E-cadherin, the major epithelial cadherin, is located in regions of cell–cell contact known as adherens junctions. E-cadherin contributes to the maintenance of the epithelial integrity through homophylic interaction; the cytoplasmic tail of E-cadherin directly binds catenins, forming a dynamic complex that regulates several intracellular signal transduction pathways, including epithelial-to-mesenchymal transition (EMT).
1. Introduction to Cadherins
Cadherins are transmembrane or membrane-associated glycoproteins that mediate Ca
2+-dependent cell–cell adhesion and have mainly been described for their instrumental role during morphogenesis
[1]. Cadherins’ functions extend to multiple aspects of morphogenesis, ranging from polarization of simple epithelia to the formation of tissues and organs architecture, the conference of resistance to detachment and the control of cellular tissue organization and cohesion
[1]. Cadherins expression occurs through a dynamic process and is regulated by a great number of developmental factors and cellular signals. From the analysis of the sequence similarity, cadherins were divided into five subfamilies: classical types I and II (E-, P-, N- and VE-cadherin), atypical (T-cadherin), desmosomal (desmogleins, desmocollins), protocadherins and cadherin-related proteins
[2]. The family of classical cadherins includes E (epithelial)-, N (neural)-, P (placental)-, VE (vascular-endothelial)-, R (retinal)- and K (kidney)-cadherins; among these, E-cadherin is essential for the formation of adherens junctions (AJs) in epithelial cells. E-cadherin mediates strong, homotypic adhesion between neighboring epithelial cells, thereby, safeguarding epithelial barrier integrity
[2][3][2,3]. The lack of a functional, tight junction and desmosome formation in the absence of E-cadherin emphasizes its central role in the regulation of epithelial cell–cell contacts
[2].
2. E-Cadherin Discovery
E-cadherin, a type-I cadherin, is generally considered the prototype of all cadherins because of its early identification and its thorough characterization both in normal and in pathological conditions. In 1977, Takeichi
[4] proposed the existence of a physiological Ca
2+-dependent cell–cell adhesion that could explain the adhesive properties of a lung cell line in addition to the more known modality of Ca
2+-independent agglutination. Takeichi discovered a surface protein of about 150 kDa involved in the Ca
2+-dependent cell–cell adhesion, reporting, for the first time, the E-cadherin adhesion potential. At the same time, other research groups investigated in this field, reaching results that, only later, were linked together. François Jacob’s group, in 1980, described a specific cell-surface glycoprotein named uvomorulin, the 84 kDa fragment of which was responsible for the Ca
2+-dependent compaction of mouse embryonal cells
[5]. By the use of antibodies against this 84 kDa fragment, cell–cell interactions were perturbated, and the compaction of embryos before implantation was prevented. Using experiments based on subsequent trypsinizations, the same research group deduced that a short-lived precursor was produced by cells from which a stable form of 120 kDa is derived; this 120 kDa protein, in presence of Ca
2+, was cleaved, giving rise to the 84 kDa active fragment. Electron microscopy revealed that uvomorulin was localized in the intermediate junctions or AJs of intestinal epithelial cells
[6], and, nowadays, it is established that the 84 kDa fragment corresponds to the ectodomain of E-cadherin. Concurrently, Wheelock’s group
[7] reported the identification and purification of a protein, expressed by epithelial cell lines and tissues, that was named cell-CAM 120/80. This identification was achieved using antibodies directed against an 80 kDa protein that was released into serum-free medium by MCF-7 human breast cancer cells
[7][8][7,8]. These antibodies caused disruption of cell–cell junctions in mouse epithelial cells and enabled characterization of the cell-surface form of the antigen as a glycoprotein of 120 kDa from which the 84 kDa fragment was released. Complementary studies, performed by Begemann and colleagues
[9], demonstrated the presence of a 124 kDa cell adhesion glycoprotein in chicken liver epithelial cells named L-CAM, which was converted into an 81 kDa protein by trypsinization in the presence of Ca
2+. Interestingly, these antibodies did not affect aggregation of retinal cells expressing R-cadherin instead of E-cadherin. Once all these pioneering studies were reconciled, in 1984, the name “cadherins” was introduced
[10] to identify this class of cell–cell adhesion molecule. The prefix “E” (for epithelial) was adopted for cadherin expressed by epithelial cells, and subsequent experiments performed by Takeichi’s group revealed the existence of other cadherins which have distinct cellular expression patterns, such as N- and P-cadherins
[11]. Once E-cadherin was definitely individuated as a cell–cell adhesion protein, the subsequent phases led to the cloning of the E-cadherin cDNA
[12], the individuation of the tertiary structure of E-cadherin extracellular domain
[13], the
res
earchtudy of the E-cadherin/catenin complexes
[14] and the demonstration of a key role of E-cadherin-mediated regulation of cellular replication
[15][16][15,16].
3. E-Cadherin Structure
E-cadherin is a single-span transmembrane protein. E-cadherin protein precursor is a polypeptide with a short signal sequence for import into the endoplasmic reticulum, a pro-peptide of about 130 amino acid residues (AA) and a mature polypeptide of about 728 AA (
Figure 1). The mature E-cadherin contains a transmembrane domain, a cytoplasmic domain of 150 AA and an ectodomain of 550 AA comprising five tandemly repeated domains. Four of these domains are known as cadherin repeats (EC1 to EC4), whereas EC5 is characterized by four conserved cysteines
[17]. E-cadherin forms calcium-dependent, homotypic cell–cell adhesion structures known as AJs that mediate intercellular adhesion
[18], cell polarity, cell–cell communication, cell survival, cell differentiation and tissue development
[19][20][21][22][19,20,21,22]. The extracellular domain is responsible for homophilic interactions between cadherin molecules expressed at the surface of neighboring cells
[17]. Cadherin cytoplasmic tails bind to proteins p120-catenin and β-catenin (alternatively, its homolog γ-catenin in some cell types), while p120-catenin regulates the stability of cadherin–catenin complexes at the plasma membrane
[23], and β-catenin interacts with the actin-binding protein α-catenin, which contains an actin-binding domain and physically links AJ complexes to the actin cytoskeleton
[23][24][23,24]. The integrity of the cadherin–catenin complex and the association with the cytoskeletal actin represent prerequisites for cell–cell adhesion
[23][24][23,24].
Figure 1. Schematic representation of E-cadherin protein. E-cadherin contains 5 extracellular cadherin (EC) repeats linked by Ca2+ binding sites, a transmembrane domain and an intracellular domain that binds p120-α-catenin and β-catenin.
4. The Pivotal Role for E-Cadherin in Salivary Gland Morphogenesis
Although the E-cadherin adhesion receptor mediates different, acknowledged functions during epithelial branching morphogenesis, relatively little is known of how E-cadherin, in addition to directly mediating intercellular adhesion, impacts the development of salivary acini and ducts. Recent studies showed that, during embryonic SMG morphogenesis, E-cadherin plays a decisive role in determining the differentiation of epithelial progenitor cells into acinar or ductal cells in a specific stage of embryonic development and in guiding the development of glandular structures until maturation
[25][36]. In vitro SMG organogenesis experiments from isolated SMG cells confirmed that E-cadherin is predominantly involved in the structuring of the branching morphogenesis of SGs
[26][37]. Clarifying the mechanism responsible for E-cadherin-mediated SMG development will have important implications for the general understanding of branching morphogenesis in the context of epithelial tissue development. It is now clear that the E12.5 SMG contains two distinct cellular populations that present a different E-cadherin junctional organization, which conditions the subsequent phases of cellular differentiation
[27][38]. The external cellular layer located in contact with the basement membrane consists of closely packed epithelial cells surrounding the polymorphic cells located in the region of the internal glandular bud. The role of E-cadherin in SMG development was investigated by inducing E-cadherin inhibition by the use of both specific antibodies against E-cadherin and siRNAs-mediated E-cadherin gene silencing. These interesting experiments revealed that the disorganized cells in the initial bud express E-cadherin and β-catenin uniformly and diffusely over their surface
[28][29][26,39]; in addition, another columnar cell population was recognized in the outer layer of the initial bud, in contact with the basement membrane, characterized by distinct E-cadherin junctions, likely to be linked to the columnar morphology. When the glandular bud grows and branches, these highly organized columnar cells remain in the outermost part. Strangely, during the E-cadherin inhibition experiments, the columnar organization of these outer cells was not lost. Probably, the lack of E-cadherin was compensated by N-cadherin, which is highly expressed in these cells. On the contrary, the cells of the inner region of the bud did not present well-structured E-cadherin junctions and also expressed markers typical of ductal cells, suggesting that they were probably destined to give rise to the ducts. The cells that gave rise to the ducts were identifiable as early as E13.5, arranged along the proximal–distal axis and characterized by a large number of F-actin filaments and by the expression of cytokeratin ductal marker K7
[25][36]. Only later, these ductal precursors acquired defined E-cadherin junctions, first detected at the apical–lateral borders of ductal cells, and appeared coincident with lumen formation
[25][36]. At this stage of glandular development, ZO-1 expression was also detected at sites apical to E-cadherin junctions, suggesting that ductal cells are linked through tight junctions
[25][29][36,39]. Therefore, a lower expression of E-cadherin in the interior layer of the glandular bud appears to be necessary to ensure cellular rearrangement; when ductal lumens are formed, the presence of E-cadherin appears to be necessary to ensure stabilization of the ducts in the developing gland (
Figure 2). Through inhibition studies performed by the use of siRNA and specific antibodies, the fundamental role of E-cadherin junctions in the ductal precursor fate was demonstrated during the lumenization process; they probably act by the modulation of apoptotic cascade
[30][31][32,40].
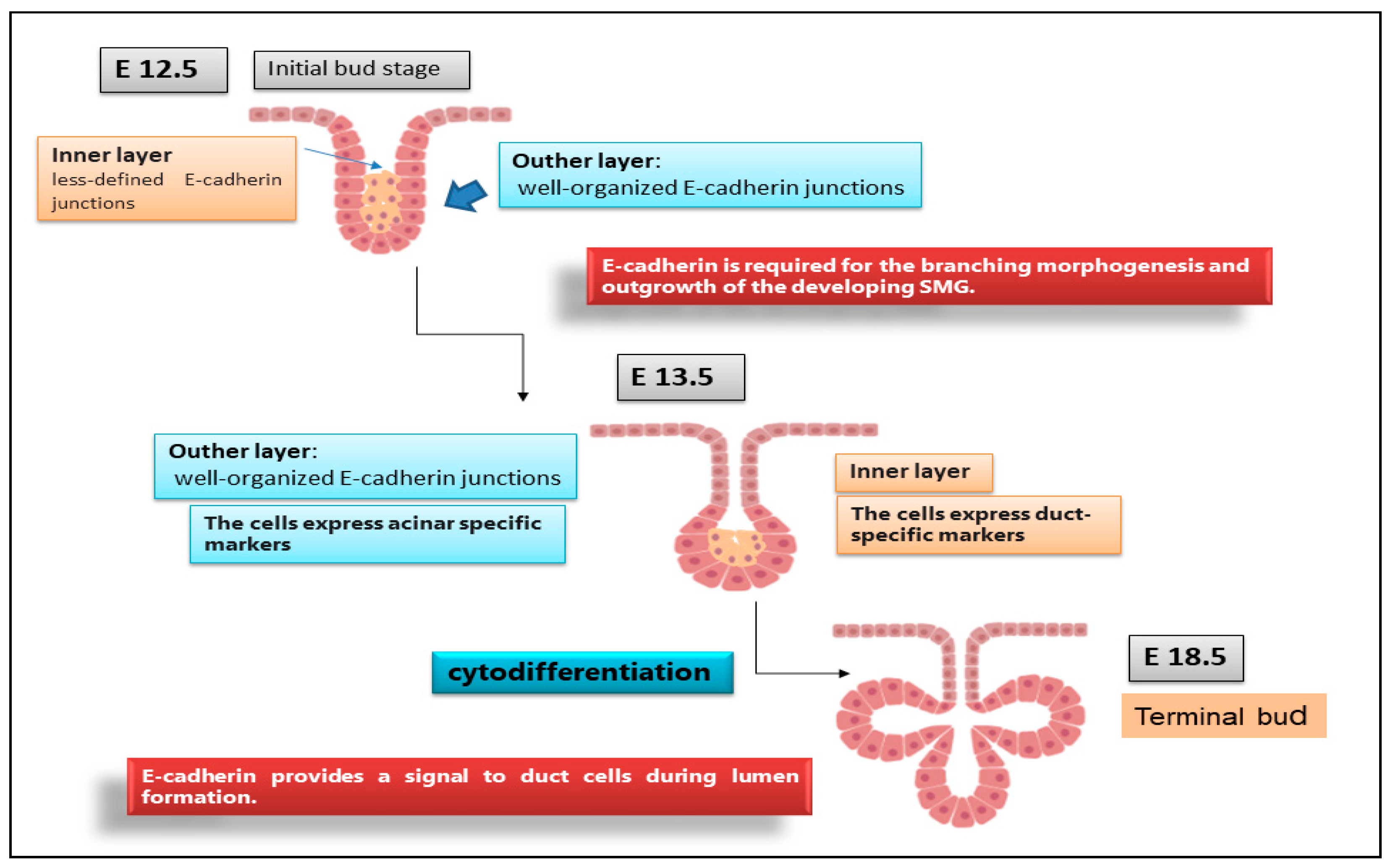
Figure 2. Organization of E-cadherin junctions during SGs morphogenesis. E12.5, E13.5, E18.5 represent stages of SGs embryonic development. At the initial E12.5, E-cadherin was localized to the lateral surfaces of the columnar cells that comprised the outer layer while, in the interior cells, was diffuse, indicating that these cells have less organized E-cadherin junctional structures. By E13.5, outer cell layer expressed a biochemical acinar marker demonstrating that acinar cells begin to differentiate very early in SGs development. The acinar progenitor layer completes the cytodifferentiation at E18.5, expressing E-cadherin in the peripheral cell layer.