industrially essential acids because they mainly act as antimicrobial agents, neutralizers, and preservatives for food
[23].
2. Use of Banana Waste-Loss
In recent decades, the world population increased up to 7 billion, generating around 683 million tons of agri-food waste, 34% related to waste-loss of food produced worldwide
[31].
The use of this waste loss has become a topic of great interest, leading to the transition and implementation of a circular economy model as a regenerative economic model
[32]. The objective is to close the life cycle of the products through an increase and optimization of use. This objective is also seen in overall favorable balances for the environment and the economy
[33].
Sustainable development of the circular economy concept is only possible if technologies are adopted to recover waste losses. The recovery of food waste loss opens up new economic growth horizons, allowing the opportunity to transform raw material into a circular loop
[34]. The banana industry produces a high amount of lignocellulosic waste that can be used in different recovery processes such as biofuels, wastewater treatment, bioplastics, organic fertilizer, and nanotechnology applications. The variety of bananas most used in waste-loss is the
Cavendish and
Gros Michel varieties due to their high availability in different countries such as India, the Philippines, and Ecuador. In addition, it should be noted that the most used residues are the peel and pseudostem from bananas due to their high content of cellulose, hemicellulose, and fiber.
Musa paradisiaca spp. is one of the most demanded varieties in the harvesting industry. Thanks to the composition of its residues, it can be used in all the applications mentioned above. Banana
Cavendish is widely used in biofuels because sugars represent the species’ main component of soluble solids. By subjecting them to cellulolytic organisms, a greater quantity of fermented sugars is necessary for biofuel production. Furthermore, the varieties
Williams,
Musa balbisiana, and
Musa accuminata have good potential for use in wastewater treatment, bioplastics and nanotechnology applications thanks to their physicochemical properties, such as its lignocellulosic content, its density, and its ability to adapt to extreme conditions of climate, soil, and water.
2.1. Biofuel Production
Due to the rapid development of the automotive industry and environmental pollution problems, the growing demand for petroleum-derived fuel has inspired efforts to explore alternative fuels
[35].
The lignocellulosic biomass from the shell, pseudostems, and rachis of banana represents a source of promising raw material for ethanol production due to its abundance and high availability. Agri-food waste can be used locally and does not trigger competition between fuel and food
[36]. The production of bioethanol from lignocellulosic biomass (
Figure 2) comprises four main stages: the pretreatment of the raw material, the enzymatic saccharification, the fermentation, and the recovery of the product—the final crucial step for the process to be economically viable on a commercial scale due to high energy consumption
[37].
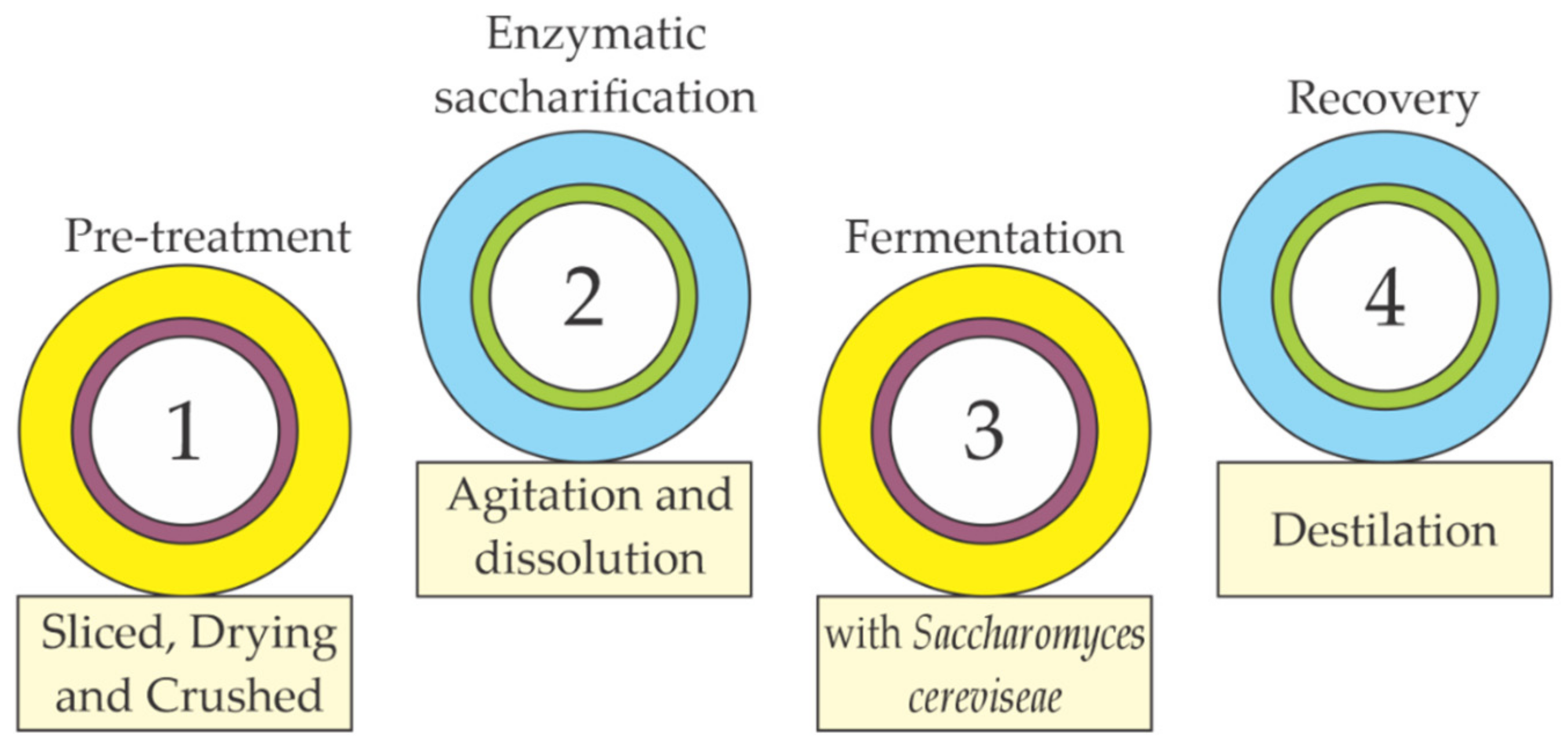
Figure 2. Stages of the bioethanol production process. Adapted from
[37].
The banana rachis is chopped to reduce their size to 1 cm in width in the pretreatment, dried, and later crushed for their correct use in the other processes
[38]. The enzymatic treatment can be carried out in two-liter bioreactors at a concentration of 60% (dry weight) of the shell. The waste loss is dissolved and stirred in purified water, and during this stage, it is vital to measure the pH, the sugar content, and the dissolved oxygen
[39]. Fermentation is the anaerobic degradation of organic substances using catalysts presented by some microscopic organisms, such as yeasts. During this process, glucose is transformed into ethanol and carbon dioxide thanks to fermentative processes of yeasts such as
Saccharomyces cerevisiae [40]. Finally, with the distillation of the alcohol, the aim is to remove the final product due to the difference in boiling point. Water is separated to obtain alcoholic beverages with the highest concentration possible (around 40%
v/
v)
[41].
Finally, with the distillation of the alcohol, the aim is to remove the final product due to the difference in boiling point as much water is separated to obtain alcoholic beverages with the highest concentration possible (around 40%
v/
v)
[41].
2.2. Wastewater Treatment
According to the World Wildlife Organization (WWF), more than 1100 million people do not have access to drinking water worldwide because the growing population demands safe drinking water insurance
[42].
Several organic and inorganic products are considered toxic pollutants frequently released into the environment, mainly in surface waters
[43]. The industrial and agricultural sectors promote chemical contamination of water due to the various metals, colorants, pesticides, drugs, and other often used compounds
[44].
There is a progressive increase in heavy metals such as chromium, mercury, nickel, cadmium, and copper in drinking and wastewater samples that account for the increasing contamination. In the case of hexavalent chromium, it behaves as a toxic element. Chromium is considered harmful for red blood cells, liver, spleen, kidney, soft tissues, and bones, affecting the digestive, respiratory and urinary systems
[45]. Chromium is a potentially toxic and carcinogenic metal originating from natural processes and anthropogenic activities such as the iron steel, electroplating, and leather industries
[46]. For removing heavy metals from the water using biomass, techniques such as adsorption, membrane filtration, or chemical precipitations can be used, which are explained schematically in
Figure 3 [47].
Figure 3. Techniques for the removal of heavy metals present in the water through banana biomass.
2.3. Bioplastics
Polymers can be natural, synthetic, or semi-synthetic (
Figure 4). Naturals include proteins, enzymes, and polysaccharides, while synthetic plastics are thermoplastics, moldable with heating, or thermosets, which are not moldable under heating
[48].
Bioplastics are also produced using other polysaccharides such as polyhydroxyalkanoates, collagen, and lipids from vegetable oils. In this sense, food waste-loss as an initial raw material is a good option for elaborating biodegradable materials
[49]. Starch is a natural polymer of glucose, and it is considered a mixture of two polysaccharides: amylose and amylopectin. Starch constitutes the reserve of plant nutrients, and behind cellulose, it is the most abundant carbohydrate in nature
[50]. Starch is not plastic, but it can become plastic through polymer technology or fermentation using various techniques such as casting, mixing, extrusion, or injection molding. Commercially, 50% of bioplastics are prepared from different starches
[51].
Figure 4. Examples of biodegradable and non-biodegradable natural and synthetic polymers. Adapted from
[52].
2.4. Nanotechnology
Nanotechnology has been a field of vertiginous development in recent years, thanks to the multiple applications in medicine, food, cosmetics, and pharmaceuticals, among other areas
[53]. The main advantage of nanometric organic substances is their higher ingestion-absorption and better bioavailability in the body, compared to ordinary counterparts on a micro or macroscopic scale
[54]. In this sense, lignocellulosic materials are a widely used green alternative to produce materials on a nanometric scale
[55]. The intelligent release of nutrients, the bioseparation of proteins, the rapid sampling of chemical and biological contaminants, the intelligent packaging, and the nanoencapsulation of nutraceuticals are emerging nanotechnology topics in the agri-food industry
[56].
Some uses of
Musaceae waste-losses have been reported, especially cellulose production (
Figure 5), while others in the field of materials such as the production of binder-free boards and carbonaceous preforms
[57].
Figure 5. Cellulose extraction from the banana pseudostem and flower stem.
2.5. Organic Fertilizers
Organic fertilizers can be defined as a product of the natural decomposition of organic matter by fermentation processes, which varies according to the type of fertilizer to be prepared. The degradation occurs naturally through the air, the sun, microorganisms, and water
[58]. Organic fertilizers come from composting organic compounds mediated by microorganisms, improving soil quality and providing nutrients to crops, thus reducing chemical fertilizer use and the undesirable polluting factors production
[59][60]. Composting systems can be classified depending on the level of aeration in the open-air system, semi-open system, and confined system, where the latter is ideal for controlling aeration
[61]. Banana peels are rich in micronutrients, suggesting their use to improve soil quality and crop yields, either through their use as an organic soil conditioner or to produce compost to meet specific plant requirements
[62]. To obtain the cake, the remains of banana leaves and pseudostems are subjected to endogenous hydrolysis, extraction, and neutralization processes. Subsequently, aerobic degradation leads to compost
[63].
Composting is done by the layered pile method (
Figure 6), with an approximate volume of 1 m
3. Banana waste loss is cut and mixed with organic matter for its subsequent degradation process. Water is sprinkled over compost piles to keep the mix moist, with approximately 50% moisture content
[64]. The process takes about twelve weeks when the core temperature of the material is close to the air temperature. During this period, the composting material is mixed at least once a week to avoid excessive temperatures from the beginning of the process
[65]. The application of this type of fertilizer improves the structure of the soils allowing better absorption of nutrients such as N, P, and K by the plants
[66]. Compost eliminates harmful bacteria and fungi, neutralizes pH, improves soil texture, and improves water retention. Soils with applied compost adsorb better nutrients that benefit plants, thus creating healthier and nutrient-rich foods
[67].
Figure 6. The layering of the compost pile.
3. Conclusions
The banana industry generates a large amount of waste loss that can be used in various recovery processes, such as biofuels, wastewater treatment, and the production of bioplastics focused on implementing a circular economy. Due to its high content of organic compounds rich in carbon such as polysaccharides, the banana peel has been used mainly to obtain bioplastics with high degradation rates and produce biofuels such as diesel and ethanol. The leaves from the banana plant are used to produce biodegradable packaging and utensils, and organic fertilizers. Due to their fibrous composition, the banana’s pseudostems are used to obtain bioplastics requiring little time for biodegradation. Cellulose and hemicellulose banana waste-loss content is of great importance in nanotechnological processes, especially in producing green nanoparticles. It has been shown that the leaves, rachis, pseudostems, and banana peel have a high potential for use, which can be reused in various recovery processes. In addition, it allows to close production cycles and reduce the accumulation of waste from the banana industry through its use in different applications, contributing to the growth of the circular economy.
Despite the wide range of application possibilities for banana industry waste, production techniques must be improved to obtain products with better characteristics, such as improving the biofuel process’s pre-treatment technique to produce more energy-efficient end products. However, the lack of pilot-scale studies and the industrial implementation of these wastes is evident, suggesting the need for more studies at higher-scale applications. We cannot forget that companies seek to innovate their productive activities to obtain products with added value every day. For that reason, it is necessary to implement the various possibilities to add value to the banana industry in a sustainable way, contributing to a circular economy.