1. Introduction
2. Challenges
2.1. Photostability
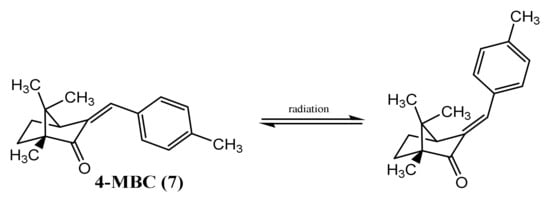
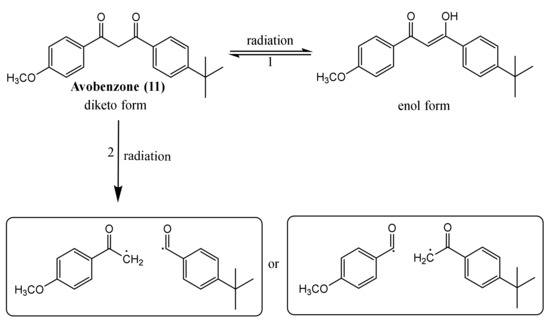

Strategies to Improve the Photostability of Organic UV Filters
2.2. Toxicity (Human and Environmental)
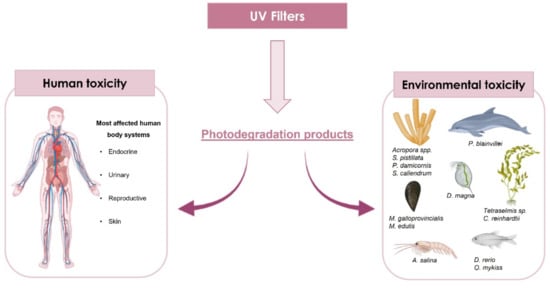
2.2.1. Human Safety
2.2.2. Environmental Safety
Corals
Other Marine Organisms
3. Prospects
3.1. Nature as a Source of Potential Photoprotective Agents and UV Filters
3.2. Synthetic Derivatives with Photoprotective and UV Filter Activity
3.2.1. Inorganic UV Filters
3.2.2. Organic UV Filters
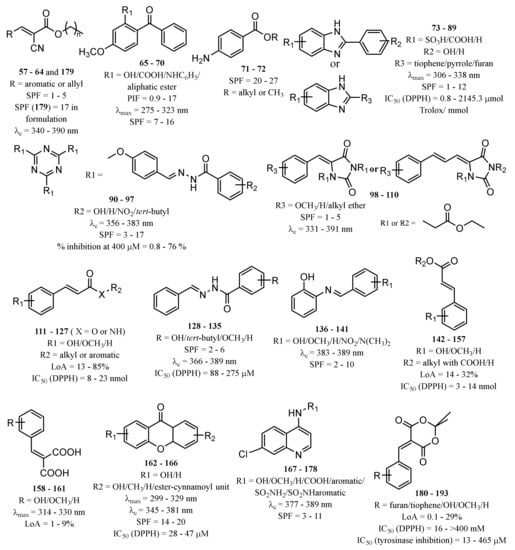
New Synthetic Derivatives Inspired by Commercialised UV Filters
Nature-Inspired Synthetised Compounds
Other New Synthetic Derivatives
4. Conclusions
Supplementary Materials
Author Contributions
Funding
Institutional Review Board Statement
Informed Consent Statement
Data Availability Statement
Acknowledgments
Conflicts of Interest
References
- Jussila, A.; Huotari-Orava, R.; Ylianttila, L.; Partonen, T.; Snellman, E. Narrow-band ultraviolet B radiation induces the expression of beta-endorphin in human skin in vivo. J. Photochem. Photobiol. B 2016, 155, 104–108. [Google Scholar] [CrossRef]
- Dahmane, R.; Pandel, R.; Trebse, P.; Poljsak, B. The role of sun exposure in skin aging. In Sun Exposure: Risk Factors, Protection Practices and Health Effects; Nova Science Publishers, Inc.: New York, NY, USA, 2015; pp. 1–40. [Google Scholar]
- Labille, J.; Catalano, R.; Slomberg, D.; Motellier, S.; Pinsino, A.; Hennebert, P.; Santaella, C.; Bartolomei, V. Assessing Sunscreen Lifecycle to Minimize Environmental Risk Posed by Nanoparticulate UV-Filters—A Review for Safer-by-Design Products. Front. Environ. Sci. 2020, 8, 00101. [Google Scholar] [CrossRef]
- Ma, Y.; Yoo, J. History of sunscreen: An updated view. J. Cosmet. Dermatol. 2021, 20, 1044–1049. [Google Scholar] [CrossRef] [PubMed]
- Pawlowski, S.; Petersen-Thiery, M. Sustainable sunscreens: A challenge between performance, animal testing ban, and human and environmental safety. In Handbook of Environmental Chemistry; Springer: Berlin/Heidelberg, Germany, 2020; Volume 94, pp. 185–207. [Google Scholar]
- Abiola, T.T.; Whittock, A.L.; Stavros, V.G. Unravelling the Photoprotective Mechanisms of Nature-Inspired Ultraviolet Filters Using Ultrafast Spectroscopy. Molecules 2020, 25, 3945. [Google Scholar] [CrossRef] [PubMed]
- L’Oreal. Garnier Ambre Solaire. 2020. Available online: https://www.loreal.com/it-it/italy/press-release/group/garnier-ambre-solaire/ (accessed on 3 August 2021).
- Roelandts, R.; Vanhee, J.; Bonamie, A.; Kerkhofs, L.; Degreef, H. A Survey of Ultraviolet Absorbers in Commercially Available Sun Products. Int. J. Dermatol. 1983, 22, 247–255. [Google Scholar] [CrossRef]
- Regulation (EC) No. 1223/2009 of the European Parliament and of the Council: Current Consolidated Version. Off. J. Eur. Union, (Last Current Consolidated Version Published on 1 October 2021); Available online: https://eur-lex.europa.eu/eli/reg/2009/1223/oj (accessed on 26 December 2021).
- Bissonnette, R. Update on Sunscreens. Skin Ther. Lett. 2008. Available online: https://www.skintherapyletter.com/sunscreen/advances-update/ (accessed on 1 October 2021).
- Chen, L.L.; Tooley, I.; Wang, S.Q. Nanotechnology in photoprotection. In Nanotechnology in Dermatology; Springer: New York, NY, USA, 2013; pp. 229–236. [Google Scholar]
- Valle-Sistac, J.; Molins-Delgado, D.; Díaz, M.; Ibáñez, L.; Barceló, D.; Silvia Díaz-Cruz, M. Determination of parabens and benzophenone-type UV filters in human placenta: First description of the existence of benzyl paraben and benzophenone-4. Environ. Int. 2016, 88, 243–249. [Google Scholar] [CrossRef]
- Rehfeld, A.; Egeberg, D.L.; Almstrup, K.; Petersen, J.H.; Dissing, S.; Skakkebæk, N.E. EDC IMPACT: Chemical UV filters can affect human sperm function in a progesterone-like manner. Endocr. Connect. 2018, 7, 16–25. [Google Scholar] [CrossRef]
- Bachelot, M.; Li, Z.; Munaron, D.; Le Gall, P.; Casellas, C.; Fenet, H.; Gomez, E. Organic UV filter concentrations in marine mussels from French coastal regions. Sci. Total Environ. 2012, 420, 273–279. [Google Scholar] [CrossRef]
- He, H.; Li, A.; Li, S.; Tang, J.; Li, L.; Xiong, L. Natural components in sunscreens: Topical formulations with sun protection factor (SPF). Biomed. Pharmacother. 2021, 134, 111161. [Google Scholar] [CrossRef]
- Giraldo, A.; Montes, R.; Rodil, R.; Quintana, J.B.; Vidal-Liñán, L.; Beiras, R. Ecotoxicological Evaluation of the UV Filters Ethylhexyl Dimethyl p-Aminobenzoic Acid and Octocrylene Using Marine Organisms Isochrysis galbana, Mytilus galloprovincialis and Paracentrotus lividus. Arch. Environ. Contam. Toxicol. 2017, 72, 606–611. [Google Scholar] [CrossRef] [PubMed]
- Lozano, C.; Givens, J.; Stien, D.; Matallana-Surget, S.; Lebaron, P. Bioaccumulation and toxicological effects of uv-filters on marine species. In Handbook of Environmental Chemistry; Springer: Berlin/Heidelberg, Germany, 2020; Volume 94, pp. 85–130. [Google Scholar]
- Bahia, M.F. Proteção Solar—Actualização, 1st ed.; Universidade do Porto: Porto, Portugal, 2003. [Google Scholar]
- Stiefel, C.; Schwack, W. Reactions of cosmetic UV filters with skin proteins: Model studies of esters with primary amines. Trends Photochem. Photobiol. 2013, 15, 105–116. [Google Scholar]
- Kockler, J.; Oelgemöller, M.; Robertson, S.; Glass, B.D. Photostability of sunscreens. J. Photochem. Photobiol. C Photochem. Rev. 2012, 13, 91–110. [Google Scholar] [CrossRef]
- Cefali, L.C.; Ataide, J.A.; Moriel, P.; Foglio, M.A.; Mazzola, P.G. Plant-based active photoprotectants for sunscreens. Int. J. Cosmet. Sci. 2016, 38, 346–353. [Google Scholar] [CrossRef] [PubMed]
- Vega, J.; Bonomi-Barufi, J.; Gomez-Pinchetti, J.L.; Figueroa, F.L. Cyanobacteria and Red Macroalgae as Potential Sources of Antioxidants and UV Radiation-Absorbing Compounds for Cosmeceutical Applications. Mar. Drugs 2020, 18, 659. [Google Scholar] [CrossRef] [PubMed]
- Solano, F. Photoprotection and skin pigmentation: Melanin-related molecules and some other new agents obtained from natural sources. Molecules 2020, 25, 1537. [Google Scholar] [CrossRef] [PubMed]
- Peyrot, C.; Mention, M.M.; Fournier, R.; Brunissen, F.; Couvreur, J.; Balaguer, P.; Allais, F. Expeditious and sustainable two-step synthesis of sinapoyl-l-malate and analogues: Towards non-endocrine disruptive bio-based and water-soluble bioactive compounds. Green Chem. 2020, 22, 6510–6518. [Google Scholar] [CrossRef]
- Reis, J.S.; Corrêa, M.A.; Ribeiro, C.A.; Dos Santos, J.L. Synthesis and evaluation of 1,3,5-triazine derivatives as sunscreens useful to prevent skin cancer. Bioorg. Med. Chem. Lett. 2019, 29, 126755. [Google Scholar] [CrossRef]
- Popiół, J.; Gunia-Krzyżak, A.; Słoczyńska, K.; Koczurkiewicz-Adamczyk, P.; Piska, K.; Wójcik-Pszczoła, K.; Żelaszczyk, D.; Krupa, A.; Żmudzki, P.; Marona, H.; et al. The involvement of xanthone and (E)-cinnamoyl chromophores for the design and synthesis of novel sunscreening agents. Int. J. Mol. Sci. 2021, 22, 34. [Google Scholar] [CrossRef]
- Herzog, B.; Wehrle, M.; Quass, K. Photostability of UV absorber systems in sunscreens. Photochem. Photobiol. 2009, 85, 869–878. [Google Scholar] [CrossRef]
- Bonda, C.A.; Lott, D. Sunscreen photostability. In Principles and Practice of Photoprotection; Springer International Publishing: Berlin/Heidelberg, Germany, 2016; pp. 247–273. [Google Scholar]
- Afonso, S.; Horita, K.; Sousa E Silva, J.P.; Almeida, I.F.; Amaral, M.H.; Lobão, P.A.; Costa, P.C.; Miranda, M.S.; Esteves Da Silva, J.C.G.; Sousa Lobo, J.M. Photodegradation of avobenzone: Stabilization effect of antioxidants. J. Photochem. Photobiol. B Biol. 2014, 140, 36–40. [Google Scholar] [CrossRef] [PubMed]
- Tarras-Wahlberg, N.; Stenhagen, G.; Larko, O.; Rosen, A.; Wennberg, A.M.; Wennerstrom, O. Changes in ultraviolet absorption of sunscreens after ultraviolet irradiation. J. Investig. Dermatol. 1999, 113, 547–553. [Google Scholar] [CrossRef] [PubMed]
- Schwack, W.; Rudolph, T. Photochemistry of dibenzoyl methane UVA filters—Part 1. J. Photochem. Photobiol. B Biol. 1995, 28, 229–234. [Google Scholar] [CrossRef]
- Downs, C.A.; DiNardo, J.C.; Stien, D.; Rodrigues, A.M.S.; Lebaron, P. Benzophenone Accumulates over Time from the Degradation of Octocrylene in Commercial Sunscreen Products. Chem. Res. Toxicol. 2021, 34, 1046–1054. [Google Scholar] [CrossRef]
- Roscher, N.M.; Lindemann, M.K.O.; Bin Kong, S.; Cho, C.G.; Jiang, P. Photodecomposition of several compounds commonly used as sunscreen agents. J. Photochem. Photobiol. A Chem. 1994, 80, 417–421. [Google Scholar] [CrossRef]
- Duarte, J.; Almeida, I.F.; Costa, M.; Da Silva, E.S.; Faria, J.L.; Sousa Lobo, J.M.; Costa, P.C.; Scalia, S. Alginate microparticles as carriers for the UV filter 2-ethylhexyl 4-methoxycinnamate: Influence on photostability. Int. J. Cosmet. Sci. 2019, 41, 585–593. [Google Scholar] [CrossRef] [PubMed]
- Chatelain, E.; Gabard, B. Photostabilization of butyl methoxydibenzoylmethane (Avobenzone) and ethylhexyl methoxycinnamate by bis-ethylhexyloxyphenol methoxyphenyl triazine (Tinosorb S), a new UV broadband filter. Photochem. Photobiol. 2001, 74, 401–406. [Google Scholar] [CrossRef]
- Kockler, J.; Oelgemöller, M.; Robertson, S.; Glass, B.D. Influence of titanium dioxide particle size on the photostability of the chemical UV-filters butyl methoxy dibenzoylmethane and octocrylene in a microemulsion. Cosmetics 2014, 1, 128–139. [Google Scholar] [CrossRef]
- Freitas, J.V.; Lopes, N.P.; Gaspar, L.R. Photostability evaluation of five UV-filters, trans-resveratrol and beta-carotene in sunscreens. Eur. J. Pharm. Sci. 2015, 78, 79–89. [Google Scholar] [CrossRef]
- Karpkird, T.; Khunsakorn, R.; Noptheeranuphap, C.; Midpanon, S. Inclusion complexes and photostability of UV filters and curcumin with beta-cyclodextrin polymers: Effect on cross-linkers. J. Incl. Phenom. Macrocycl. Chem. 2018, 91, 37–45. [Google Scholar] [CrossRef]
- Paris, C.; Lhiaubet-Vallet, V.; Jimenez, O.; Trullas, C.; Miranda, M.A. A blocked diketo form of avobenzone: Photostability, photosensitizing properties and triplet quenching by a triazine-derived UVB-filter. Photochem. Photobiol. 2009, 85, 178–184. [Google Scholar] [CrossRef] [PubMed]
- Coelho, L.; Almeida, I.F.; Sousa Lobo, J.M.; Sousa, E.S.J.P. Photostabilization strategies of photosensitive drugs. Int. J. Pharm. 2018, 541, 19–25. [Google Scholar] [CrossRef] [PubMed]
- De Oliveira, C.A.; Dario, M.F.; Sarruf, F.D.; Mariz, I.F.A.; Velasco, M.V.R.; Rosado, C.; Baby, A.R. Safety and efficacy evaluation of gelatin-based nanoparticles associated with UV filters. Colloids Surf. B Biointerfaces 2016, 140, 531–537. [Google Scholar] [CrossRef] [PubMed]
- Graziola, F.; Candido, T.M.; De Oliveira, C.A.; Peres, D.D.; Issa, M.G.; Mota, J.; Rosado, C.; Consiglieri, V.O.; Kaneko, T.M.; Velasco, M.V.R.; et al. Gelatin-based microspheres crosslinked with glutaraldehyde and rutin oriented to cosmetics. Bras. J. Pharm. Sci. 2016, 52, 603–612. [Google Scholar] [CrossRef]
- Oliveira, C.A.D.; Peres, D.D.; Graziola, F.; Chacra, N.A.B.; Araújo, G.L.B.D.; Flórido, A.C.; Mota, J.; Rosado, C.; Velasco, M.V.R.; Rodrigues, L.M.; et al. Cutaneous biocompatible rutin-loaded gelatin-based nanoparticles increase the SPF of the association of UVA and UVB filters. Eur. J. Pharm. Sci. 2016, 81, 1–9. [Google Scholar] [CrossRef]
- Al-Rawashdeh, N.A.F.; Al-Sadeh, K.S.; Al-Bitar, M.B. Physicochemical study on microencapsulation of hydroxypropyl-β-cyclodextrin in dermal preparations. Drug Dev. Ind. Pharm. 2010, 36, 688–697. [Google Scholar] [CrossRef]
- Scalia, S.; Casolari, A.; Iaconinoto, A.; Simeoni, S. Comparative studies of the influence of cyclodextrins on the stability of the sunscreen agent, 2-ethylhexyl-p-methoxycinnamate. J. Pharm. Biomed. Anal. 2002, 30, 1181–1189. [Google Scholar] [CrossRef]
- Scalia, S.; Tursilli, R.; Iannuccelli, V. Complexation of the sunscreen agent, 4-methylbenzylidene camphor with cyclodextrins: Effect on photostability and human stratum corneum penetration. J. Pharm. Biomed. Anal. 2007, 44, 29–34. [Google Scholar] [CrossRef]
- Shokri, J.; Hasanzadeh, D.; Ghanbarzadeh, S.; Dizadji-Ilkhchi, M.; Adibkia, K. The effect of beta-cyclodextrin on percutaneous absorption of commonly used Eusolex® sunscreens. Drug Res. 2013, 63, 591–596. [Google Scholar] [CrossRef]
- Gonzalez, H.; Tarras-Wahlberg, N.; Stromdahl, B.; Juzeniene, A.; Moan, J.; Larko, O.; Rosen, A.; Wennberg, A.M. Photostability of commercial sunscreens upon sun exposure and irradiation by ultraviolet lamps. BMC Dermatol. 2007, 7, 1. [Google Scholar] [CrossRef]
- Shaath, N.A. Ultraviolet filters. Photochem. Photobiol. Sci. 2010, 9, 464–469. [Google Scholar] [CrossRef] [PubMed]
- Huong, S.P.; Rocher, E.E.; Fourneron, J.-D.; Charles, L.; Monnier, V.; Bun, H.; Andrieu, V. Photoreactivity of the sunscreen butylmethoxydibenzoylmethane (DBM) under various experimental conditions. J. Photochem. Photobiol. A Chem. 2008, 196, 106–112. [Google Scholar] [CrossRef]
- Juliano, C.; Magrini, G.A. Cosmetic ingredients as emerging pollutants of environmental and health concern. A mini-review. Cosmetics 2017, 4, 11. [Google Scholar] [CrossRef]
- Bordalo, D.; Leite, C.; Almeida, Â.; Soares, A.M.V.M.; Pretti, C.; Freitas, R. Impacts of UV filters in Mytilus galloprovincialis: Preliminary data on the acute effects induced by environmentally relevant concentrations. Sustainability 2020, 12, 6852. [Google Scholar] [CrossRef]
- Picot Groz, M.; Martinez Bueno, M.J.; Rosain, D.; Fenet, H.; Casellas, C.; Pereira, C.; Maria, V.; Bebianno, M.J.; Gomez, E. Detection of emerging contaminants (UV filters, UV stabilizers and musks) in marine mussels from Portuguese coast by QuEChERS extraction and GC-MS/MS. Sci. Total Environ. 2014, 493, 162–169. [Google Scholar] [CrossRef]
- Cunha, S.C.; Fernandes, J.O.; Vallecillos, L.; Cano-Sancho, G.; Domingo, J.L.; Pocurull, E.; Borrull, F.; Maulvault, A.L.; Ferrari, F.; Fernandez-Tejedor, M.; et al. Co-occurrence of musk fragrances and UV-filters in seafood and macroalgae collected in European hotspots. Environ. Res. 2015, 143, 65–71. [Google Scholar] [CrossRef]
- Gadelha, J.R.; Rocha, A.C.; Camacho, C.; Eljarrat, E.; Peris, A.; Aminot, Y.; Readman, J.W.; Boti, V.; Nannou, C.; Kapsi, M.; et al. Persistent and emerging pollutants assessment on aquaculture oysters (Crassostrea gigas) from NW Portuguese coast (Ria De Aveiro). Sci. Total Environ. 2019, 666, 731–742. [Google Scholar] [CrossRef]
- Mitchelmore, C.L.; He, K.; Gonsior, M.; Hain, E.; Heyes, A.; Clark, C.; Younger, R.; Schmitt-Kopplin, P.; Feerick, A.; Conway, A.; et al. Occurrence and distribution of UV-filters and other anthropogenic contaminants in coastal surface water, sediment, and coral tissue from Hawaii. Sci. Total Environ. 2019, 670, 398–410. [Google Scholar] [CrossRef]
- Shick, J.M.; Lesser, M.P.; Jokiel, P.L. Effects of ultraviolet radiation on corals and other coral reef organisms. Glob. Chang. Biol. 1996, 2, 527–545. [Google Scholar] [CrossRef]
- Wijgerde, T.; van Ballegooijen, M.; Nijland, R.; van der Loos, L.; Kwadijk, C.; Osinga, R.; Murk, A.; Slijkerman, D. Adding insult to injury: Effects of chronic oxybenzone exposure and elevated temperature on two reef-building corals. Sci. Total Environ. 2020, 733, 139030. [Google Scholar] [CrossRef]
- Corinaldesi, C.; Marcellini, F.; Nepote, E.; Damiani, E.; Danovaro, R. Impact of inorganic UV filters contained in sunscreen products on tropical stony corals (Acropora spp.). Sci. Total Environ. 2018, 637–638, 1279–1285. [Google Scholar] [CrossRef] [PubMed]
- Cahova, J.; Blahova, J.; Marsalek, P.; Doubkova, V.; Franc, A.; Garajová, M.; Tichy, F.; Mares, J.; Svobodova, Z. The biological activity of the organic UV filter ethylhexyl methoxycinnamate in rainbow trout (Oncorhynchus mykiss). Sci. Total Environ. 2021, 774, 145570. [Google Scholar] [CrossRef] [PubMed]
- Gago-Ferrero, P.; Díaz-Cruz, M.S.; Barceló, D. UV filters bioaccumulation in fish from Iberian river basins. Sci. Total Environ. 2015, 518–519, 518–525. [Google Scholar] [CrossRef] [PubMed]
- Balmer, M.E.; Buser, H.R.; Müller, M.D.; Poiger, T. Occurrence of some organic UV filters in wastewater, in surface waters, and in fish from Swiss lakes. Environ. Sci. Technol. 2005, 39, 953–962. [Google Scholar] [CrossRef] [PubMed]
- Gago-Ferrero, P.; Alonso, M.B.; Bertozzi, C.P.; Marigo, J.; Barbosa, L.; Cremer, M.; Secchi, E.R.; Azevedo, A.; Lailson-Brito, J., Jr.; Torres, J.P.M.; et al. First determination of UV filters in marine mammals. octocrylene levels in Franciscana dolphins. Environ. Sci. Technol. 2013, 47, 5619–5625. [Google Scholar] [CrossRef]
- Wawrzynczak, A.; Feliczak-Guzik, A.; Nowak, I. Nanosunscreens: From nanoencapsulated to nanosized cosmetic active forms. In Nanobiomaterials in Galenic Formulations and Cosmetics: Applications of Nanobiomaterials; Elsevier Inc.: Amsterdam, The Netherlands, 2016; pp. 25–46. [Google Scholar]
- Wang, S.Q.; Balagula, Y.; Osterwalder, U. Photoprotection: A Review of the Current and Future Technologies. Dermatol. Ther. 2010, 23, 31–47. [Google Scholar] [CrossRef] [PubMed]
- Janjua, N.R.; Mogensen, B.; Andersson, A.M.; Petersen, J.H.; Henriksen, M.; Skakkebaek, N.E.; Wulf, H.C. Systemic absorption of the sunscreens benzophenone-3, octyl-methoxycinnamate, and 3-(4-methyl-benzylidene) camphor after whole-body topical application and reproductive hormone levels in humans. J. Invest. Dermatol. 2004, 123, 57–61. [Google Scholar] [CrossRef]
- Rodriguez, I.; Fenollosa, R.; Meseguer, F. Silicon Microspheres as UV, Visible and Infrared Filters for Cosmetics. Available online: https://www.cosmeticsandtoiletries.com/formulating/function/uvfilter/premium-Silicon-Microspheres-as-UV-Visible-and-Infrared-Filters-for-Cosmetics-208701831.html (accessed on 12 July 2021).
- Ambrogi, V.; Latterini, L.; Marmottini, F.; Pagano, C.; Ricci, M. Mesoporous silicate MCM-41 as a particulate carrier for octyl methoxycinnamate: Sunscreen release and photostability. J. Pharm. Sci. 2013, 102, 1468–1475. [Google Scholar] [CrossRef]
- Revised Guidance Document 150 on Standardised Test Guidelines for Evaluating Chemicals for Endocrine Disruption; OECD Publishing: Paris, France, 2018.
- Witorsch, R.J.; Thomas, J.A. Personal care products and endocrine disruption: A critical review of the literature. Crit. Rev. Toxicol. 2010, 40, 515563. [Google Scholar] [CrossRef]
- Rehfeld, A.; Dissing, S.; Skakkebæk, N.E. Chemical UV filters mimic the effect of progesterone on Ca2+ signaling in human sperm cells. Endocrinology 2016, 157, 4297–4308. [Google Scholar] [CrossRef]
- Ponzo, O.J.; Silvia, C. Evidence of reproductive disruption associated with neuroendocrine changes induced by UV-B filters, phtalates and nonylphenol during sexual maturation in rats of both gender. Toxicology 2013, 311, 41–51. [Google Scholar] [CrossRef] [PubMed]
- Axelstad, M.; Boberg, J.; Hougaard, K.S.; Christiansen, S.; Jacobsen, P.R.; Mandrup, K.R.; Nellemann, C.; Lund, S.P.; Hass, U. Effects of pre- and postnatal exposure to the UV-filter Octyl Methoxycinnamate (OMC) on the reproductive, auditory and neurological development of rat offspring. Toxicol. Appl. Pharmacol. 2011, 250, 278–290. [Google Scholar] [CrossRef] [PubMed]
- Ozáez, I.; Martínez-Guitarte, J.L.; Morcillo, G. Effects of in vivo exposure to UV filters (4-MBC, OMC, BP-3, 4-HB, OC, OD-PABA) on endocrine signaling genes in the insect Chironomus riparius. Sci. Total Environ. 2013, 456, 120–126. [Google Scholar] [CrossRef] [PubMed]
- Li, A.J.; Law, J.C.F.; Chow, C.H.; Huang, Y.; Li, K.; Leung, K.S.Y. Joint Effects of Multiple UV Filters on Zebrafish Embryo Development. Environ. Sci. Technol. 2018, 52, 9460–9467. [Google Scholar] [CrossRef] [PubMed]
- Li, V.W.T.; Tsui, M.P.M.; Chen, X.; Hui, M.N.Y.; Jin, L.; Lam, R.H.W.; Yu, R.M.K.; Murphy, M.B.; Cheng, J.; Lam, P.K.S.; et al. Effects of 4-methylbenzylidene camphor (4-MBC) on neuronal and muscular development in zebrafish (Danio rerio) embryos. Environ. Sci. Pollut. Res. 2016, 23, 8275–8285. [Google Scholar] [CrossRef]
- Lorigo, M.; Mariana, M.; Cairrao, E. Photoprotection of ultraviolet-B filters: Updated review of endocrine disrupting properties. Steroids 2018, 131, 46–58. [Google Scholar] [CrossRef]
- Balázs, A.; Krifaton, C.; Orosz, I.; Szoboszlay, S.; Kovács, R.; Csenki, Z.; Urbányi, B.; Kriszt, B. Hormonal activity, cytotoxicity and developmental toxicity of UV filters. Ecotoxicol. Environ. Saf. 2016, 131, 45–53. [Google Scholar] [CrossRef]
- Chen, T.H.; Hsieh, C.Y.; Ko, F.C.; Cheng, J.O. Effect of the UV-filter benzophenone-3 on intra-colonial social behaviors of the false clown anemonefish (Amphiprion ocellaris). Sci. Total Environ. 2018, 644, 1625–1629. [Google Scholar] [CrossRef]
- Carvalhais, A.; Pereira, B.; Sabato, M.; Seixas, R.; Dolbeth, M.; Marques, A.; Guilherme, S.; Pereira, P.; Pacheco, M.; Mieiro, C. Mild effects of sunscreen agents on a marine flatfish: Oxidative stress, energetic profiles, neurotoxicity and behaviour in response to titanium dioxide nanoparticles and oxybenzone. Int. J. Mol. Sci. 2021, 22, 1567. [Google Scholar] [CrossRef]
- Pablos, M.V.; Garcia-Hortiguela, P.; Fernandez, C. Acute and chronic toxicity of emerging contaminants, alone or in combination, in Chlorella vulgaris and Daphnia magna. Environ. Sci. Pollut. Res. Int. 2015, 22, 5417–5424. [Google Scholar] [CrossRef]
- Elder, D.P.; Snodin, D.J. Drug substances presented as sulfonic acid salts: Overview of utility, safety and regulation. J. Pharm. Pharmacol. 2009, 61, 269–278. [Google Scholar] [CrossRef] [PubMed]
- Lozano, C.; Matallana-Surget, S.; Givens, J.; Nouet, S.; Arbuckle, L.; Lambert, Z.; Lebaron, P. Toxicity of UV filters on marine bacteria: Combined effects with damaging solar radiation. Sci. Total Environ. 2020, 722, 1377803. [Google Scholar] [CrossRef] [PubMed]
- Huang, Y.; Law, J.C.F.; Lam, T.K.; Leung, K.S.Y. Risks of organic UV filters: A review of environmental and human health concern studies. Sci. Total Environ. 2021, 755, 142486. [Google Scholar] [CrossRef] [PubMed]
- Pico, Y.; Belenguer, V.; Corcellas, C.; Diaz-Cruz, M.S.; Eljarrat, E.; Farre, M.; Gago-Ferrero, P.; Huerta, B.; Navarro-Ortega, A.; Petrovic, M.; et al. Contaminants of emerging concern in freshwater fish from four Spanish Rivers. Sci. Total Environ. 2019, 659, 1186–1198. [Google Scholar] [CrossRef]
- Duis, K.; Junker, T.; Coors, A. Review of the environmental fate and effects of two UV filter substances used in cosmetic products. Sci. Total Environ. 2022, 808, 151931. [Google Scholar] [CrossRef]
- Mitchelmore, C.L.; Burns, E.E.; Conway, A.; Heyes, A.; Davies, I.A. A Critical Review of Organic Ultraviolet Filter Exposure, Hazard, and Risk to Corals. Environ. Toxicol. Chem. 2021. [Google Scholar] [CrossRef]
- Tsui, M.M.P.; Lam, J.C.W.; Ng, T.Y.; Ang, P.O.; Murphy, M.B.; Lam, P.K.S. Occurrence, Distribution, and Fate of Organic UV Filters in Coral Communities. Environ. Sci. Technol. 2017, 51, 4182–4190. [Google Scholar] [CrossRef]
- He, T.; Tsui, M.M.P.; Tan, C.J.; Ng, K.Y.; Guo, F.W.; Wang, L.H.; Chen, T.H.; Fan, T.Y.; Lam, P.K.S.; Murphy, M.B. Comparative toxicities of four benzophenone ultraviolet filters to two life stages of two coral species. Sci. Total Environ. 2019, 651, 2391–2399. [Google Scholar] [CrossRef]
- Downs, C.A.; Kramarsky-Winter, E.; Segal, R.; Fauth, J.; Knutson, S.; Bronstein, O.; Ciner, F.R.; Jeger, R.; Lichtenfeld, Y.; Woodley, C.M.; et al. Toxicopathological Effects of the Sunscreen UV Filter, Oxybenzone (Benzophenone-3), on Coral Planulae and Cultured Primary Cells and Its Environmental Contamination in Hawaii and the U.S. Virgin Islands. Arch. Environ. Contam. Toxicol. 2016, 70, 265–288. [Google Scholar] [CrossRef]
- The Senate, TWENTY-NINTH LEGISLATURE, State of Hawaii. Available online: https://www.capitol.hawaii.gov/session2018/bills/SB2571_.HTM (accessed on 29 April 2021).
- Danovaro, R.; Bongiorni, L.; Corinaldesi, C.; Giovannelli, D.; Damiani, E.; Astolfi, P.; Greci, L.; Pusceddu, A. Sunscreens cause coral bleaching by promoting viral infections. Environ. Health Perspect. 2008, 116, 441–447. [Google Scholar] [CrossRef]
- Thorel, E.; Clergeaud, F.; Jaugeon, L.; Rodrigues, A.M.S.; Lucas, J.; Stien, D.; Lebaron, P. Effect of 10 UV filters on the brine shrimp Artemia salina and themarinemicroalga Tetraselmis sp. Toxics 2020, 8, 29. [Google Scholar] [CrossRef] [PubMed]
- Mao, F.; He, Y.; Gin, K.H. Evaluating the joint toxicity of two benzophenone-type UV filters on the green alga Chlamydomonas reinhardtii with response surface methodology. Toxics 2018, 6, 8. [Google Scholar] [CrossRef] [PubMed]
- Boyd, A.; Stewart, C.B.; Philibert, D.A.; How, Z.T.; El-Din, M.G.; Tierney, K.B.; Blewett, T.A. A burning issue: The effect of organic ultraviolet filter exposure on the behaviour and physiology of Daphnia magna. Sci. Total Environ. 2021, 750, 141707. [Google Scholar] [CrossRef] [PubMed]
- Quintaneiro, C.; Teixeira, B.; Benedé, J.L.; Chisvert, A.; Soares, A.M.V.M.; Monteiro, M.S. Toxicity effects of the organic UV-filter 4-Methylbenzylidene camphor in zebrafish embryos. Chemosphere 2019, 218, 273–281. [Google Scholar] [CrossRef] [PubMed]
- Pawlowski, S.; Herzog, B.; Sohn, M.; Petersen-Thiery, M.; Acker, S. EcoSun Pass: A tool to evaluate the ecofriendliness of UV filters used in sunscreen products. Int. J. Cosmet. Sci. 2021, 43, 201–210. [Google Scholar] [CrossRef] [PubMed]
- Krutmann, J.; Passeron, T.; Gilaberte, Y.; Granger, C.; Leone, G.; Narda, M.; Schalka, S.; Trullas, C.; Masson, P.; Lim, H.W. Photoprotection of the future: Challenges and opportunities. J. Eur. Acad. Dermatol. Venereol. 2020, 34, 447–454. [Google Scholar] [CrossRef] [PubMed]
- Yarovaya, L.; Waranuch, N.; Wisuitiprot, W.; Khunkitti, W. Effect of grape seed extract on skin fibroblasts exposed to UVA light and its photostability in sunscreen formulation. J. Cosmet. Dermatol. 2020, 20, 1271–1282. [Google Scholar] [CrossRef]
- Hubner, A.; Sobreira, F.; Neto, A.V.; de Oliveira Pinto, C.A.S.; Dario, M.F.; Díaz, I.E.C.; Lourenço, F.R.; Rosado, C.; Baby, A.R.; Bacchi, E.M. The synergistic behavior of antioxidant phenolic compounds obtained fromwinemaking waste’s valorization, increased the efficacy of a sunscreen system. Antioxidants 2019, 8, 530. [Google Scholar] [CrossRef]
- Da Silva, A.C.P.; Paiva, J.P.; Diniz, R.R.; dos Anjos, V.M.; Silva, A.B.S.M.; Pinto, A.V.; dos Santos, E.P.; Leitão, A.C.; Cabral, L.M.; Rodrigues, C.R.; et al. Photoprotection assessment of olive (Olea europaea L.) leaves extract standardized to oleuropein: In vitro and in silico approach for improved sunscreens. J. Photochem. Photobiol. B Biol. 2019, 193, 162–171. [Google Scholar] [CrossRef]
- Polonini, H.C.; Brandão, M.A.F.; Raposo, N.R.B. A natural broad-spectrum sunscreen formulated from the dried extract of Brazilian Lippia sericea as a single UV filter. RSC Adv. 2014, 4, 62566–62575. [Google Scholar] [CrossRef]
- Alves, G.D.A.D.; de Souza, R.O.; Rogez, H.; Masaki, H.; Fonseca, M.J.V. Cecropia obtusa, an Amazonian ethanolic extract, exhibits photochemoprotective effect in vitro and balances the redox cellular state in response to UV radiation. Ind. Crops Prod. 2016, 94, 893–902. [Google Scholar] [CrossRef]
- Anurukvorakun, O.; Boonruang, R.; Lahpun, N. Formulation strategy, stability issues, safety and efficacy evaluations of Acacia catechu whitening cream. Int. J. Appl. Pharm. 2019, 11, 91–96. [Google Scholar] [CrossRef]
- Wróblewska, K.B.; Baby, A.R.; Grombone Guaratini, M.T.; Moreno, P.R.H. In vitro antioxidant and photoprotective activity of five native Brazilian bamboo species. Ind. Crops Prod. 2019, 130, 208–215. [Google Scholar] [CrossRef]
- Lohezic-Le Devehat, F.; Legouin, B.; Couteau, C.; Boustie, J.; Coiffard, L. Lichenic extracts and metabolites as UV filters. J. Photochem. Photobiol. B Biol. 2013, 120, 17–28. [Google Scholar] [CrossRef] [PubMed]
- Lee, S.C.; Lee, S.H.; Won, K. Wood Powder as a New Natural Sunscreen Ingredient. Biotechnol. Bioprocess Eng. 2019, 24, 258–263. [Google Scholar] [CrossRef]
- Rasheed, A.; Shama, S.N.; Mohanalakshmi, S.; Ravichandran, V. Formulation, characterization and in vitro evaluation of herbal sunscreen lotion. Orient. Pharm. Exp. Med. 2012, 12, 241–246. [Google Scholar] [CrossRef]
- Widiyati, E. Determination of ultraviolet filter activity on coconut oil cosmetic cream. AIP Conf. Proc. 2017, 1868, 020004. [Google Scholar] [CrossRef]
- Bhattacharya, S.; Sherje, A.P. Development of resveratrol and green tea sunscreen formulation for combined photoprotective and antioxidant properties. J. Drug Deliv. Sci. Technol. 2020, 60, 102000. [Google Scholar] [CrossRef]
- L’Alloret, F.; Candau, D.; Seité, S.; Pygmalion, M.J.; Ruiz, L.; Josso, M.; Meaudre, H.; Gauchet, L.; Pena, A.M.; Colonna, A. New combination of ultraviolet absorbers in an oily emollient increases sunscreen efficacy and photostability. Dermatol. Ther. 2012, 2, 4. [Google Scholar] [CrossRef]
- Montenegro, L.; Santagati, L.M. Use of vegetable oils to improve the sun protection factor of sunscreen formulations. Cosmetics 2019, 6, 25. [Google Scholar] [CrossRef]
- Mota, M.D.; Costa, R.Y.S.; Guedes, A.A.S.; Silva, L.C.R.C.E.; Chinalia, F.A. Guava-fruit extract can improve the UV-protection efficiency of synthetic filters in sun cream formulations. J. Photochem. Photobiol. B Biol. 2019, 201, 111639. [Google Scholar] [CrossRef] [PubMed]
- Milani, L.P.G.; Garcia, N.O.S.; Morais, M.C.; Dias, A.L.S.; Oliveira, N.L.; Conceição, E.C. Extract from byproduct Psidium guajava standardized in ellagic acid: Additivation of the in vitro photoprotective efficacy of a cosmetic formulation. Rev. Bras. Farmacogn. 2018, 28, 692–696. [Google Scholar] [CrossRef]
- Niculae, G.; Lacatusu, I.; Badea, N.; Stan, R.; Vasile, B.S.; Meghea, A. Rice bran and raspberry seed oil-based nanocarriers with self-antioxidative properties as safe photoprotective formulations. Photochem. Photobiol. Sci. 2014, 13, 703–716. [Google Scholar] [CrossRef]
- Almeida, W.A.D.S.; Antunes, A.D.S.; Penido, R.G.; Correa, H.S.D.G.; Nascimento, A.M.D.; Andrade, Â.L.; Santos, V.R.; Cazati, T.; Amparo, T.R.; Souza, G.H.B.D.; et al. Photoprotective activity and increase of SPF in sunscreen formulation using lyophilized red propolis extracts from Alagoas. Rev. Bras. Farmacogn. 2019, 29, 373–380. [Google Scholar] [CrossRef]
- Llewellyn, C.A.; Airs, R.L. Distribution and abundance of MAAs in 33 species of microalgae across 13 classes. Mar. Drugs 2010, 8, 1273–1291. [Google Scholar] [CrossRef] [PubMed]
- Liu, Z. Occurrence of mycosporine-like amino acids (MAAs) in the bloom-forming cyanobacterium Microcystis aeruginosa. J. Plankton Res. 2004, 26, 963–966. [Google Scholar] [CrossRef]
- Rangel, K.C.; Villela, L.Z.; Pereira, K.D.C.; Colepicolo, P.; Debonsi, H.M.; Gaspar, L.R. Assessment of the photoprotective potential and toxicity of Antarctic red macroalgae extracts from Curdiea racovitzae and Iridaea cordata for cosmetic use. Algal Res. 2020, 50, 101984. [Google Scholar] [CrossRef]
- Prasedya, E.S.; Syafitri, S.M.; Geraldine, B.A.F.D.; Hamdin, C.D.; Frediansyah, A.; Miyake, M.; Kobayashi, D.; Hazama, A.; Sunarpi, H. UVA photoprotective activity of brown macroalgae Sargassum cristafolium. Biomedicines 2019, 7, 77. [Google Scholar] [CrossRef]
- Proteau, P.J.; Gerwick, W.H.; Garcia-Pichel, F.; Castenholz, R. The structure of scytonemin, an ultraviolet sunscreen pigment from the sheaths of cyanobacteria. Experientia 1993, 49, 825–829. [Google Scholar] [CrossRef]
- Kang, M.R.; Jo, S.A.; Lee, H.; Yoon, Y.D.; Kwon, J.H.; Yang, J.W.; Choi, B.J.; Park, K.H.; Lee, M.Y.; Lee, C.W.; et al. Inhibition of Skin Inflammation by Scytonemin, an Ultraviolet Sunscreen Pigment. Mar. Drugs 2020, 18, 300. [Google Scholar] [CrossRef]
- Teixeira, T.R.; Rangel, K.C.; Tavares, R.S.N.; Kawakami, C.M.; Dos Santos, G.S.; Maria-Engler, S.S.; Colepicolo, P.; Gaspar, L.R.; Debonsi, H.M. In Vitro Evaluation of the Photoprotective Potential of Quinolinic Alkaloids Isolated from the Antarctic Marine Fungus Penicillium echinulatum for Topical Use. Mar. Biotechnol. 2021, 23, 357–372. [Google Scholar] [CrossRef] [PubMed]
- Maciel, O.M.C.; Tavares, R.S.N.; Caluz, D.R.E.; Gaspar, L.R.; Debonsi, H.M. Photoprotective potential of metabolites isolated from algae-associated fungi Annulohypoxylon stygium. J. Photochem. Photobiol. B Biol. 2018, 178, 316–322. [Google Scholar] [CrossRef] [PubMed]
- Regalado, E.L.; Rodriguez, M.; Menendez, R.; Concepcion, A.A.; Nogueiras, C.; Laguna, A.; Rodriguez, A.A.; Williams, D.E.; Lorenzo-Luaces, P.; Valdes, O.; et al. Repair of UVB-damaged skin by the antioxidant sulphated flavone glycoside thalassiolin B isolated from the marine plant Thalassia testudinum Banks ex Konig. Mar. Biotechnol. 2009, 11, 74–80. [Google Scholar] [CrossRef] [PubMed]
- Ahmed, F.E.; Setlow, R.B. Ultraviolet Radiation-induced DNA Damage and Its Photorepair in the Skin of the Platyfish Xiphophorus. Cancer Res. 1993, 53, 2249–2255. [Google Scholar]
- Ryu, B.; Qian, Z.-J.; Kim, M.-M.; Nam, K.W.; Kim, S.-K. Anti-photoaging activity and inhibition of matrix metalloproteinase (MMP) by marine red alga, Corallina pilulifera methanol extract. Radiat. Phys. Chem. 2009, 78, 98–105. [Google Scholar] [CrossRef]
- Yabe, S.; Sato, T. Cerium oxide for sunscreen cosmetics. J. Solid State Chem. 2003, 171, 7–11. [Google Scholar] [CrossRef]
- Seixas, V.C.; Serra, O.A. Stability of sunscreens containing CePO4: Proposal for a new inorganic UV filter. Molecules 2014, 19, 9907–9925. [Google Scholar] [CrossRef]
- Polonini, H.C.; Lopes, R.S.; Beatriz, A.; Gomes, R.S.; Silva, A.O.; De Lima, R.V.; Nunes, G.A.; Brandão, M.A.F.; Raposo, N.R.B.; De Lima, D.P. Synthesis and evaluation of octocrylene-inspired compounds for Uv-filter activity. Quim. Nova 2014, 37, 1004–1009. [Google Scholar] [CrossRef]
- González, M.T.P.; Fumagalli, F.; Benevenuto, C.G.; da Silva Emery, F.; Gaspar, L.R. Novel benzophenone-3 derivatives with promising potential as UV filters: Relationship between structure, photoprotective potential and phototoxicity. Eur. J. Pharm. Sci. 2017, 101, 200–210. [Google Scholar] [CrossRef]
- Gonçalves, M.C.; Rossoni, J.V., Jr.; Rabelo, A.C.S.; Costa, D.C.; Cazati, T.; Taylor, J.G.; Dos Santos, V.M.R. Preliminary studies of the cytotoxicity and photoprotective properties of benzophenone and lactone derivatives. Rev. Virtual Quim. 2018, 10, 600–608. [Google Scholar] [CrossRef]
- Abbas, N.; Mahmood, Z.; Manzoor, S.; Akhtar, Z.; Tariq, M. Synthesis, characterization and sunscreen protection factor of novel synthesized Para Amino Benzoic Acid (PABA) esters. J. Chem. Soc. Pak. 2019, 41, 701–706. [Google Scholar]
- Bino, A.; Baldisserotto, A.; Scalambra, E.; Dissette, V.; Vedaldi, D.E.; Salvador, A.; Durini, E.; Manfredini, S.; Vertuani, S. Design, synthesis and biological evaluation of novel hydroxy-phenyl-1H-benzimidazoles as radical scavengers and UV-protective agents. J. Enzyme Inhib. Med. Chem. 2017, 32, 527–537. [Google Scholar] [CrossRef] [PubMed]
- Djuidje, E.N.; Durini, E.; Sciabica, S.; Serra, E.; Balzarini, J.; Liekens, S.; Manfredini, S.; Vertuani, S.; Baldisserotto, A. Skin damages—Structure activity relationship of benzimidazole derivatives bearing a 5-membered ring system. Molecules 2020, 25, 4324. [Google Scholar] [CrossRef] [PubMed]
- Popiół, J.; Gunia-Krzyzak, A.; Piska, K.; Zelaszczyk, D.; Koczurkiewicz, P.; Słoczynska, K.; Wójcik-Pszczoła, K.; Krupa, A.; Kryczyk-Poprawa, A.; Zesławska, E.; et al. Discovery of novel UV-filters with favorable safety profiles in the 5-arylideneimidazolidine-2,4-dione derivatives group. Molecules 2019, 24, 2321. [Google Scholar] [CrossRef]
- Peyrot, C.; Mention, M.M.; Brunissen, F.; Allais, F. Sinapic acid esters: Octinoxate substitutes combining suitable uv protection and antioxidant activity. Antioxidants 2020, 9, 782. [Google Scholar] [CrossRef] [PubMed]
- Reis, J.S.; Corrêa, M.A.; Chung, M.C.; Dos Santos, J.L. Synthesis, antioxidant and photoprotection activities of hybrid derivatives useful to prevent skin cancer. Bioorg. Med. Chem. 2014, 22, 2733–2738. [Google Scholar] [CrossRef] [PubMed]
- Polonini, H.C.; Lima, L.L.; Gonçalves, K.M.; Do Carmo, A.M.R.; Da Silva, A.D.; Raposo, N.R.B. Photoprotective activity of resveratrol analogues. Bioorg. Med. Chem. 2013, 21, 964–968. [Google Scholar] [CrossRef]
- Horbury, M.D.; Holt, E.L.; Mouterde, L.M.M.; Balaguer, P.; Cebrian, J.; Blasco, L.; Allais, F.; Stavros, V.G. Towards symmetry driven and nature inspired UV filter design. Nat. Commun. 2019, 10, 4748. [Google Scholar] [CrossRef]
- Rioux, B.; Peyrot, C.; Mention, M.M.; Brunissen, F.; Allais, F. Sustainable synthesis of p-hydroxycinnamic diacids through proline-mediated knoevenagel condensation in Ethanol: An access to potent phenolic UV filters and radical scavengers. Antioxidants 2020, 9, 331. [Google Scholar] [CrossRef]
- Resende, D.I.S.P.; Almeida, M.C.; Maciel, B.; Carmo, H.; Lobo, J.S.; Pozzo, C.D.; Cravo, S.M.; Rosa, G.P.; Kane-Pagès, A.; Do Carmo Barreto, M.; et al. Efficacy, stability, and safety evaluation of new polyphenolic xanthones towards identification of bioactive compounds to fight skin photoaging. Molecules 2020, 25, 2782. [Google Scholar] [CrossRef]
- Polonini, H.C.; Dias, R.M.P.; Souza, I.O.; Gonçalves, K.M.; Gomes, T.B.B.; Raposo, N.R.B.; Da Silva, A.D. Quinolines derivatives as novel sunscreening agents. Bioorg. Med. Chem. Lett. 2013, 23, 4506–4510. [Google Scholar] [CrossRef] [PubMed]
- Giacomo, B.; Luca, B.; Nicola, L.; Luigi, R. Development of an innovative and eco-friendly UV radiation absorber, based on furan moieties. Cosmetics 2020, 7, 6. [Google Scholar] [CrossRef]
- Peyrot, C.; Mention, M.M.; Brunissen, F.; Balaguer, P.; Allais, F. Innovative bio-based organic UV-A and blue light filters from Meldrum’s acid. Molecules 2020, 25, 2178. [Google Scholar] [CrossRef] [PubMed]