Metformin is the first-line pharmacotherapy for type 2 diabetes mellitus (T2D). Metformin exerts its glucose-lowering effect primarily through decreasing hepatic glucose production (HGP). However, the precise molecular mechanisms of metformin remain unclear due to supra-pharmacological concentration of metformin used in the study.
1. Introduction
The global prevalence of diabetes mellitus has been rising rapidly, and the number of patients with diabetes mellitus has quadrupled during the past 3 decades
[1]. Metformin is currently the first-line drug for the treatment of type 2 diabetes (T2D), primarily owing to its function in reducing HGP
[2][3]. However, the underlying mechanisms of metformin action remain incompletely understood.
Metformin belongs to the biguanide class originally found from the plant
Galega officinalis [4]. Metformin alleviates hyperglycemia in T2D primarily through suppression of HGP
[5] via several mechanisms
[6][7][8]. Metformin, at low concentration, activates AMPK, which triggers CBP-CREB-TORC2 complex dissociation and decreases glucagon-induced cAMP accumulation through cyclic nucleotide phosphodiesterase-4B (PDE4B)
[6][9]. A low-dose metformin treatment also reduces endogenous HGP through inhibiting mitochondrial glycerophosphate dehydrogenase
[10]. Moreover, a high concentration of metformin contributes to a significant decrease in ATP and increase in AMP levels
[11][12]. The increased AMP/ATP ratio enhances AMPK activity and the elevated AMP also inhibits adenylate cyclase, thus reducing cAMP levels and suppressing PKA activity, which blocks glucagon-induced HGP
[13]. Depletion of ATP by metformin also contributes to the suppression of hepatic gluconeogenesis
[11]. AMP also inhibits the activity of fructose −1,6-bisphophatase-1 (FBP1), a rate-controlling enzyme in gluconeogenesis
[14]. Therefore, metformin with different concentrations targets different molecules, whereby it suppresses HGP.
Aspirin, a widely used drug for the treatment of a number of diseases, including fever, pain, rheumatic fever, and inflammatory conditions. Salicylate, an active metabolite of aspirin, is commonly claimed to associate with suppression of protein kinase IκB kinase β (IKKβ) in the NF-κB pathway, reducing inflammation in patients with T2D
[15][16][17][18]. Salicylate activates AMPK and inhibits dephosphorylation of AMPKα-T172 and improves metformin resistance
[17][19], suggesting that salicylate might mitigate the glucagon-controlled glucose metabolism and glucose homeostasis.
Foxo1, a member of the O-class forkhead/winged helix transcriptional factor, is a key regulator in control of gluconeogenesis
[20][21][22]. Recently, we established that glucagon-stimulated PKA promotes Foxo1 phosphorylation at S273, promoting Foxo1 nucleus translocation and enhancing gluconeogenesis
[23]. However, whether metformin-regulated HGP relies on Foxo1 and how metformin regulates Foxo1 remains unknown.
2. Metformin Suppresses Glucagon-Induced HGP through PKA-Foxo1 Pathway in Hepatocytes
To detect whether metformin-mediated HGP suppression requires Foxo1, we treated control and Foxo1 knockout (FKO) primary hepatocytes with different doses of metformin. In control hepatocytes, glucagon significantly increased HGP and 50 μM metformin treatment led to an insignificant reduction in glucagon-induced HGP (Figure 1A). In FKO hepatocytes, glucagon also significantly elevated HGP, whereas 50 μM of metformin had no effect on glucagon-induced HGP (Figure 1B). We then treated hepatocytes with 100 μM of metformin and found that 100 μM metformin treatment significantly abolished glucagon-induced HGP by 27% in control hepatocytes (Figure 1C); however, 100 μM of metformin barely affected glucagon-induced HGP in FKO hepatocytes (Figure 1D). Treatment with 200 μM of metformin significantly reduced glucagon-induced HGP by 34% in control hepatocytes (Figure 1E). In FKO hepatocytes, 200 μM of metformin significantly resulted in a 19% reduction in glucagon-induced HGP (Figure 1F). PKA, a key player in glucagon signaling in control of glucose metabolism, promotes Foxo1 activity via S273 phosphorylation
[23]. We then investigated whether PKA is involved in the metformin-induced HGP suppression. Glucagon significantly increased PKA activity by 24% in control hepatocytes. Treatment with 50 μM of metformin led to an insignificant inhibition on PKA activity upon glucagon challenge; however, 100, 200, and 500 μM metformin treatment significantly suppressed glucagon-induced HGP by 30%, 30%, and 36%, respectively (Figure 1G). Consistently, 100 μM of metformin significantly attenuated glucagon-induced HGP in control hepatocytes, whereas inhibition of PKA by H89 blocked the suppressive effect of metformin on HGP upon glucagon treatment (Figure 1H). Collectively, these results indicate that metformin suppresses glucagon-induced HGP partially through PKA-Foxo1 pathway in hepatocytes.
Figure 1. Metformin suppresses glucagon-induced HGP through the PKA–Foxo1 signaling pathway in primary hepatocytes. (A,B) Primary hepatocytes isolated from control (A) and FKO (B) mice were pretreated with 50 μM of metformin for 30 min and treated with 100 nM of glucagon for 3 h. Glucose content was measured, n = 3 independent experiments/group. (C,D) Primary hepatocytes isolated from control (C) and FKO (D) mice were pretreated with 100 μM of metformin for 30 min and treated with 100 nM of glucagon for 3 h. Glucose content was measured, n = 3 independent experiments/group. (E,F) Primary hepatocytes isolated from control (E) and FKO (F) mice were pretreated with 200 μM of metformin for 30 min and treated with 100 nM of glucagon for 3 h. Glucose content was measured, n = 3 independent experiments/group. (G) Control hepatocytes were pretreated with 50, 100, 200, and 500 μM of metformin for 30 min and treated with 100 nM of glucagon for 1 h. PKA activity was analyzed, n = 3–5 independent experiments/group. (H) Control hepatocytes were pretreated with H89 and metformin, followed by 100 nM glucagon treatment for 3 h. Glucose content was measured, n = 3 independent experiments/group. All are are presented as mean ± SEM. * p < 0.05, ** p < 0.01, *** p < 0.001, NS: no significance, CNTR: control.
3. Metformin Inhibits Blood Glucose and HGP through Foxo1 in Mice
We then examined whether metformin lowers blood glucose and HGP through hepatic Foxo1 in mice. Here, we used control (floxed Foxo1) and hepatic Foxo1 gene knockout (FKO) mice
[22]. Intraperitoneal (i.p.) injections of 100, 150, and 200 mg/kg body weight metformin decreased fasting blood glucose by 15%, 26%, and 25%, respectively, in control mice (Figure 2A,C,E). However, hepatic Foxo1 deficiency diminished the suppressive effect of metformin on fasting blood glucose in FKO mice (Figure 2B,D,F). Consistently, i.p. injection of 150 and 200 mg/kg body weight metformin significantly decreased glucose output upon pyruvate challenge in control mice by 39% and 41%, respectively (Figure 2G,I). However, both 150 and 200 mg/kg body weight metformin administration had no effect on pyruvate-induced glucose output in FKO mice (Figure 2H,J). Thus, these results suggest that hepatic Foxo1 is required for the suppressive effect of metformin on blood glucose and glucose output in mice.
Figure 2. Metformin suppresses blood glucose in mice through hepatic Foxo1 gene. (A,B) 100 mg/kg body weight metformin (Met) was acutely i.p. injected into control (A)and FKO (B) mice. Blood glucose was monitored at indicated time points, n = 5–6 mice/group. (C,D) 150 mg/kg body weight metformin (Met) was acutely i.p. injected into control (C) and FKO (D) mice. Blood glucose was monitored at indicated time points, n = 5–6 mice/group. (E,F) 200 mg/kg body weight metformin (Met) was acutely i.p. injected into control (E) and FKO (F) mice. Blood glucose was monitored at indicated time points, n = 5–6 mice/group. (G,H) 150 mg/kg body weight metformin (Met) was acutely i.p. injected into control (G) and FKO (H) mice after 16 h fasting. After 45 min, pyruvate tolerance test was performed, n = 5–6 mice/group. (I,J) Control (I) and FKO (J) mice were fasted for 16 h and then i.p. injected with 200 mg/kg body weight metformin. After 45 min, pyruvate tolerance test was performed, n = 5 mice/group. All data are presented as mean ± SEM. *** p < 0.001, **** p <0.0001. CNTR: control.
4. Foxo1-S273 Mutations Attenuate the Suppressive Effect of Metformin on HGP in Both Hepatocytes and Mice
Given that PKA increases Foxo1 activity through Foxo1-S273 phosphorylation, as we previously demonstrated
[23], we further evaluated whether Foxo1-S273 phosphorylation plays a key role in metformin-induced HGP suppression. Here, we used Foxo1-S273D or A mutation mouse, where serine (S) was replaced by aspartic acid (D) or alanine (A) to mimic constitutive phosphorylation or dephosphorylation, respectively
[23]. Consistently, in wild type (WT) hepatocytes, 100 μM metformin treatment significantly attenuated glucagon-induced HGP by 16% (Figure 3A); however, in Foxo1
S273D hepatocytes, the suppressive effect of metformin was abolished (Figure 3B). The metformin tolerance test in mice indicated that 150 mg/kg metformin i.p. injection significantly decreased fasting blood glucose by 21% in WT mice, whereas Foxo1-S273D mutation blocked the effect of metformin on reducing blood glucose in mice (Figure 3C). Further analysis of pyruvate-induced glucose production showed that 150 mg/kg metformin injection significantly reduced blood glucose by 28% during pyruvate tolerance test in WT mice; such an effect was blocked in Foxo1-S273D mutant mice (Figure 3D).
Figure 3. Foxo1-S273D mutation blocks metformin-induced HGP and blood glucose suppression in mice. (A,B) Primary hepatocytes isolated from WT (A) and Foxo1S273D (B) mice were pretreated with 100 μM of metformin for 30 min and then treated with 100 nM of glucagon for 3 h. Glucose content was measured, n = 3–4 independent experiments/group. (C) 150 mg/kg body weight metformin (Met) was acutely i.p. injected into 16 h fasting WT and Foxo1S273D mice. Blood glucose was monitored at indicated time points, n = 5–6 mice/group. (D) WT and Foxo1S273D mice were fasted for 16 h and then i.p. injected with 150 mg/kg body weight metformin. After 45 min, pyruvate tolerance test was performed, n = 6–9 mice/group. All data are presented as mean ± SEM. * p < 0.05, ** p < 0.01, NS: no significance.
We then treated mice with 50 mg/kg body weight metformin via daily oral injection for 4 weeks and evaluated glucose homeostasis after a chronic treatment. In control mice, the chronic treatment of metformin significantly reduced fasting blood glucose (Figure 4A) and decreased pyruvate-induced glucose production (Figure 4D). Importantly, in either FKO or Foxo1S273A mice, the chronic treatment of metformin had no effect on reducing fasting blood glucose (Figure 4B,C) and glucose output upon pyruvate challenge (Figure 4E,F). We further detected the effect of metformin on glucose tolerance in mice. In order to reduce variability in baseline blood glucose and insulin levels, we performed overnight (16 h) fasting to mobilize the glucose reservoirs and deplete liver glycogen stores. The control mice with chronic metformin treatment showed a significant improvement in glucose tolerance (Figure 4G); however, both FKO and Foxo1S273A mice had no significant changes in glucose tolerance in response to chronic metformin treatment (Figure 4H,I). Insulin sensitivity was not affected by chronic treatment of metformin in control, FKO, and Foxo1S273A mice (Figure 4J–L). These results indicate that metformin decreases blood glucose and HGP largely through Foxo1 and Foxo1-S273 phosphorylation.
Figure 4. Chronic metformin treatment reduces blood glucose through interference of Foxo1-S273 phosphorylation. (A–C) Blood glucose in WT (A), FKO (B), and Foxo1S273A (C) mice chronically treated with 50 mg/kg body weight metformin after 16 h fasting, n = 5–7 mice/group. (D–F) Pyruvate tolerance test in WT (D), FKO (E), and Foxo1S273A (F) mice chronically treated with 50 mg/kg body weight metformin, n = 5–7 mice/group. (G–I) Glucose tolerance test in WT (G), FKO (H), and Foxo1S273A (I) mice chronically treated with 50 mg/kg body weight metformin, n = 5–7 mice/group. (J–L) Insulin tolerance test in WT (J), FKO (K), and Foxo1S273A (L) mice chronically treated with 50 mg/kg body weight metformin, n = 5–7 mice/group. All data are presented as mean ± SEM. * p < 0.05, ** p < 0.01, *** p < 0.001.
5. Salicylate Inhibits Glucagon-Induced HGP via Foxo1 and Has No Improvement on Metformin-Mediated HGP Suppression
It is known that salicylate treatment improves glucose homeostasis and insulin sensitivity [29-31]. We next examined whether salicylate treatment enhances the beneficial effect of metformin on the regulation of glucose metabolism. We firstly treated the control and FKO hepatocytes with salicylate and found that salicylate significantly abolished glucagon-induced HGP; however, hepatic Foxo1 deficiency blocked the suppressive effect of salicylate on HGP upon glucagon treatment (Figure 5A). Indeed, salicylate treatment markedly decreased glucagon-induced PKA activity (Figure 5B) and inhibition of PKA by H89 diminished the salicylate-mediated HGP suppression in control hepatocytes treated with glucagon (Figure 5C). Consistently, acute salicylate i.p. injection significantly decreased fasting blood glucose in control mice (Figure 5D); such an effect was blocked in FKO mice (Figure 5E). However, salicylate administration did not enhance the suppressive effect of metformin on fasting blood glucose in control mice (Figure 5F). We then treated mice with 50 mg/kg body weight metformin and 125 mg/kg body weight salicylate through daily oral injection for 4 weeks. Consistently, chronic treatment of metformin significantly decreased fasting blood glucose in control mice, which was abolished in FKO mice. Salicylate treatment had little effect on fasting blood glucose and did not further enhance metformin-mediated reduction in fasting blood glucose in control mice. Hepatic Foxo1 deficiency blocked the suppressive effect of both metformin and salicylate on fasting blood glucose (Figures 5G,H). Chronic treatment of metformin and salicylate significantly deceased pyruvate-induced HGP by 17% and 14%, respectively, in control mice; however, salicylate treatment did not further enhance the metformin-mediated HGP suppression (Figure 5I). The inhibitory effects of metformin and salicylate on HGP during pyruvate tolerance test were diminished in FKO mice (Figure 5J). Thus, these results indicated that salicylate suppresses HGP through Foxo1 without further improvement on metformin-mediated HGP suppression.
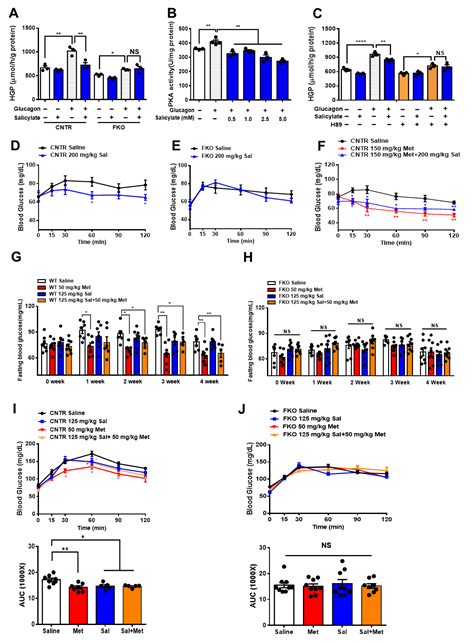
Figure 5. Salicylate suppresses glucagon-induced HGP through the PKA–Foxo1 signaling pathway in hepatocytes and has no improvement on metformin action on glucose homeostasis. (A) Primary hepatocytes isolated from control and FKO mice were pretreated with 0.5 mM of salicylate for 30 min and treated with 100 nM of glucagon for 3 h. Glucose content was measured, n = 3 independent experiments/group. (B) Control primary hepatocytes were pretreated with 0.5, 1, 2.5, and 5 mM of salicylate for 30 min, followed by 100 nM treatment for 1 h. PKA activity was measured, n = 3–4. (C) Control hepatocytes were pretreated with H89 and 0.5 mM of salicylate, followed by 100 nM glucagon treatment for 3 h. Glucose content was measured, n = 3 independent experiments/group. (D,E) Control (D) and FKO (E) mice were i.p. injected with 200 mg/kg body weight salicylate (Sal) after 16 h fasting. Blood glucose was monitored at indicated time points, n = 5–9 mice/group. (F) Control mice were i.p. injected with 150 mg/kg body weight metformin (Met) or 150 mg/kg body weight metformin (Met) + 200 mg/kg body weight salicylate (Sal) after 16 h fasting. Blood glucose was measured at indicated time points, n = 5–6 mice/group. (G,H) Control (G) and FKO (H) mice were administered with 50 mg/kg body weight metformin (Met), 125 mg/kg body weight salicylate (Sal), or 50 mg/kg body weight Met + 125 mg/kg body weight Sal via oral injection. Blood glucose was monitored after 16 h fasting, n = 5–8 mice/group. (I,J) Pyruvate tolerance test in control (I) and FKO (J) mice administered with 50 mg/kg body weight metformin (Met), 125 mg/kg body weight salicylate (Sal), or 50 mg/kg body weight Met + 125 mg/kg body weight Sal via oral injection, n = 5–8 mice/group. All data are presented as mean ± SEM. * p < 0.05, ** p < 0.01, NS: no significance, CNTR: control.
6. Metformin and Salicylate Improve HFD-induced Glucose Dysregulation through PKA-Foxo1 Pathway
We next evaluated the roles of metformin and salicylate in glucose homeostasis in diet-induced obese mice. The WT mice were fed with HFD for 6 weeks and then treated with metformin and salicylate for 7 weeks under HFD feeding. Both metformin and salicylate administrations significantly decreased fasting blood glucose levels by 20% and 21%, respectively, in the HFD-fed mice. However, salicylate treatment did not further enhance the suppressive effect of metformin on fasting blood glucose (Figure 6A). Consistently, the HFD feeding significantly impaired glucose tolerance and pyruvate tolerance, as compared with normal chow diet-fed control mice, and the effect of HFD was partially rescued by metformin or salicylate treatment. However, co-treatment of metformin and salicylate only resulted in an insignificant improvement in glucose tolerance and pyruvate-induced glucose output, as compared to metformin treatment group alone (Figures 6B,C). In addition to the anti-hyperglycemic effect, metformin showed a comparable effect in reducing the mRNA levels of pro-inflammatory markers in the liver of HFD-fed mice as compared to the salicylate treatment, but there was no enhanced effect of the combined treatment (Figure 6D). We further analyzed the protein expression in livers and found that HFD feeding activated the PKA®Foxo1-S273 phosphorylation; this was evident by increased levels of pan-PKA substrates’ phosphorylation, pFoxo1-S273, and protein abundance of total Foxo1 and G6PC (Figure 6E). Of note, metformin or/and salicylate alleviated the activation of the hepatic PKA®Foxo1 signaling pathway in the HFD-fed mice with a modest additive effect (Figure 6E). In addition, neither metformin nor salicylate treatment significantly altered serum insulin and glucagon levels (Figure 6F). Collectively, the above results suggest that metformin and salicylate improved glucose homeostasis through the PKA®Foxo1 signaling pathway and salicylate treatment does not significantly enhance the beneficial effect of metformin on glucose regulation in HFD-fed mice.
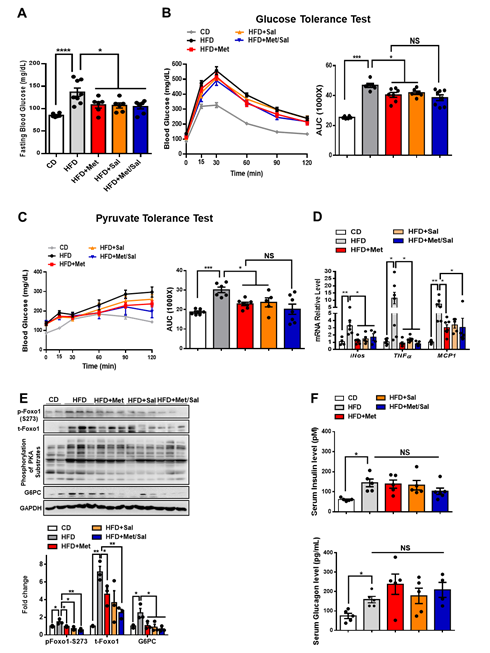
Figure 6. Metformin and salicylate improve glucose homeostasis in HFD-fed mice. (A) Fasting blood glucose level in HFD mice treated with metformin and salicylate for 7 weeks after 16 h fasting, n = 6–8 mice/group. (B) Glucose tolerance test in HFD mice treated with metformin and salicylate for 7 weeks, n = 6–8 mice/group. (C) Pyruvate tolerance test in HFD mice treated with metformin and salicylate for 7 weeks, n = 6–8 mice/group. (D) The mRNA expression levels of inflammatory factors in livers of 16 h overnight fasted HFD mice treated with metformin and salicylate for 7 weeks, n = 4–6 mice/group. (E) The protein levels of gluconeogenic genes in livers of 16 h overnight fasted HFD mice treated with metformin and salicylate for 7 weeks, n = 3 mice/group. (F) The insulin and glucagon levels in serum from HFD-fed mice treated with metformin and salicylate for 7 weeks, n = 4–5 mice/group. All data are presented as mean ± SEM. * p < 0.05, ** p < 0.01, *** p < 0.001, **** p < 0.0001. CD: chow diet.
7. The Effect of Metformin and Aspirin Combined Therapy on Blood Profiles in T2D Patients
Patients with T2D were next recruited and treated with either metformin or combined metformin and aspirin (abbreviated hereafter as Group M and Group M + A). Both M and M + A groups exhibited improvement in blood glucose profile, which is indicated by the significant decrease in HbA1c by 0.57 ± 0.14 (%) and 0.72 ± 0.19 (%), respectively, and the significant reduction in blood glucose by 1.10 ± 0.33 (mmol/L) and 1.22 ± 0.57 (mmol/L), respectively; however, aspirin administration did not significantly enhance the effect of metformin on blood glucose profile (Figures 7A,B). Blood insulin was significantly decreased in M group by 3.21 ± 1.14 (mIU/mL) and in M + A group by 1.70 ± 0.61 (mIU/mL), where no additional effect of salicylate was observed (Figure 7C). Metformin treatment group showed a significant reduction in blood glucagon level, which was not observed in metformin and salicylate co-treatment group (Figure 7D).
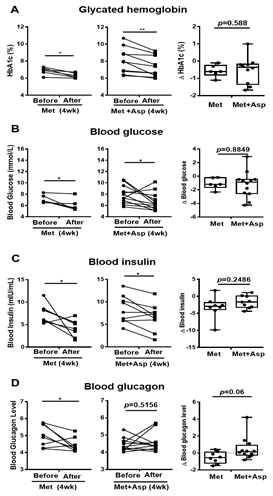
Figure 7. Blood profiles of clinical trial patients with metformin or metformin + aspirin for 4 weeks. (A–D) The HbA1c (A), glucose (B), insulin (C), and glucagon (D) profiles in type 2 diabetic patients’ blood before and after 4 week-treatment of metformin or metformin + aspirin, n = 6–12 patients/group. * p < 0.05, ** p < 0.01.