2. Preparation of Mono/Hybrid Nanofluids
Nanofluids are organized according to their preparation using one- or two-step methods (
Figure 12). In a one-step approach, nanoparticles are prepared and mixed directly in a base fluid using physical or chemical processes. In the two-step method, nanoparticles are obtained using physical or chemical methods and then effectively infused in an essential base liquid
[25][33]. Several investigators have reviewed the preparation of different mono/hybrid nanofluids based on various base fluids
[26][27][28][29][30][31][32][33][34][35][36][34,35,36,37,38,39,40,41,42,43,44]. Spherical ZnO particles were synthesized using a sol–gel annealing process at 500–600 °C in
[37][45]. The ball milling process was used to grind aluminum nitride carbon nanocomposite (a nontoxic ceramic) for heat transfer experiments
[38][46]. Making nanofluids through a single-step method is expensive and time-consuming. The control of particle agglomeration is the primary problem in the two-step method. Ultrasonication minimizes nanoparticle sedimentation and improves nanofluid stability
[39][47]. Due to simplicity, 95% of researchers used a two-step method when preparing nanofluids (see
Table 1).
Figure 12. Flowchart for producing hybrid nanofluids [40]. Flowchart for producing hybrid nanofluids [48].
Table 1.
Examples of hybrid nanofluids adopting two-step preparation.
107].
Ultrasonic mills, baths, stirrers, and high-pressure homogenizers are used for the dispersion of nanoparticles. Baby and Sundara
[51][59] used an economical method to synthesize hydrogen-functionalized, exfoliation-induced silver-decorated graphene (Ag/HEG) and prepared nanofluids. Ag/HEG was distributed in a mixture of deionized water/ethylene glycol using ultrasonic agitation without surfactant. The hybrid nanofluid was observed to be stable for more than 3 months. Aravind and Ramaprabhu
[54][62] prepared MWCNT nanocomposites with graphene shells and synthesized them by chemical vapor deposition. The prepared hybrid nanofluid was stable for an extended period. Megatif et al.
[72][80] prepared a CNT–TiO
2 hybrid nanocomposite and dispersed it in water to obtain a hybrid nanofluid. The surfactant SDBS was added to the suspension for proper dispersion. They sonicated the solution for 15 min and tested its stability. The solution was stable for 2 days.
Although most (95%) of the researchers adhered to the two-step method, nanofluids synthesized by the expensive and complex one-step method improve the stability of nanoparticle suspensions in base oils due to high sedimentation rates with short sonication times
[100][108]. Ultrasonication lessens the sedimentation of nanoparticles, resulting in enhanced nanofluid stability. A better understanding of the mechanisms of nanofluids at the atomic level is required to address particle transport, aggregation, and stability issues with minimal experimentation.
No sophisticated equipment is required to produce nanofluids using a simple two-step method. Dispersion of nanoparticles requires sonication times of 3–10 h
[101][109]. Amin et al.
[102][110] critically reviewed the properties of single and hybrid nanofluids based on organic and synthetic materials. Malika and Sonavan
[103][111] used a two-step method to prepare CuO–ZnO/water hybrid nanofluids. Ultrasonication provided nanofluid stability. FESEM/EDS, dynamic light scattering, and zeta potential measurements provide insight into nanoparticle morphology, shape, and size. The stability of Al
2O
3–CuO/(50/50) EG/W (ethylene glycol/water) hybrid nanofluids at 60 °C was confirmed by zeta potential measurements
[104][112].
The stability of trihybrid nanofluids was tested by mixing three types of nanoparticles (i.e., Al
2O
3, TiO
2, and SiO
2 with volume concentrations of 0.05–0.3%) in a water/ethylene glycol-based fluid
[105][113] and a recommended sonication time of 10 h at a zeta potential of 25.1 mV. To improve the stability of nanofluids, Afshari et al.
[106][114] highlighted properties such as the acidity degree of the nanofluid, ultrasonication, nanoparticle material, base fluid type, nanoparticle concentration, surfactants, and surface modification of nanoparticles. Arora and Gupta
[107][115] reviewed stability evaluation techniques (spectral absorbance, sedimentation, zeta-potential, and electron microscopy) and enhancement techniques (ultrasonication, surfactant addition, particle surface modifications, and pH change). Future research should focus on industrial applications to minimize pressure losses, the concentration of nanoparticles, and the long-term stability of hybrid nanofluids.
The stability characteristics of mono and hybrid nanofluids have been studied using zeta potential measurements and vibrating sample magnetometry (VSM) analysis
[108][116]. To maintain nanofluid stability, Zainon and Azmi
[23][29] recommend analysis by sonication, pH modification, surfactant, TEM, field emission scanning electron microscopy (FESEM), XRD, zeta potential, and UV/visible spectroscopy techniques. Bumataria et al.
[109][117] used single and hybrid nanofluids to study heat transfer consider in heat pipe technologies. The use of dispersing agents and sonication increases the stability of nanofluids
[110][118]. Excellent suspension stability could be obtained by adding small amounts of SDBS and PEG to DW (hybrid nanofluid 25% Al
2O
3 + 75% TiO
2)
[111][119]. The hybrid nanofluid’s stability was high, as the zeta potential value (i.e., the electrostatic repulsive force between the nanoparticle and the base fluid) was 42.6 mV compared to the reference value of 30 mV. Said et al.
[112][120] investigated the stability of carbon nanofibers (CNF), functionalized carbon nanofibers (F-CNF), reduced graphene oxide (rGO), and F-CNF/rGO nanofluids. Hybrid nanofluids (FCNF/rGO) showed higher stability than CNF, F-CNF, and rGO nanofluids.
Muthoka et al.
[113][121] investigated the stability of hybrid nanofluids with two nanoparticles in PCM/DI water. The stability of surfactant-free MgO and 24 wt.% primary liquid was poor after 24 h, whereas the functionalized MWCNT solution showed no separation after 24 h. It was confirmed that the nanofluid’s low-temperature stability was increased using a surfactant. Acid treatment with CNF was used to test stability
[114][122]. The zeta potential of 0.02 vol.% F–CNF nanofluids measured after 2 and 90 days was −42.9 and −41.8 mV, indicating improved stability compared to the −16.3 and −15.5 mV UNV zeta potentials, which were characterized by relatively unstable dispersion. Alawi et al.
[115][123] synthesized aqueous nanofluids PEG–GnP, PEG–TGr, Al
2O
3, and SiO
2. The dispersion stability of the carbon-based nanofluid and the metal oxide nanofluid was observed for 30 days, and the high dispersibility of PEG–HNP and PEG–TGr in an aqueous medium with low sedimentation was confirmed. Compared to GnP/DW nanofluids, TiO
2/DW nanofluids showed superior stability
[116][124]. The addition of CTAB surfactant showed excellent stability of ternary hybrid nanofluids
[117][125]. Uysal
[118][126] used a 500 rpm homogenizer to mix and stabilize nano-graphene in vegetable oil. Al-Waeli et al.
[119][127] demonstrated high nanofluid stability (over 80 days) with CTAB and tannic acid + ammonia solution. The stability of Al
2O
3/water nanofluids using CTAB and SDBS surfactants was investigated for various pH values
[120][128]. Kazemi et al.
[121][129] visually observed the stability of SiO
2/water and G/water nanofluids. SiO
2/water nanofluids were found stable at all pH values (see
Table 2 for the stability of various nanofluids).
Table 2.
Stability of different nanofluids with surfactants.
Jana et al.
[41][49] infused various volume fractions of CNTs in water to obtain CNT suspensions. Au nanoparticles were suspended with CNT in varying volume fractions to obtain CNT–Au suspensions. The hybrid suspension was sonicated for 1 h using an ultrasonic cleaner to get an adequately dispersed solution. Bhosale and Borse
[55][63] prepared a hybrid nanofluid (Al
2O
3–CuO water) by mixing 2.5 mg of CuO and Al
2O
3 in distilled water. Later, the concentration was varied to 0.25%, 0.5%, and 1.0% volume. Toghraie et al.
[74][82] prepared ZnO–TiO
2/EG hybrid nanofluids by dispersing equal volumes of ZnO and titanium dioxide (TiO
2) nanoparticles in a given amount of pure EG as a base liquid. The stability of the prepared nanofluid was confirmed, ensuring no sedimentation. Paul et al.
[47][55] synthesized Al–Zn nanoparticles by stirring. They prepared hybrid nanofluids through a two-step process. Al–Zn nanoparticles were added to ethylene glycol (base fluid), followed by sonication and magnetic stirring. Suresh et al.
[48][56] obtained a hybrid powder of alumina–copper using a thermochemical method, including spray-drying, oxidation of the precursor powder, hydrogen reduction, and homogenization. They used different volume fractions (0.1%, 0.33%, 0.75%, 1.0%, and 2.0%). Baby and Sundara
[46][54] used a hydrogen-induced exfoliation and chemical reduction process of graphite oxide (GO) to synthesize grapheme decorated with CuO (CuO/HEG). The HEG obtained was functionalized by acid treatment and coated with CuO nanoparticles. CuO/HEG was dispersed in the base liquid (water/EG) by ultrasonication. Nine et al.
[58][66] reported an economical and beneficial process for synthesizing Cu
2O and Cu/Cu
2O nanoparticles with a mean size of less than 30 nm. A ball milling process was used to synthesize Cu/Cu
2O–water hybrid nanofluids. Madhesh et al.
[63][71] prepared a copper–titania hybrid nanofluid by uniformly dispersing an aqueous solution of titania (5 g) and copper acetate (0.5 g) in an ultrasonic vibrator for 2 h using reducing agents at 45 °C and atmospheric pressure. A one-step method was described for a hybrid nanofluid containing silver and silica nanoparticles by Botha et al.
[49][57]. Ho et al.
[50][58] prepared phase change material (PCM) suspensions using interfacial poly-condensation and emulsion techniques. Nanofluid Al
2O
3–water was obtained by adding Al
2O
3 nanoparticles in water (base liquid). Chen et al.
[53][61] prepared Ag/MWCNT nanocomposites using the silver mirror reaction. Functionalized MWCNTs were used to fabricate Ag/MWCNT nanocomposites using sodium dodecyl sulfate (SDS) as a surfactant and formaldehyde as a reducing agent.
3. Characterization and Stability of Mono/Hybrid Nanofluids
Several forces, such as van der Waals attraction, buoyancy, gravity, and electrostatic repulsion, cause destabilization and sediment formation. Van der Waals attraction and gravity decrease the stability of colloidal suspensions. Stability is a critical factor in the effectiveness of nanofluids for technological applications. All thermo-physical properties of nanofluids depend on their stability. The instability of nanofluids can reduce their effectiveness in many heat transfer applications. It is caused by the tendency of nanoparticles to form clusters in liquids. An SEM image of the Al
2O
3–MWCNT/water hybrid nanofluid is shown in
Figure 23 [93][101].
Figure 23.
SEM image of Al
2
O
3–MWCNT/water hybrid nanofluid [93]. –MWCNT/water hybrid nanofluid [101].
The particle aggregation causes the separation of nanoparticles from base fluids and forms sedimentation
[94][102]. The coagulation rate is determined from the collision frequency of particles in Brownian motion and cohesion probability
[95][103]. Removal of agglomeration propensity yields stable nanofluids. Methods adopted for assessing the stability of nanofluids are the sedimentation method, spectral absorbance, centrifugation method, transmittance measurement, zeta potential measurement, and dynamic light scattering
[96][104]. For long-term stable and homogenous nanofluids, the following surfactants can be added
[97][98][105,106]: anionic (sodium dodecyl sulfate and sodium dodecyl benzene sulfonate), cationic (cetyl trimethyl ammonium bromide), nonionic (Span-80 and Tween-20), and polymer (polyvinyl pyrrolidone, polyvinyl alcohol, and gum Arabic). Surfactants improve the wettability of the nanoparticles and the base fluids by reducing the base fluid’s surface tension and improving the nanoparticles’ dispersibility
[99][
Brownian motion of nanoparticles, micro-convection, clustering, and pH value strongly affect the thermal properties of hybrid nanofluids
[133][141]. Solidification and clustering of nanocomposites of different sizes in nanofluids affect their thermal properties
[134][135][142,143]. The stabilization and evaporation of single and hybrid nanofluids have been studied in specific systems from a statistical point of view
[136][137]. Bhattad et al. [138] investigated with Al2O3 - TiO2 - water hybrid solution. Commercially purchased nanoparticles were mixed with the primary fluid (DI Water) using a mechanical stirrer followed by ultrasonication. Afterward[144,
the solution was characterized to verify the shape, size, proper mixing and stability145].
SEM (Scanning electron microscopy) and TEM (Transmission electron microscopy) tests were performed and measured the mean size of Al2O3 and TiO2 nanoparticles by ImageJ 2.0.0-rc-3 as 45 nm and 20 nm, respectively. The small-size particles in Figure 3 represent TiO2 nanoparticles, whereas larger ones are the Al2O3 nanoparticles. Both types of nanoparticles were found to be spherical, with a shape factor of 1. One of the key challenges in studying nanofluids is ensuring their stability and homogeneity. A stability test involving gravitational settling was performed to address this issue, and images of the test tube were taken at different intervals (Figure 4). The results showed that there was no sedimentation throughout the 7-day investigation.
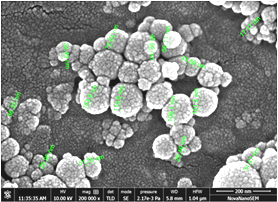
(a)
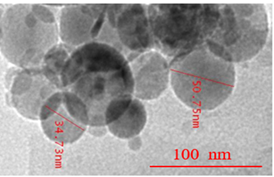
(b)
Figure 3. (a). SEM image of Al2O3- TiO2/water hybrid nanofluid; (b). TEM image of Al2O3- TiO2/water hybrid nanofluid.
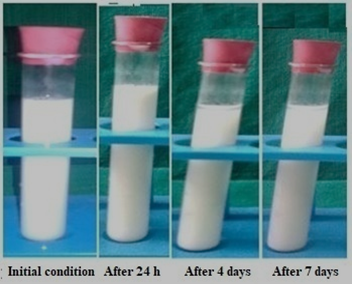
Figure 4. Stability analysis of a sample showing no sedimentation for 7 days.