The genetic mutation of SARS-CoV-2 -especially RBD- might be linked to the viral properties that influence the viral transmission mode and severity of COVID-19 as well as RBD conformation. One of the dominant variants during COVID-19 pandemic has been the D614G mutation (not in the RBD region) of S glycoprotein; several reports have claimed that this mutation is able to increase the infectivity and stability of SARS-CoV-2. Up until now, most neutralizing antibodies against SARS-CoV-2 have been targeting its RBD. However, there have been several mutations reported in SARS-CoV-2 RBD, such as N501Y, L452R, S477N, E484K, A502S, N439K, S494P, T478K, K417N, and K417T. These mutations pose a threat due to their role in host cell entry via the hACE2 receptor, which might strengthen SARS-CoV-2 infectivity, conformation and stability of RBD, viral load, or resistance against neutralizing antibodies.
1. Overview
The receptor-binding domain (RBD) of severe acute respiratory syndrome coronavirus 2 (SARS-CoV-2) mediates the viral–host interaction and is a target for most neutralizing antibodies. Nevertheless, SARS-CoV-2 RBD mutations pose a threat due to their role in host cell entry via the human angiotensin-converting enzyme 2 receptor that might strengthen SARS-CoV-2 infectivity, viral load, or resistance against neutralizing antibodies. To understand the molecular structural link between RBD mutations and infectivity, the top five mutant RBDs (i.e., N501Y, E484K L452R, S477N, and N439K) were selected based on their recorded case numbers. These mutants along with wild-type (WT) RBD were studied through all-atom molecular dynamics (MD) simulations of 100 ns. The principal component analysis and the free energy landscape were used too. Interestingly, N501Y, N439K, and E484K mutations were observed to increase the rigidity in some RBD regions while increasing the flexibility of the receptor-binding motif (RBM) region, suggesting a compensation of the entropy penalty. However, S477N and L452R RBDs were observed to increase the flexibility of the RBM region while maintaining similar flexibility in other RBD regions in comparison to WT RBD. Therefore, both mutations (especially S477N) might destabilize the RBD structure, as loose conformation compactness was observed. The destabilizing effect of S477N RBD was consistent with previous work on S477N mutation. Finally, the free energy landscape results showed that mutations changed WT RBD conformation while local minima were maintained for all mutant RBDs. In conclusion, RBD mutations definitely impact the WT RBD structure and conformation as well as increase the binding affinity to angiotensin-converting enzyme receptor.
2. Genetic Mutation of SARS-CoV-2
The pandemic of coronavirus disease 2019 (COVID-19) has infected over 173 million individuals (at the time of this writing) and caused millions of deaths around the globe
[1]. Severe acute respiratory syndrome coronavirus 2 (SARS-CoV-2), responsible for COIVD-19, is a single-stranded positive-strand RNA virus that belongs to the Coronaviridae family
[2]. Coronaviruses (CoVs) were previously known to be present in the environment and to infect humans, although the earlier infections resulted in mild symptoms and were limited to local areas. However, deadly human CoVs, such as SARS-CoV and Middle East respiratory syndrome coronavirus (MERS-CoV) as well as SARS-CoV-2, have appeared in the past two decades. These CoVs are more severe and cover more ground in every passing phase, as they can cause deadly pneumonia in humans along with other gastrointestinal diseases
[3][4][5]. SARS-CoV-2 is characterized by efficient transmission and its ability to rapidly spread worldwide despite its lower mortality rate (3.3%) in comparison to SARS-CoV (10%) and MERS-CoV (37%)
[6].
Structural components of SARS-CoV-2 have been extensively studied
[7][8][9][10][11]. On mature virus, the spike (S) glycoprotein on the surface of SARS-CoV-2 is composed of an extracellular domain (EC), transmembrane (anchor) domain, and short intracellular tail domain (IC)
[11][12]. EC domain has two functional subunits: a receptor-binding subunit (S1) and a membrane-fusion (S2) subunit
[13]. The host cell (cellular) proteases cleave S protein at the boundary between S1–S2 site and S2′ site during host–virus membrane fusion
[11][14]. Further, the S1 subunit compromises the receptor-binding domain (RBD) that is essential for receptor binding and contributes to stabilizing the S2 subunit that harbors the fusion machinery. After the S1 subunit binds to the cellular receptor, subsequent structural rearrangements of metastable S glycoprotein occur to allow fusion between the viral and the host cell membranes. The structural rearrangements can be explained by the conformational dynamics behavior of the S glycoprotein trimer that eventually results in an open (standing) conformation in order to successfully achieve binding and fusion events
[7]. In fact, S glycoprotein is a target for immune cells that neutralize the virus, as many vaccines have been developed based on the antigenicity of S glycoprotein. SARS-CoV-2 RBD is known to bind to human angiotensin converting enzyme 2 (AEC2), specifically through the receptor-binding motif (RMB) of RBD, to mediate the viral–host interaction. Moreover, the RBM bears a flexible nature and contains most of the SARS-CoV-2 RBD residues that bind directly to ACE2 receptor
[10]. However, RBD must adopt a specific conformation (up conformation) to bind efficiently to ACE2
[15].
Importantly, the genetic mutation of SARS-CoV-2 might be linked to the viral properties that influence the viral transmission mode and severity of COVID-19 as well as RBD conformation
[15][16]. One of the dominant variants during COVID-19 pandemic has been the D614G mutation (not in the RBD region) of S glycoprotein; several reports have claimed that this mutation is able to increase the infectivity and stability of SARS-CoV-2
[17][18][19][20][21]. Up until now, most neutralizing antibodies against SARS-CoV-2 have been targeting its RBD
[22][23][24][25][26]. However, there have been several mutations reported in SARS-CoV-2 RBD, such as N501Y, L452R, S477N, E484K, A502S, N439K, S494P, T478K, K417N, and K417T. These mutations pose a threat due to their role in host cell entry via the hACE2 receptor, which might strengthen SARS-CoV-2 infectivity, conformation and stability of RBD, viral load, or resistance against neutralizing antibodies
[27][28][29][30][31]. For instance, according to a recent report, the N439K variant showed resistance against several neutralizing antibodies, including one authorized by the U.S. Food and Drug Administration (FDA) for emergency use
[32]. It is clear that these mutation sites are mostly located in the RBM in the RBD region of SARS-CoV-2, which has shown a flexible nature. Importantly, the molecular dynamics and flexibility of the RBD region might have contributed to SARS-CoV-2 infectivity
[31][32].
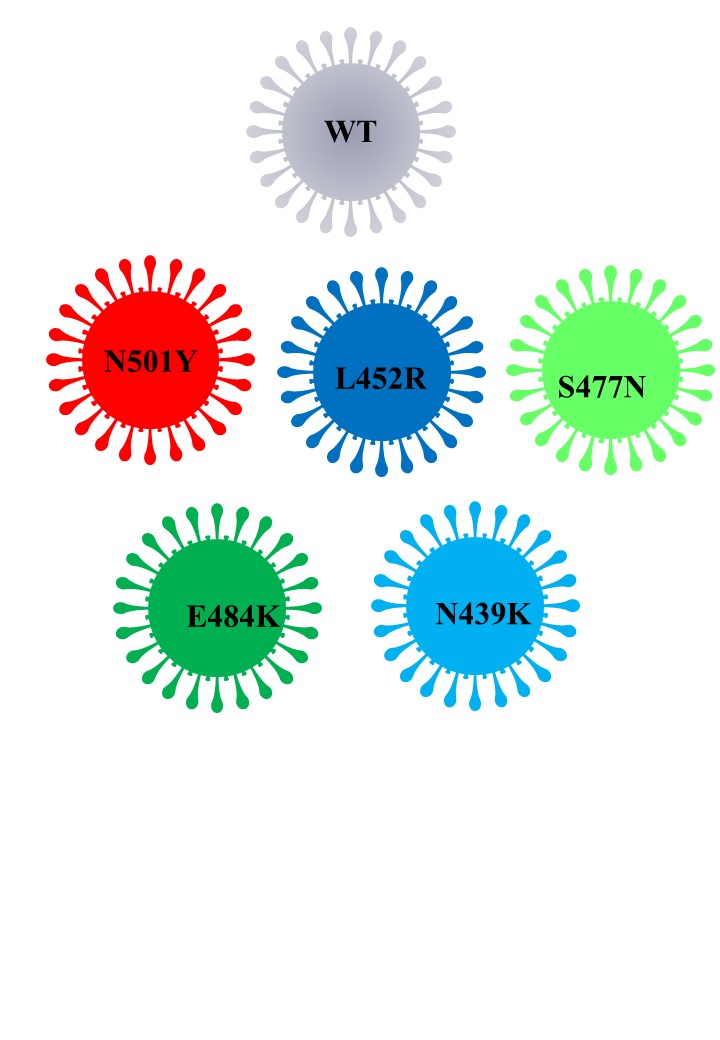
3. Conclusions
Mutations in proteins can affect protein conformation, folding, and stability, and can eventually influence protein–protein interactions and protein thermodynamics
[33]. There are several observed mutations in the RBD of SARS-CoV-2 S glycoprotein that improve its infectivity and strengthen the viral binding interaction to ACE2 receptor
[27]. These mutations compromise the neutralizing ability of anti-SARS-CoV-2 antibodies; therefore, it is necessary to study the mutation effects on the RBD structure (e.g., conformation, stability, dynamics, etc.)
[24][26]. Several structural and dynamic studies at the molecular level show that SARS-CoV-2 RBDs have to adapt open conformation (also known as “up” or “standing”) to effectively bind to ACE2 receptors
[8][16][34][35]. So far, mutations in non-RBD residues, such as the D614G variant, can populate RBD open conformation rather than closed conformations
[36]. Most of the virulent point mutations occur in the RBM loop that directly binds hACE2 and are more prone to conformational variations; thus, these mutations may have the ability to generate a more stable complex with high binding affinity
[37]. For instance, N439K, L452R, T478I, and E484D mutations on RBM have significant free energy changes, and they constitute approximately 58% of all mutations on RBD. Global data analysis shows that infectivity strengthening and virion stable mutations are on the rise (especially the frequency of S477N, N439K, V483A, and V367F), clearly indicating the natural selection of mutations with stronger transmissibility
[27].
Here, we studied five critical mutant RBDs according to the RBD mutation tracking website (CovMT)
[38] by utilizing the all-atom MD simulation technique. The N501Y flexibility in loop Y473–C489 of RBM was comparable to WT RBD; thus, suggesting that tyrosine mutation did not alter neither the loop Y473–C489 flexibility or the whole RBD conformational compactness. Only N501Y RBD showed different loop Y495–Y508 conformations. The mutation of alanine instead of tyrosine at 501 (i.e., N501A) shows an increase in loop Y473–C489 flexibility and conformational compactness according to a related study
[34]. Moreover, the same loop Y473–C489 in SARS-CoV RBD showed a higher flexibility in comparison to SARS-CoV-2 RBD
[34][35]. This suggests that the higher infectivity of the N501Y variant might be attributed to an improvement in the N501Y RBD conformation and therefore a higher affinity to ACE2 receptor. Previously, substitution mutations in the SARS-CoV-2 RBD, N501, L452, N439, E484, T470, and Q498 have been shown to enhance binding affinity for hACE2, thereby increasing infectivity and transmissibility in comparison to the natural SARS-CoV-2
[39].
On the other hand, the loop Y473–C489 flexibility was increased in L452R, N439K, and E484K RBDs, and these mutations were associated with higher infectivity and binding affinity to ACE2. This was consistent with previously reported structural analyses that showed that the RBM region has the highest flexibility
[9][32][40]. However, the observed rigidity in some parts of RBDs (i.e., non-RBM regions) in N439K and E484K RBDs might compensate for the entropy penalty due to flexibility in the loop Y473–C489. Therefore, the N501Y, N439K, and E484K mutations studied in this work have insignificant changes in the overall RBD flexibility. This was indicated by the SARS-CoV-2 mutations may augment the conformational sampling to avoid the entropy cost upon interaction with ACE2 receptor. In contrast, S477N and L452R RBDs showed comparable flexibility to WT RBD in non-RBM regions but higher flexibility in the RBM regions, as well as a loose conformational compactness. Our results were consistent with a pervious study that showed that S477N has a destabilizing effect on RBD structure and therefore less prone to develop disease
PCA, FEL, and porcupine plot results suggested that the destabilizing effect could be noticed in the loop Y473–C489 of S477N RBD as compared to WT RBD. However, the conformational sampling of energetically favorable conformations of mutant RBDs showed local minima, thereby indicating stable structures for these mutant RBDs. The COVDI-19 pandemic presents a continuing threat to global health due to its ongoing critical mutations. To conquer this pandemic, it is necessary to investigate the effects of SARS-CoV-2 mutations at all possible levels, such as structural, functional, and activity levels. Moreover, RBD mutations directly affect the ability of SARS-CoV-2 to binding to ACE2 receptor and therefore affect its infectivity. Our structural investigation of critical mutant RBDs along with WT RBD showed that RBD mutations have a direct impact on the molecular structural of SARS-CoV-2. These data might be helpful for researchers investigating antiviral agents and vaccine research and development against SARS-CoV-2, especially mutant SARS-CoV-2 viruses.