Acute kidney injury (AKI) remains a complex challenge with diverse underlying pathological mechanisms and etiologies. The clinical applications of AKI biomarkers are becoming extensive and serving as relevant tools in distinguishing acute tubular necrosis from other acute renal conditions. Also, these biomarkers can offer significant insights into the risk of progression to chronic kidney disease (CKD) and in the context of kidney transplantation.
1. Introduction
Currently, international guidelines for the diagnosis and stratification of acute kidney injury (AKI) are based solely on the loss of glomerular function, assessed through serum creatinine (SCr) dosage and urinary output. This has been the norm for more than 100 years. Although these guidelines and classifications, such as RIFLE, AKIN, and KDIGO 2012, are essential in defining, stratifying, and treating patients, it has become evident that they primarily focus on renal function and excretion, lacking precision regarding the underlying injury mechanism. Indeed, it has become apparent that a phenomenon like acute kidney injury involving multiple aspects, such as etiology, pathophysiological mechanisms, prognosis, and treatment, should not be reduced into a single biochemical test. SCr might not be the ideal biomarker for acute kidney injury for multiple reasons. Firstly, creatinine production is not always the reflection of a stable state; it is the product of the metabolism of creatine and phosphocreatine in muscle cells. This production can vary significantly with factors such as age, gender, muscle mass, and protein intake. This variability also underscores that creatinine requires time to accumulate, rendering it a delayed marker. Furthermore, it is not specific to renal or tubular injury; elevated creatinine levels can be observed in all phenotypes ranging from prerenal azotemia to obstructive disease. Therefore, its elevation does not provide substantial evidence regarding the location, etiology, or treatment of the injury. Finding the ideal biomarker can be challenging, especially in the context of AKI. It should not only reflect the renal injury specificity and offer mechanistic insight but also be reproducible, hold a prognostic capacity, and be affordable and noninvasive.
Biomarkers are defined and categorized according to the BEST (Biomarkers, EndpointS, and other Tools) resource, a joint task force between the Food and Drug Administration (FDA) and National Institutes of Health (NIH), which divides biomarkers into different categories portraying their functions ranging from diagnosis and monitoring to prognosis and predictive value, leading to clinical and surrogate endpoints biomarker
[1]. Ideally, the biomarker should then undergo verification in multicenter cohorts, followed by prospective cohorts focusing on specific outcomes. After these validation steps, the biomarker should also be tested in specifically designed clinical trials to ensure its role in changing patient outcomes in clinical practice
[2]. Finally, in the case of AKI, an ideal biomarker may not replace SCr and urine output but should rather be considered as of additional value, as illustrated by an insightful model proposed by De Oliveira et al.
[3]. In this model, the three elements are combined in a dynamic visualization of the actual insult, combining injury with dysfunction. This concept brings us closer to the truth, where etiologies may range from initial phases of damage, where the injury is represented by an increase in biomarkers while function is still preserved, indicated by normal serum creatinine and urine output. On the other end of the spectrum, scholars find etiologies with dysfunction, leading to a rise in creatinine and a decrease in urine output, but without injury or an increase in biomarkers.
2. Secretion to Significance of Biomarkers
In approaching AKI biomarkers, it is essential to recognize that various categorization methods exist, including differentiation by site of secretion or whether these are assessed in blood or urine (Figure 1). However, it seems more relevant to categorize biomarkers according to their alignment with underlying pathological processes.
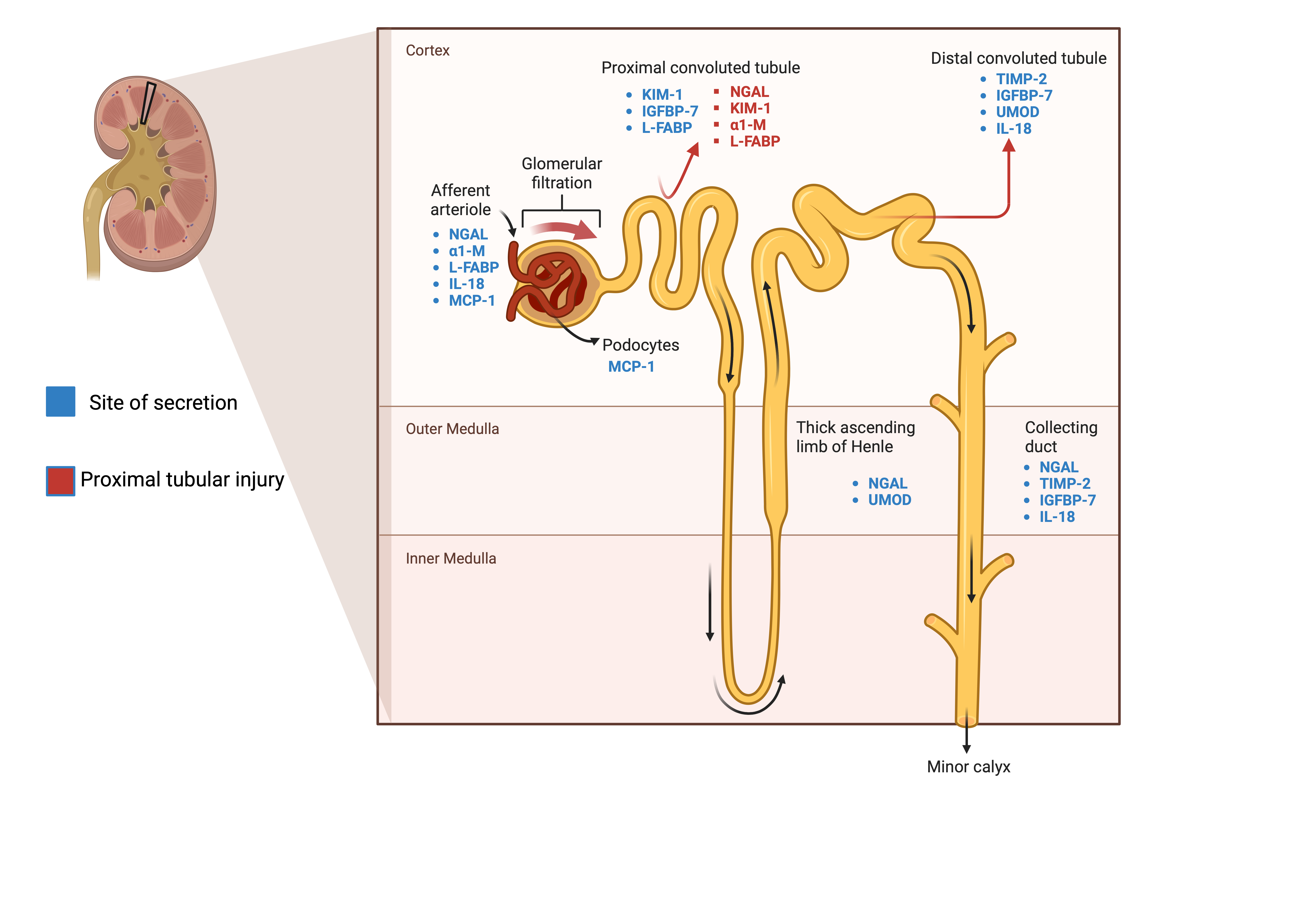
2.1. Tubular Injury
2.1.1. NGAL, Neutrophil Gelatinase-Associated Lipocalin
NGAL, also known as lipocalin 2 (LCN2), plays a role in facilitating the transport of hydrophilic substances across cell membranes
[4], participates in cellular defense mechanisms, and influences iron metabolism
[5]. NGAL is not exclusively secreted by the kidney but is found in epithelial cells throughout various organs, such as the lungs and the digestive system, in response to various inflammatory signals
[6]. The renal secretion of NGAL, particularly in the ascending limb of the loop of Henle and within the collecting ducts, accounts for a negligible fraction of the overall plasma NGAL concentration, as confirmed by renal vein sampling
[7]. Plasma NGAL is completely reabsorbed by the proximal tubule after it is freely filtered. Therefore, the detection of urinary NGAL of plasma origin is only possible in the case of proximal tubular injury or when the synthesis exceeds the tubular reabsorption capacity. On the other hand, a decrease in GFR leads to a decrease in NGAL excretion and, therefore, increases its plasma accumulation.
2.1.2. KIM-1, Kidney Injury Molecule 1
KIM-1, a transmembrane glycoprotein serving as an adhesion molecule, was originally identified in the S3 segment of the proximal tubule. While its expression remains minimal in normal kidneys, it experiences significant upregulation in response to ischemic-reperfusion injury in rat models
[8]. This observation was extended to human subjects, where the presence of the soluble released form of urinary KIM-1 was confirmed to be associated with acute tubular necrosis, thus establishing it as a marker of proximal tubular injury
[9]. It is worth noting that the genes that code for KIM-1, as well as NGAL, were found to be significantly upregulated late after the injury. This could potentially function as an indicator of persistent renal damage following the initial acute insult and may, therefore, serve as a prognostic factor for the development of subsequent chronic kidney disease (CKD)
[10].
2.1.3. TIMP-2 Tissue Inhibitor of Metalloproteinases-2 and IGFBP-7 Insulin-Like Growth Factor-Binding Protein 7
G1 cell cycle arrest is a recognized response mechanism for kidney injury
[11]. TIMP-2 and IGFBP-7 play important roles by upregulating the expression of p27 and p53/21, respectively, and consequently inhibiting the progression of the cell cycle through the inhibition of cyclin-dependent protein kinase complexes. Notably, a predominant localization of expression is observed in the area of the collecting duct/distal tubule for TIMP-2 and in the proximal tubules, the collecting duct/distal tubules, and the podocytes for IGFBP7
[12]. The products of urinary TIMP-2 and IGFBP-7 have been established as early biomarkers for acute kidney injury and validated in independent multicenter cohorts
[13]. They are currently available commercially under the name NEPHROCHECK. Both biomarkers are continuously expressed in tubular cells, and they are subsequently released and detectable in urine as early as 4 h following kidney injury. This release is more pronounced due to the reduced endocytic uptake by damaged proximal tubules
[14].
2.2. Tubular Function
2.2.1. Cystatin C
Plasma cystatin C is a well-established biomarker for evaluating glomerular function and filtration, offering an advantage over creatinine because it remains unaffected by variables such as age, gender, muscle mass, and dietary choices. Its production is constant through all nucleated cells, and it is freely filtered in the kidneys without it being secreted or absorbed. Longtime used in clinical practice, leading recently to the development of a cystatin C-based equation, where sex and race variables are not required for the estimation of GFR
[15].
2.2.2. α1-M, α1-Microglobuline
In the assessment of tubular function, we also have α1-M, also called protein HC and α
1-microglycoprotein. Like NGAL, it belongs to the lipocalin family. This glycoprotein, with immunosuppressive proprieties, is synthesized by the liver and released into the bloodstream, where it exists either in a free form or associated with IgA immunoglobulin. The former is freely filtered by the glomerulus and is then mostly reabsorbed by the proximal tubule, making it a marker of proximal tubule dysfunction
[16][17].
2.2.3. L-FABP, Liver Type Fatty Acid-Binding Protein
FABP, a member of the superfamily of lipid-binding protein LBP, includes L-FABP, which is secreted by the liver. Their primary role is to bind to fatty acids, particularly in the context of hypoxic injury, facilitating their transport and, therefore, serving as ischemic markers. This function also contributes to the reduction of oxidative stress
[18]. Furthermore, L-FABP, secreted by the liver, is also expressed in the kidney, predominantly among the proximal tubular cells. This expression increases during acute proximal ischemic injury, making urinary L-FABP an early marker of tubular function.
2.2.4. UMOD, Uromodulin
Also known as Tamm–Horsfall protein, UMOD is a glycoprotein exclusively secreted in the kidney and specifically by the thick ascending limb of the loop of Henle and the early distal convoluted tubule. It plays a role in salt and blood pressure homeostasis as well as immunomodulatory and anti-inflammatory effects
[19]. Diminished urine uromodulin levels have been linked to more pronounced histological lesions, including interstitial fibrosis, tubular atrophy IFTA, and glomerulosclerosis, whereas it was negatively correlated with inflammatory biomarkers such as IL-6, TNFα, and NGAL
[20].
2.3. Kidney Inflammation
2.3.1. IL-18
IL-18 is a pro-inflammatory cytokine, a member of the IL-1 family produced by kidney macrophages
[21][22]. It is found in renal tubular epithelial cells and particularly in the intercalated cells of the distal tubule and the collecting duct
[23], but also the proximal tubule
[3], possibly reflecting the diverse types and locations of injury and, therefore, the associated inflammatory responses. The levels of IL-18 doubled in animal models of ischemic acute renal failure, while the neutralization of IL-18 before the insult resulted in reduced creatinine level, tubular damage on histology and neutrophile
[24], and macrophage infiltration
[25], in particular M2 profibrotic macrophages highlighting its role in the progression of AKI to fibrosis and CKD
[26].
2.3.2. MCP-1, Monocyte Chemoattractant Protein-1
Serum MCP-1 or Chemokine (CC-motif) ligand 2 (CCL2) is secreted by epithelial, endothelial, and immune cells. Its primary function is to recruit monocytes and macrophages to the site of injury through interaction with the cell surface receptor CCR2
[27]. In the kidney, its expression is triggered by pro-inflammatory cytokines, such as IL-18. When MCP-1 is detected in urine, it stems from local production within the kidney rather than being a product of serum filtration in the glomerulus
[28]. MCP-1 is notably recognized for its association with endothelial dysfunction and its role in predicting the activity of lupus nephritis
[29].
2.4. Adaptive Repair and Fibrosis
2.4.1. EGF, Epidermal Growth Factor
EGF, through its receptor EGFR, plays a role in adaptive repair and fibrosis in acute kidney injury. In a physiological state, away from injury, reduced EGFR activation impairs renal epithelial development. However, when subjected to ischemic or toxic injury in the proximal tubule, EGFR phosphorylation increases.
2.4.2. Pro and Anti-Angiogenic Markers
VEGF, vascular endothelial growth factor, is essential in angiogenesis and endothelial cell differentiation; its mRNA was mainly detected in podocytes, distal tubules, and collecting ducts
[30]. It plays a role in renal repair responses to ischemic injury, where its expression is upregulated in tubular cells. This is followed by the secretion of a free form and mainly extravesicles, promoting endothelial proliferation.
3. Clinical Applications of Biomarkers
3.1. Acute kidney Injury Post-Cardiac Surgery
Acute kidney injury occurs in 14% to 30% of hospitalizations for cardiac surgery, worsening the overall prognosis and increasing the risk of mortality, prolonged hospitalization, and healthcare burden. Therefore, the need for early injury detection is crucial. The elevation in SCr or the reduction in urinary output indicates already advanced injury. Translational Research in Biomarker Endpoints Consortium (TRIBE-AKI) was designed to explore the role of postoperative biomarkers in predicting short-term outcomes in the context of cardiac surgery, such as acute kidney injury, the need for dialysis and increased hospital stay
[31][32], but also the long-term prognosis, such as overall mortality and progression to CKD.
In 2013, five urinary biomarkers (NGAL, IL-18, KIM-1, L-FABP, and albumin) were measured over the three days following cardiac surgery in 1199 patients from six different centers, with a median follow-up of three years. These biomarkers were found to be independently associated with a 2 to 3.2-fold increase in mortality risk in patients who had clinical AKI and measured for the highest percentile of this panel. In patients without clinical AKI, IL-18 and KIM-1 were also independently correlated with mortality
[33]. The concentrations of the five urinary biomarkers were also associated with a longer duration of AKI, and a duration longer than seven days was correlated with a 5-fold increase in three-year mortality
[34]. It is important to highlight that hematuria, proteinuria, leucocyte esterase, and nitrite, detected in dipstick tests, can influence the concentration of urinary biomarkers. This impact was evident in a post hoc analysis involving four specific biomarkers (NGAL, IL-8, KIM-1, and L-FABP), where the mentioned factors resulted in false-negative outcomes. In such cases, these biomarkers do not precisely mirror tubular injury
[35].
In the same cohort, pre-operative and 3-day post-operative plasma NGAL was measured in 1191 patients undergoing cardiac surgery, with a median follow-up of 3 years. Pre-operative levels of plasma NGAL were also found to be predictive of 3-year mortality after cardiac surgery, indicating prognostic value. Meanwhile, post-operative NGAL lost this association when correlated with SCr, highlighting the different physiopathologies of urinary and plasma NGAL as previously discussed
[36].
3.2. Cardio-Renal and Hepatorenal Syndromes during Acute Hospital Illness
Identifying the etiology of AKI presents a significant challenge in cirrhotic patients who often present with multiple comorbidities and are susceptible to infectious complications and potential adverse effects from treatments such as diuretics. The etiology of AKI in these cases may range from prerenal azotemia to acute tubular injury (ATI) and hepatorenal syndrome (HRS). Timely and accurate diagnosis is crucial, as AKI significantly increases the risk of mortality in these patients, and management approaches differ significantly.
For all these reasons, there is a pressing need to identify valid biomarkers that can aid in distinguishing between the diagnoses of hepatorenal syndrome, tubular injury, or prerenal azotemia. Early on, studies of biomarkers concentrated on using them as a tool to identify patients who had tubular injury and, thus, should be excluded from vasopressive therapies. By incorporating biomarkers along with clinical judgment and other arguments, clinicians can refine their diagnosis. One study combined uNGAL, IL-18, L-FABP, and albumin, defined specific cut-offs for each biomarker, and showed that patients with all biomarkers above the cut-off had a 91% of having ATN vs. 7% for those without any positive marker
[37]. Later, the focus shifted more towards the ability to identify patients who had HRS and should benefit from specific therapies, even predicting which patients could benefit further from such therapy. In this context, a study included one hundred sixty-two patients with cirrhosis and acute kidney injury, followed until death, liver transplant, or 90 days after inclusion; 39.5% of these patients had hepatorenal syndrome.
IL-18 was also studied in this context but was found to have lower accuracy in predicting ATN vs. HRS but was associated with higher hospital mortality
[38][38][39]. Using these biomarkers can also provide an additional argument in the decision between combined kidney–liver or liver transplantation alone
[40].
Cardiorenal syndrome is another clinical scenario where decisions are not as straightforward, and both cardiologists and nephrologists often lack specific arguments to balance the need for diuretics and the rise in creatinine. Current therapeutic approaches involve diuretics to restore effective renal perfusion pressure and increase hemoconcentration, which are associated with decreased mortality and heart failure rehospitalization, even in the context of in-hospital worsening renal function
[41]. As relying solely on clinical parameters may be insufficient to identify response to therapy, biomarkers can serve as an additional factor in this situation, as well as in resolving heterogeneity in pathophysiological mechanisms of cardiorenal syndromes. Urinary NGAL and IL-18 were found to be independently associated with AKI progression in patients with acute decompensated heart failure, and the addition of these biomarkers along with urinary angiotensinogen to a clinical model improved the risk stratification and identified the population with the highest risk of adverse kidney outcomes
[42]. Baseline urinary L-FABP levels in patients hospitalized with acute decompensated heart failure were also found to be an independent predictor of AKI in these patients
[43].
3.3. Diagnosing Acute Interstitial Nephritis
Diagnosis of acute interstitial nephritis (AIN) and distinction with acute tubular necrosis and other acute renal conditions can be challenging. It is crucial to establish the diagnosis of AIN in a timely manner since efficient therapeutics exist, entailing discontinuation of the causative agent or treating the underlying disease. The use of biomarkers in this context is also beneficial, providing an alternative to kidney biopsy in cases when diagnosis is unclear. To identify potential biomarkers, a study included 218 patients who underwent kidney biopsy for acute kidney injury from two different centers. The histological diagnosis of AIN was found in 15% of the biopsies, reflecting its actual proportion among the causes of acute kidney injury. Among twelve urine and ten plasma biomarkers measured, urinary TNF-α and IL-9 were independently associated with AIN, and their levels were higher in patients whose biopsies showed more severe histological lesions of AIN, such as tubulitis or lymphocytic and eosinophil infiltrates. What is noteworthy is the creation of two models: one comparing these biomarkers to a clinician’s judgment and the other comparing them to a model consisting of clinical variables typically associated with AIN. The addition of these biomarkers to both models significantly improved their ability to predict the diagnosis, raising the AUC of the clinicians from 0.62 to 0.84 and the clinical model from 0.69 to 0.84. This serves as an example of how biomarkers can be incorporated into clinical or other biological models, thereby enhancing their utility
[44]. In addition, CXCL9 has recently been identified as a biomarker for AIN. It emerged as the top protein biomarker among 180 urine proteins associated with AIN in urine proteomics analysis of 88 patients with AKI, of which 35% had biopsy-proven AIN. Urinary CXCL9 also exhibited a correlation with the severity of histological lesions. This aligns with the fact that CXCL9 is a chemokine, by binding to CXCR-3, whose expression is induced by IFN-γ, guides activated T lymphocytes to the site of inflammation, primarily in tubular regions. The inclusion of CXCL9 in the previously mentioned models further enhanced their performance. Finally, the combination of CXCL9 with TNF-α and IL-9 was found to have the most diagnostic accuracy
[45].
3.4. Prediction of Contrast-Associated AKI
Many studies have suggested the potential use of biomarkers in predicting contrast-associated AKI, such as NGAL and IL-18
[46][47]. However, most were single-center studies with low event rates. Identifying high-risk patients can guide the nephrologist in approving the use of a contrast test, especially in non-urgent situations. It can also assist in determining which patients require hospitalization and close follow-up. In this regard, a larger study based on the PRESERVE trial cohort was conducted, measuring plasma biomarkers in 916 patients and urine biomarkers in 797 patients with chronic kidney disease: MCP-1, KIM-1, NGAL, IL-18, UMOD, and YKL-40 in 19 different centers. These markers were measured one to two hours pre-angiography. Patients with pre-angioplasty higher plasma KIM-1, NGAL, and YKL-40 were found to have a higher risk for major adverse kidney events such as the need for dialysis or persistent decrease in kidney function (defined as an increase in serum creatinine by >50%) as well as and death within 90 days (MAKE-D). Urine creatinine-corrected IL-18, MCP-1, and YKL-40 were also correlated with higher MAKE-D.
3.5. AKI Biomarkers in Kidney Transplantation
Biomarkers also play a role in the field of kidney transplantation, where they could assist in decisions regarding donor selection and kidney graft monitoring. They can also have the potential to improve the prediction of graft survival risk, as demonstrated recently in a study involving 709 stable kidney transplant recipients. Urinary and plasma levels of NGAL and calprotectin were measured at least two months post-transplant, with a follow-up period extending to 58 months. The study revealed that plasma NGAL independently predicted renal allograft loss
[48].
In the post-transplant context, another valuable tool for assessing kidney allografts is donor-derived cell-free DNA (dd-cfDNA), as it is released into the bloodstream with a half-life ranging from 30 to 120 min in the context of graft injury
[49]. What appears clinically relevant is its high negative predictive value, helping clinicians avoid invasive biopsies in high-risk patients. In a retrospective observational study of 317 kidney transplant recipients with preserved kidney graft function, rejection episodes were more commonly found in patients with high dd-cfDNA (≥1%) compared to those with low dd-cfDNA (<0.5%)
[50]. Furthermore, dd-cfDNA elevation precedes other clinical manifestations of rejection and detection of de novo donor-specific antibodies by several weeks. dd-cfDNA correlates strongly with antibody- and T-cell-mediated rejection, but it is not entirely specific to graft rejection as elevated levels are also observed in conditions such as acute tubular necrosis, pyelonephritis, and BK virus nephropathy
[51].
Ultimately, AKI biomarker assessment in combination and integrated with relevant clinical information should enable tailoring of AKI management and treatment of individual patients at early stages, with therefore the potential to improve clinical practice, save costs, and reduce risk of progression to CKD.