Functional and nutraceutical foods provide an alternative way to improve immune function to aid in the management of various diseases. With the development of research into nutraceuticals, dietary polyphenols are getting attention due to their immunomodulatory role. There is evidence that dietary polyphenols can influence dendritic cells, have an immunomodulatory effect on macrophages, increase proliferation of B cells, T cells, and suppress Type 1 T helper (Th1), Th2, Th17, and Th9 cells. Further, polyphenols have a beneficial role in the prevention and treatment of inflammatory bowel disease, allergy, asthma, and auto-immune diseases (type 1 diabetes, rheumatoid arthritis, and multiple sclerosis).
1. Introduction
With advancing knowledge of the importance of adequate nutrition, and increased public health awareness about diet, there is growing attention on the health benefits of natural products including those that are rich in polyphenols. Polyphenols are the most extensive group of non-energetic secondary metabolites and are produced by plants in response to stress [1] (Figure 1). Polyphenols have been called ‘lifespan essentials’ due to their significant impact on health [2]. There are as many as 8000 different polyphenols which are divided into different classes based on their chemical structure. Despite the different classifications, all polyphenols have the key structural features of an aromatic ring and at least one hydroxyl group [3,4]. Dietary polyphenols are abundant in plant-based foods such as fruits, vegetables, dry legumes, cereals, olives, cocoa, tea, coffee and wine [5]. Some common dietary polyphenols include the lignins present in nuts and whole-grain cereals; pro-anthocyanidins in grapes, pine bark and cocoa; anthocyanins/anthocyanidins in brightly colored fruits and vegetables like berries; isoflavones in soybeans; catechins in green tea, grapes and wine; tannins in tea and nuts; quercetin in grapes and onion; resveratrol in wines and naringenin/hesperidin in citrus fruits [6].
Research into the beneficial health effects of polyphenols has increased considerably over the last two decades [7]. Polyphenols have shown anti-inflammatory, antimicrobial, antioxidant, anticarcinogenic, antiadipogenic, antidiabetic and neuroprotective effects [8–12]. Polyphenols may also counteract cytotoxicity and apoptosis due to their immunomodulatory properties [13] and regulate innate and adaptive immunity. Polyphenols have also been shown to reduce oxidative stress and inflammation [14], modulate immune cells, regulate gut microbiota composition and immunity (Figure 1). Through this regulation of the immune system, polyphenols could beneficially impact a number of chronic diseases [15].
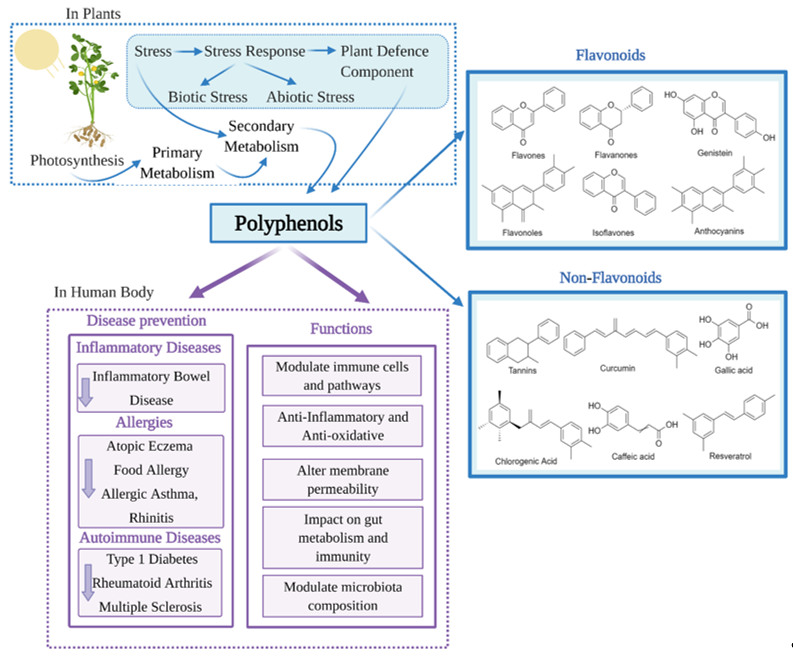
Figure 1. Classification and health benefits of polyphenols.
2. Immune Modulation of Polyphenols to Immune Cells
The immune system as a whole consists of innate and adaptive immunity, each with different roles and functions [16]. The innate immune system is the first line of defense, and protects against foreign antigens through the skin, pulmonary system, and gut epithelial cells, forming a barrier between the organism and its environment [17]. The innate system is broadly divided into cellular and non-cellular systems. The cellular system consists of several cell subsets, including dendritic cells (DCs), monocytes, macrophages, granulocytes and natural killer (NK) cells. The non-cellular system is very diverse, ranging from simple mucus barriers to complex protein pathways, such as the complement cascade, however all function to prevent pathogen entry, and facilitate pathogen destruction by phagocytosis [18]. The adaptive immune system comprises T and B cells. B cells secrete antibodies, whilst T cells are involved in the production of cytokines, direct cytotoxic destruction of infected or malignant tissue, and activation of other immune cells [16]. Polyphenols modulate immune responses in both the innate and adaptive systems, having both stimulatory and inhibitory effects in different areas [19] (Figure 2).
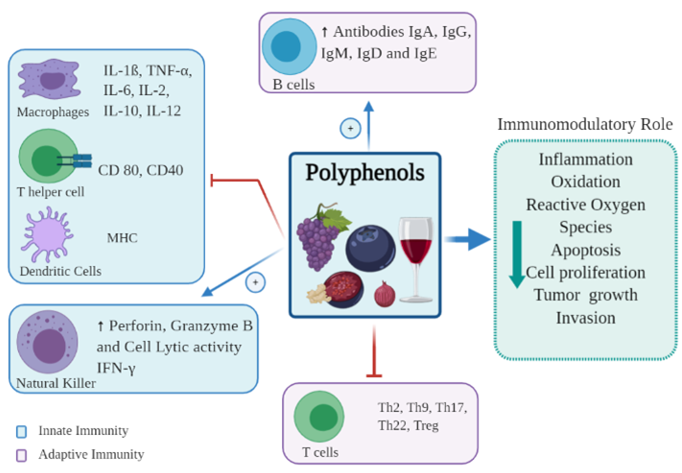
Figure 2. Immunomodulatory effects of polyphenols on immune cells.
2.1. Effects of Polyphenols on Dendritic Cells
DCs are the most potent antigen-presenting cells which act to prime the adaptive immune system to recognize foreign antigens, and so are vital in the initiation and regulation of the adaptive immune response [20]. It has been shown that polyphenols can influence the differentiation of DCs [21]. In fact, resveratrol has been identified as affecting human DC differentiation from monocytes, with a strong potential for regulatory action [22]. Likewise, epigallocatechin gallate (EGCG) induces apoptosis and affects the phenotype of developing DCs. Molecules that are essential for antigen presentation by DCs such as CD83, CD80, CD11c, and major histocompatibility complex (MHC) class II, are downregulated by EGCG, suggesting an immunosuppressive action [23]. Other polyphenols, including EGCG, curcumin, quercetin, apigenin, silibinin, and blackberry polyphenols cause inhibition of murine bone marrow-derived DC maturation and expression of MHC molecules, reducing antigen uptake and decreasing secretion of the pro-inflammatory cytokines interleukin-1 (IL-1), IL-2, IL-6, IL-12 [24–27]. A study in an animal model showed that administration of fisetin (50 mg/kg) decreased DC migration and DC allo-stimulatory capacity [28]. Similarly, in vitro resveratrol has an inhibitory effect on DC maturation [29].
2.2. Effects of Polyphenols on Monocytes and Macrophages
Macrophages are phagocytes that ingest pathogens and dead cells, which differentiate from the transitory monocyte. Like DCs, macrophages can also function as antigen-presenting cells (albeit with less potent activity) being able to activate naïve T cells into effector T cells in the presence of an antigen [19]. Macrophages play an important role in inflammation, host defense, and tissue repair [30,31]. Importantly, macrophages also play a pathogenic role in various chronic diseases including asthma, inflammatory bowel disease, atherosclerosis and rheumatoid arthritis [31–33]. Macrophages are classically considered in two categories, known as polarization: the classical inflammatory M1 and immunosuppressive/anabolic M2 phenotypes. Initiation of M1 differentiation is by interferon-γ (IFN-γ) stimulation and the activation of toll-like receptors (TLRs) by bacterial lipopolysaccharides (LPS); while M2 polarization is triggered by IL-4 [34]. It has been shown that polyphenolic cocoa extract suppressed M1 mediated inflammation and drove M2 polarization of activated macrophages [35]. Polyphenol-rich green tea has anti-tumor effects secondary to the activation of macrophages and NK cells [36]. Inonotus sanghuang, a plant known for its medicinal value, rich in rutin, quercetin, quercitrin, isorhamnetin and chlorogenic acid, has been shown to reduce inflammation by modulating the interaction between macrophages and adipocytes. It was suggested that in this way it may improve insulin resistance and metabolic syndrome [37]. Moreover, Overman et al. reported that grape powder extract decreased LPS-stimulated inflammation in macrophages and reduce insulin resistance [38].
Monocytes and macrophages play a fundamental role in the progression of atherosclerosis [35]. Increased oxidative stress causes low-density lipoprotein oxidation (oxLDL), with the resulting lipoproteins engulfed by macrophages resulting in the formation of foam cells. This process triggers an inflammatory response in the neighboring endothelial cells which secrete pro-inflammatory cytokines and chemokines [39–41]. When monocytes migrate towards the intima, they transform into macrophages on stimulation by macrophage colony-stimulating factor, increasing the expression of scavenger receptors outside the cell [39,40]. Polyphenols are known to regulate this interplay between immune and vascular endothelial function. Evidence has shown that polyphenols reduce atherosclerotic progression by increasing high-density lipoprotein (HDL) levels and decrease LDL accumulation in macrophages, reducing foam cell formation [3,42].
2.3. Effects of Polyphenols on Natural Killer Cells
NK cells are a subset of lymphocytes, but are part of the innate immune response, with the function of eliminating infected or malignant cells [19]. NK cells have a strong cytolytic function and a considerable role in immune regulation [43]. NK cells are activated by CD4+ T cell secretion of IL-2 and IFN-γ [44]. Once activated NK cells secrete perforin and granzyme B, which induce apoptosis and necrosis in target cells. Polyphenols have immunomodulatory effects on NK cells, increasing their number and activity. Green tea catechin metabolites increase NK cell cytotoxicity [45] and quercetin enhances NK cell lytic activity [46] in animal models. In a clinical trial, a healthy participants prescribed a diet low in polyphenols and supplemented with juices rich in polyphenols increased lymphocyte proliferative responsiveness, IL-2 secretion and lytic activity by NK cells [47]. Berries rich in flavonoids and pro-anthocyanidins have a cancer-preventive effect but are also involved in the modulation of NK cells [48]. A study in marathon runners noted that daily consumption of 250 g of blueberries for six weeks resulted in doubled NK cell counts [49]. Evidence showed that purple sweet potato leaves that are rich in flavonoids enhanced the lytic activity of NK cells in 16 healthy participants [50].
References
- Swallah, M.S.; Sun, H.; Affoh, R.; Fu, H.; Yu, H. Antioxidant potential overviews of secondary metabolites (polyphenols) in fruits. International Journal of Food Science 2020, 2020.
- Chandrasekara, A.; Shahidi, F. Content of insoluble bound phenolics in millets and their contribution to antioxidant capacity. Journal of agricultural and food chemistry 2010, 58, 6706-6714.
- Santhakumar, A.B.; Battino, M.; Alvarez-Suarez, J.M. Dietary polyphenols: Structures, bioavailability and protective effects against atherosclerosis. Food and Chemical Toxicology 2018, 113, 49-65.
- Yamagata, K.; Tagami, M.; Yamori, Y. Dietary polyphenols regulate endothelial function and prevent cardiovascular disease. Nutrition 2015, 31, 28-37.
- Cardona, F.; Andrés-Lacueva, C.; Tulipani, S.; Tinahones, F.J.; Queipo-Ortuño, M.I. Benefits of polyphenols on gut microbiota and implications in human health. The Journal of nutritional biochemistry 2013, 24, 1415-1422.
- Martin, K.R.; Appel, C.L. Polyphenols as dietary supplements: A double-edged sword. Nutrition and Dietary Supplements 2009, 2, 1-12.
- Quiñones, M.; Miguel, M.; Aleixandre, A. Beneficial effects of polyphenols on cardiovascular disease. Pharmacological research 2013, 68, 125-131.
- Cassidy, A.; O’Reilly, É.J.; Kay, C.; Sampson, L.; Franz, M.; Forman, J.P.; Curhan, G.; Rimm, E.B. Habitual intake of flavonoid subclasses and incident hypertension in adults. The American journal of clinical nutrition 2011, 93, 338-347.
- Chiva-Blanch, G.; Urpi-Sarda, M.; Ros, E.; Valderas-Martinez, P.; Casas, R.; Arranz, S.; Guillén, M.; Lamuela-Raventós, R.M.; Llorach, R.; Andres-Lacueva, C. Effects of red wine polyphenols and alcohol on glucose metabolism and the lipid profile: a randomized clinical trial. Clinical nutrition 2013, 32, 200-206.
- Hanhineva, K.; Törrönen, R.; Bondia-Pons, I.; Pekkinen, J.; Kolehmainen, M.; Mykkänen, H.; Poutanen, K. Impact of dietary polyphenols on carbohydrate metabolism. International journal of molecular sciences 2010, 11, 1365-1402.
- Hooper, L.; Kay, C.; Abdelhamid, A.; Kroon, P.A.; Cohn, J.S.; Rimm, E.B.; Cassidy, A. Effects of chocolate, cocoa, and flavan-3-ols on cardiovascular health: a systematic review and meta-analysis of randomized trials. The American journal of clinical nutrition 2012, 95, 740-751.
- Ahtesh, F.B.; Stojanovska, L.; Feehan, J.; de Courten, M.P.; Flavel, M.; Kitchen, B.; Apostolopoulos, V. POLYPHENOL RICH SUGAR CANE EXTRACT INHIBITS BACTERIAL GROWTH.
- Scalbert, A.; Manach, C.; Morand, C.; Rémésy, C.; Jiménez, L. Dietary polyphenols and the prevention of diseases. Critical reviews in food science and nutrition 2005, 45, 287-306.
- Hussain, T.; Tan, B.; Yin, Y.; Blachier, F.; Tossou, M.C.B.; Rahu, N. Oxidative stress and inflammation: what polyphenols can do for us? Oxidative medicine and cellular longevity 2016, 2016.
- Wolowczuk, I.; Verwaerde, C.; Viltart, O.; Delanoye, A.; Delacre, M.; Pot, B.; Grangette, C. Feeding our immune system: impact on metabolism. Clinical and Developmental Immunology 2008, 2008.
- Medzhitov, R.; Janeway, C. Innate immunity. New England Journal of Medicine 2000, 343, 338-344.
- Beutler, B. Innate immunity: an overview. Molecular immunology 2004, 40, 845-859.
- Clark, R.; Kupper, T. Old meets new: the interaction between innate and adaptive immunity. Journal of Investigative Dermatology 2005, 125, 629-637.
- Hachimura, S.; Totsuka, M.; Hosono, A. Immunomodulation by food: impact on gut immunity and immune cell function. Bioscience, biotechnology, and biochemistry 2018, 82, 584-599.
- Buckwalter, M.R.; Albert, M.L. Orchestration of the immune response by dendritic cells. Current Biology 2009, 19, R355-R361.
- del Cornò, M.; Scazzocchio, B.; Masella, R.; Gessani, S. Regulation of dendritic cell function by dietary polyphenols. Critical reviews in food science and nutrition 2016, 56, 737-747.
- Švajger, U.; Obermajer, N.; Jeras, M. Dendritic cells treated with resveratrol during differentiation from monocytes gain substantial tolerogenic properties upon activation. Immunology 2010, 129, 525-535.
- Yoneyama, S.; Kawai, K.; Tsuno, N.H.; Okaji, Y.; Asakage, M.; Tsuchiya, T.; Yamada, J.; Sunami, E.; Osada, T.; Kitayama, J. Epigallocatechin gallate affects human dendritic cell differentiation and maturation. Journal of allergy and clinical immunology 2008, 121, 209-214.
- Lee, J.S.; Kim, S.G.; Kim, H.K.; Lee, T.H.; Jeong, Y.I.; Lee, C.M.; Yoon, M.S.; Na, Y.J.; Suh, D.S.; Park, N.C. Silibinin polarizes Th1/Th2 immune responses through the inhibition of immunostimulatory function of dendritic cells. Journal of cellular physiology 2007, 210, 385-397.
- Huang, R.-Y.; Yu, Y.-L.; Cheng, W.-C.; OuYang, C.-N.; Fu, E.; Chu, C.-L. Immunosuppressive effect of quercetin on dendritic cell activation and function. The Journal of Immunology 2010, 184, 6815-6821.
- Yoon, M.-S.; Lee, J.S.; Choi, B.-M.; Jeong, Y.-I.; Lee, C.-M.; Park, J.-H.; Moon, Y.; Sung, S.-C.; Lee, S.K.; Chang, Y.H. Apigenin inhibits immunostimulatory function of dendritic cells: Implication of immunotherapeutic adjuvant. Molecular pharmacology 2006, 70, 1033-1044.
- Gupta, S.C.; Tyagi, A.K.; Deshmukh-Taskar, P.; Hinojosa, M.; Prasad, S.; Aggarwal, B.B. Downregulation of tumor necrosis factor and other proinflammatory biomarkers by polyphenols. Archives of Biochemistry and Biophysics 2014, 559, 91-99.
- Liu, S.-H.; Lin, C.-H.; Hung, S.-K.; Chou, J.-H.; Chi, C.-W.; Fu, S.-L. Fisetin inhibits lipopolysaccharide-induced macrophage activation and dendritic cell maturation. Journal of agricultural and food chemistry 2010, 58, 10831-10839.
- Buttari, B.; Profumo, E.; Facchiano, F.; Ozturk, E.I.; Segoni, L.; Saso, L.; Riganò, R. Resveratrol prevents dendritic cell maturation in response to advanced glycation end products. Oxidative medicine and cellular longevity 2013, 2013.
- Bottazzi, B.; Doni, A.; Garlanda, C.; Mantovani, A. An integrated view of humoral innate immunity: pentraxins as a paradigm. Annual review of immunology 2009, 28, 157-183.
- Murray, P.J.; Wynn, T.A. Protective and pathogenic functions of macrophage subsets. Nature reviews immunology 2011, 11, 723-737.
- Hansson, G.K.; Hermansson, A. The immune system in atherosclerosis. Nature immunology 2011, 12, 204.
- Kamada, N.; Hisamatsu, T.; Okamoto, S.; Chinen, H.; Kobayashi, T.; Sato, T.; Sakuraba, A.; Kitazume, M.T.; Sugita, A.; Koganei, K. Unique CD14+ intestinal macrophages contribute to the pathogenesis of Crohn disease via IL-23/IFN-γ axis. The Journal of clinical investigation 2008, 118, 2269-2280.
- Sica, A.; Mantovani, A. Macrophage plasticity and polarization: in vivo veritas. The Journal of clinical investigation 2012, 122, 787-795.
- Dugo, L.; Belluomo, M.G.; Fanali, C.; Russo, M.; Cacciola, F.; Maccarrone, M.; Sardanelli, A.M. Effect of cocoa polyphenolic extract on macrophage polarization from proinflammatory M1 to anti-inflammatory M2 state. Oxidative medicine and cellular longevity 2017, 2017.
- Park, H.-R.; Hwang, D.; Suh, H.-J.; Yu, K.-W.; Kim, T.Y.; Shin, K.-S. Antitumor and antimetastatic activities of rhamnogalacturonan-II-type polysaccharide isolated from mature leaves of green tea via activation of macrophages and natural killer cells. International journal of biological macromolecules 2017, 99, 179-186.
- Zhang, M.; Xie, Y.; Su, X.; Liu, K.; Zhang, Y.; Pang, W.; Wang, J. Inonotus sanghuang polyphenols attenuate inflammatory response via modulating the crosstalk between macrophages and adipocytes. Frontiers in immunology 2019, 10, 286.
- Overman, A.; Bumrungpert, A.; Kennedy, A.; Martinez, K.; Chuang, C.C.; West, T.; Dawson, B.; Jia, W.; McIntosh, M. Polyphenol-rich grape powder extract (GPE) attenuates inflammation in human macrophages and in human adipocytes exposed to macrophage-conditioned media. International Journal of Obesity 2010, 34, 800-808.
- McLaren, J.E.; Michael, D.R.; Ashlin, T.G.; Ramji, D.P. Cytokines, macrophage lipid metabolism and foam cells: implications for cardiovascular disease therapy. Progress in lipid research 2011, 50, 331-347.
- Moss, J.W.E.; Ramji, D.P. Nutraceutical therapies for atherosclerosis. Nature Reviews Cardiology 2016, 13, 513.
- Ramji, D.P.; Davies, T.S. Cytokines in atherosclerosis: Key players in all stages of disease and promising therapeutic targets. Cytokine & growth factor reviews 2015, 26, 673-685.
- Sevov, M.; Elfineh, L.; Cavelier, L.B. Resveratrol regulates the expression of LXR-α in human macrophages. Biochemical and biophysical research communications 2006, 348, 1047-1054.
- Ruggeri, L.; Capanni, M.; Urbani, E.; Perruccio, K.; Shlomchik, W.D.; Tosti, A.; Posati, S.; Rogaia, D.; Frassoni, F.; Aversa, F. Effectiveness of donor natural killer cell alloreactivity in mismatched hematopoietic transplants. Science 2002, 295, 2097-2100.
- Hu, J.-Y.; Zhang, J.; Cui, J.-L.; Liang, X.-Y.; Lu, R.; Du, G.-F.; Xu, X.-Y.; Zhou, G. Increasing CCL5/CCR5 on CD4+ T cells in peripheral blood of oral lichen planus. Cytokine 2013, 62, 141-145.
- Kim, Y.H.; Won, Y.-S.; Yang, X.; Kumazoe, M.; Yamashita, S.; Hara, A.; Takagaki, A.; Goto, K.; Nanjo, F.; Tachibana, H. Green tea catechin metabolites exert immunoregulatory effects on CD4+ T cell and natural killer cell activities. Journal of agricultural and food chemistry 2016, 64, 3591-3597.
- Exon, J.H.; Magnuson, B.A.; South, E.H.; Hendrix, K. Dietary quercetin, immune functions and colonic carcinogenesis in rats. Immunopharmacology and immunotoxicology 1998, 20, 173-190.
- Bub, A.; Watzl, B.; Blockhaus, M.; Briviba, K.; Liegibel, U.; Müller, H.; Pool-Zobel, B.L.; Rechkemmer, G. Fruit juice consumption modulates antioxidative status, immune status and DNA damage. The Journal of nutritional biochemistry 2003, 14, 90-98.
- McAnulty, L.S.; Nieman, D.C.; Dumke, C.L.; Shooter, L.A.; Henson, D.A.; Utter, A.C.; Milne, G.; McAnulty, S.R. Effect of blueberry ingestion on natural killer cell counts, oxidative stress, and inflammation prior to and after 2.5 h of running. Applied Physiology, Nutrition, and Metabolism 2011, 36, 976-984.
- McAnulty, L.S.; Collier, S.R.; Landram, M.J.; Whittaker, D.S.; Isaacs, S.E.; Klemka, J.M.; Cheek, S.L.; Arms, J.C.; McAnulty, S.R. Six weeks daily ingestion of whole blueberry powder increases natural killer cell counts and reduces arterial stiffness in sedentary males and females. Nutrition research 2014, 34, 577-584.
- Chen, C.-M.; Li, S.-C.; Lin, Y.-L.; Hsu, C.-Y.; Shieh, M.-J.; Liu, J.-F. Consumption of purple sweet potato leaves modulates human immune response: T-lymphocyte functions, lytic activity of natural killer cell and antibody production. World Journal of Gastroenterology: WJG 2005, 11, 5777.
This entry is adapted from the peer-reviewed paper 10.3390/nu13030728