The concept of sustainability, with a focus on energy, has emerged as a central tenet in addressing the mounting global challenges of environmental degradation and resource depletion. Indicators of sustainability focusing on energy are crucial tools used to assess and monitor progress toward achieving a more sustainable energy system. These indicators provide valuable insights into the environmental, social, and economic dimensions of energy practices and their long-term impacts. By analyzing and understanding these indicators, policymakers, businesses, and communities can make informed decisions, formulate effective policies, and steer their efforts toward a more sustainable energy future. These indicators serve as navigational guides, steering the world toward energy practices that support both present needs and the well-being of future generations.
1. Introduction
It is increasingly important to include environmental, social, and governance considerations when evaluating results, especially in an industry as impacting as energy. Incorporating sustainability indicators assessments provides a more holistic evaluation of the long-term feasibility and harmony of deals with broader sustainable development objectives
[1]. The sustainable principles serve as a unifying framework, promoting the responsible stewardship of resources, inclusivity, and intergenerational well-being. It further underscores how these principles provide a shared foundation for individuals, organizations, and societies to collaboratively contribute to a sustainable future
[2].
A sustainability index implies providing information on the mechanisms and logic that operate in the area under analysis and quantifying the most important phenomena that occur in the system under study
[3]. With this index, it is possible to understand how human activity affects the environment, alert people on the risks of survival, predict future situations, and offer sustainable alternatives in making better policy decisions
[4].
Indicators make it possible to select the most relevant information, simplify complex phenomena, quantify qualitative information, and communicate information between collectors and users. They have applications for evaluating conditions and trends about objectives and goals, comparing results considering similar methodologies, and providing warning information to anticipate future conditions, among several other uses
[5].
Sustainability is a balancing act between social, environmental, and economic dimensions
[6]. The three dimensions of sustainability may imply conflicts due to the very concept of each part: environmental sustainability means the temporal maintenance of the fundamental characteristics of ecosystems under the use of their components and interactions; economic sustainability results in stable profitability over time; social sustainability associates the idea of systemic organization that must have cultural and ethical values of involved groups and society in a way that is acceptable to organizations over time
[7].
These sustainability dimensions represent different aspects of human well-being and development that need to be balanced to ensure a sustainable and equitable future for both current and future generations
[8]. These dimensions are interconnected and interdependent
[9]. Achieving sustainability requires finding synergies and trade-offs among them, as actions in one dimension can have implications for the others
[10].
The idea of the interconnections between sustainability dimensions is presented in Figure 1, especially focusing on energy; there are topics that are in more than one dimension, such as electricity access, which has social and economic importance.
Figure 1. Interconnection of sustainability dimensions.
Environmentally, indicators may include the share of renewable energy in the total energy mix, the reduction in greenhouse gas emissions, and improvements in energy efficiency. Social indicators might encompass access to affordable and reliable energy services for all, equitable distribution of energy resources, and the creation of green jobs in the renewable energy sector. On the economic front, indicators may assess the cost-effectiveness of sustainable energy solutions, investments in renewable energy infrastructure, and the overall resilience of energy systems to market fluctuations.
The social dimension focuses on the well-being and quality of life of people
[11]. It includes aspects such as social equity, justice, human rights, health, education, cultural preservation, and community engagement
[12]. In a sustainable society, social systems should ensure that all individuals have access to basic needs, opportunities, and a high quality of life without compromising the needs of future generations.
The environmental dimension relates to the health and resilience of ecosystems and the natural environment. It involves maintaining biodiversity, conserving resources, reducing pollution, addressing climate change, and promoting sustainable land use and resource management
[13]. A sustainable approach considers the Earth’s finite resources and aims to minimize negative impacts on the environment.
The economic dimension concerns the long-term viability of economic systems. It involves promoting economic growth and development while ensuring that it does not come at the expense of social well-being or environmental health
[14]. Sustainable economies strive to balance prosperity with the responsible use of resources, ethical practices, and consideration of the impacts of economic activities on society and the environment
[15].
The energy supply system consists of the physical infrastructure that defines the system configuration for resource input
[16] and its expected response
[17]. Resource inputs include primary energy inputs, such as crude oil, coal, natural gas, hydropower hydroelectric
[18], nuclear
[19], or renewable energy. The combination of resources and the configuration of infrastructure defines the production capacity of the energy system and, therefore, its ability to meet society’s needs at any given time
[20].
2. Technology and Efficiency Improvement
Hydrogen fuel cell buses represent a promising avenue for sustainable urban transportation, but their energy efficiency is contingent on the effective management of power distribution and consumption. The study presented by Shen et al.
[21] delves into the utilization of deep reinforcement learning algorithms to enhance the energy efficiency of hydrogen fuel cell buses by dynamically adjusting their velocity profiles. As well evaluated nowadays, the energy supply has become more efficient with the use of time series forecasting
[22] using hybrid models
[23], classification (computer vision
[24], convolutional neural networks
[25], deep neural networks
[26], multilayer perceptron, and k-nearest neighbors
[27]), and Internet of Things (IoT) using embedded systems
[28].
Measuring energy sustainability using fuzzy logic can be performed with biological inputs, which can have a highly polluting character when released inappropriately into the environment. Parameters are first defined in governance in order to reduce this impact as quickly as possible so that these two processes, being carried out by specialized teams, are later assigned importance in each social, economic, technical and environmental indicator in the form of numerical scores according to the scenario and location analyzed formulating technological priorities seeking planning and decisions that are more appropriate
[29].
These strategies can be carried out from a small site to reach entire countries, and they can become more complex and assist energy policies by categorizing the selection of energy plant sites, structural decisions assessing global costs, and productivity gains due to increased exports by raising the renewable matrix with trading partners and the risks associated with this energy stance with existing and expected marketing possibilities
[30][31][32].
Although artificial intelligence (AI) algorithms are complex and their combination with other statistical tools is required by technical criteria
[33], at the end of the operation, the results must be easy to understand for legislators and decision-makers in the area of energy policy, clearly dealing with the recognition of qualitative and quantitative indicators and having robustness, that is, guaranteeing the conclusions obtained, and there is a need to minimize the consumption of time and financial resources compared to human choices experts presenting strategies that improve the investment environment and reduce risks in operational energy planning both micro and macro population environments
[34].
Within the electricity sector, generation, transmission, and distribution systems are encompassed by specific protocols. It can be evaluated from the literature that these established standards facilitate the adoption of a certain method that will have greater adaptability in the iteration of the data that will be inserted in the proposed model and obtain an energy sustainability index
[35], determining the degree of influence for each related indicator
[36]. Focused on improving energy access, researchers have evaluated advanced methods to analyze the transmission lines’ performance, using models to determine how the leakage current, electric field, and partial discharges are affecting the power system supply.
Among the methodologies for creating and developing these indices in line with the objectives of sustainable development within the electricity sector, three methods stand out that are related to AI algorithms: multicriteria decision analysis, aggregate normalization in minimum and maximum discrepancies, and hierarchical analytical process. These are related in the three dimensions most evaluated in the literature: environmental, economic and social. In addition, the cultural, institutional, and technical aspects can also be added, depending on the complexity and need foreseen, as well as the size of each project
[37][38][39].
To classify technologies that are similar in technical characteristics, fuzzy logic can be an excellent alternative for reducing the costs and time required for investor decisions, by establishing criteria and weights for the needs envisaged by this group using the technique of the order of preference using similarity in an ideal system solution (TOPSIS)
[40], improving the consistency of comparisons by matrices and sub-criteria, and it is used more significantly and with less sensitivity in the classification of nuclear plants; however, it is not limited to this type of plant and can be applied to other energy transformation plants.
Through the vision of a hierarchical analytical process (AHP), weight criteria are determined, and the relative importance values of different parameters are calculated; when embedded in logic such as fuzzy logic, the consistency of data from human uncertainty can be improved
[41]. This allows decisions to be made that are more sensitive, realistic, and concrete among the particularities from the perspectives of energy policy alternatives with conflicting criteria, both in the AHP tool and in the analytical network process (ANP), which is the most widely used technique in the approximate comparison of pairs within the context of multi-criteria decision methods; both complement each other, since the AHP methodology makes it more consistent and reduces the redundancy of the ANP, which has the disadvantage of disregarding mutual relationships between criteria, which the ANP tool adjusts for.
Formulating the sustainability index prior to and before applying the AHP and ANP tools, the authors in the literature carry out a SWOT analysis, which consists of assessing the strengths, weaknesses, opportunities, and threats in a matrix to which a project may be subjected at all stages from conception to operation
[42].
Smart electric railway networks play a pivotal role in modernizing and optimizing public transportation systems while minimizing their environmental footprint. The research presented by
[43] examines the methodologies utilized to design and implement energy-management systems in these networks to ensure efficient energy usage and reduced operational costs.
Microgrids, which are localized energy systems that incorporate distributed energy resources, have gained prominence as a means to enhance energy resilience and reduce environmental impact. However, the dynamic and decentralized nature of microgrids poses complexities in managing energy supply and demand.
3. Sustainable Development Index and Indicators
The objectives of sustainable development are interconnected and have as one of their fundamental requirements the elaboration of energy policies that enable the generation of sustainable electricity and that can be ranked from the formulation of national and/or international indexes generated from a series of data and indicators
[44]. This can be carried out through operations with the statistical analysis and aggregation of an index composed of different indicators previously chosen
[45].
There is a relationship between the dimensions of sustainability and their resources. In
Figure 2, the environmental (ENV), economic (ECO), social (SOC), technical (TEC), and institutional (INS) dimensions are placed with their source, and the interconnection between the dimensions is presented in gray.
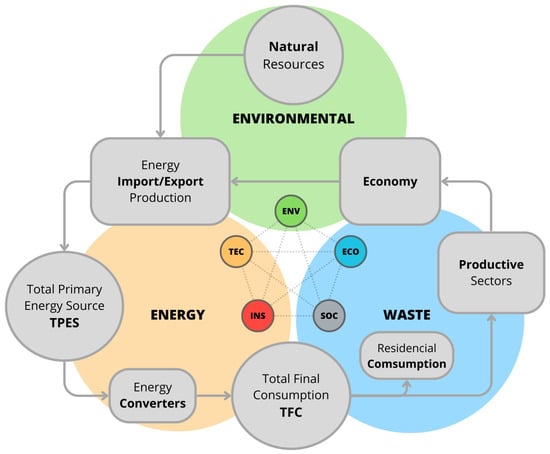
Figure 2. Dimensions and indicators of sustainability.
Sustainability Indicators
The indicators have scores characterized by relevance, contextual sensitivity, and robustness, where they can combine and produce distinct indices at the municipal, state, or country scale concerning the quality of electricity, low carbon financing, and social effects such as job creation and acceptability of technology by society
[46].
Regarding the relationship between consumption and quality of energy produced, the factor of the human development index (HDI) is considered an indispensable precedent and significantly related to the volume of energy consumption per person and is a determinant in the strategies that can be adopted according to socioeconomic development associated with government regulation; traditionally, in higher income countries, these strategies are the established investments and promotion of a safer and environmentally friendly energy market
[47].
When the HDI is incorporated into the joint assessment of the management of the electricity matrix, equity in energy access, environmental sustainability, and energy security, the formation of the scope contained in the energy sustainability index provided by the World Energy Council of the analyzed region is obtained
[47].
Considering the environmental, economic, social, technical, and institutional dimensions and giving the description of indicators to measure sustainability, the significance of each indicator is presented in Table 1.
Table 1. Indicators purposes and your references.
These indicators are used to evaluate the level of sustainability for the considered dimensions. These indicators are calculated as follows:
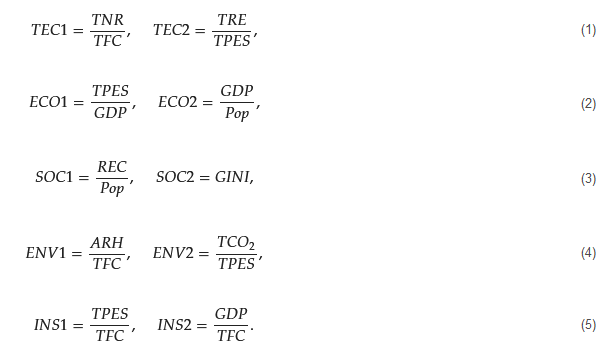
To apply the indicators, it is necessary to normalize them to have a standard parameter for comparisons. There are some indicators that have used by the same authors, which is because there is a relationship between indicators. In Table 2, a matrix of these relationships is presented.
Table 2. Relationship matrix between indicators.
Any index of a regional, national, or international nature requires interpretation prior to its application in order to establish a reliable characterization, verifying that additional indicators can be included and that it meets the prerequisites designated by the targets previously established in governance plans. Subsidies and guarantees are critical factors within developing countries and can be considered as additional indicators to measure their importance quantitatively and qualitatively [57].
Besides all indicators having a relation to the indicators of the same dimension, some of them have an additional relationship with other dimensions, which highlights the statement previously discussed in the introduction section regarding the interconnection between the sustainability dimensions.
The determination of the weights of each indicator, especially when there is a large number of them, becomes one of the biggest problems in the aggregation of multiple-criteria decision analysis, so it is recommended to use the criteria of the local energy policy within computational resources in the literature, such as statistical tools, data envelopment analysis, the method of organizing, and the ranking of preferences in order to carry out the enrichment of evaluations, which can collaborate in reducing the difficulty or subjectivity proposed in the creation and methodological development of an environmental performance index of the energy generated in each project. In addition, this computational and human analysis should be established in different scenarios proposed preliminarily, and sensitivity analyses will be designed after obtaining data
[58].
When structuring different variables in the analysis of indices, one can seek to evaluate the correlation and dependence of two series via a cross-validation model that collaborates in the understanding of the established conditionals and in the analysis of the generated curves determining what the dependent and independent variables are
[59]. By verifying different biomass electricity-generation technologies using this tool, aided by green total productivity factors through a hierarchical analytical process, it is possible to verify which of the alternatives are the most and least cost-effective in the economy, as well as which have the greatest and lowest emission of greenhouse gases or pollution at the local level and whether the technological innovation that has less polluting character is justified and what maximum investment limit must be met
[60].
4. Conclusions
The sustainability concept focusing on energy is a critical and multifaceted approach that recognizes the importance of preserving and efficiently utilizing our planet’s finite resources.
By shifting toward renewable and clean energy sources, such as solar, wind, hydro, geothermal, and bioenergy, we can significantly reduce greenhouse gas emissions and curb the detrimental impact of fossil fuels on our environment. Embracing sustainable energy solutions not only mitigates the effects of climate change but also fosters energy independence and resilience, as these sources are naturally replenished and less susceptible to geopolitical tensions.
Integrating sustainable energy practices into our daily lives and industries promotes economic growth and job creation. The transition to clean energy technologies and infrastructures opens up new avenues for innovation and investment, stimulating green industries and green-collar employment opportunities. Energy efficiency used as a daily practice, for instance, reducing the consumption of air conditioning, has an impact on the preservation of natural resources and a reduction in the emissions of pollutants.
The concept of sustainability in energy also entails energy efficiency, which involves optimizing our energy consumption across various sectors. Implementing energy-efficient technologies and practices, such as smart grids, energy-efficient buildings, and industrial processes, can significantly reduce energy wastage and enhance overall system performance. However, achieving a sustainable energy future requires collaborative efforts on a global scale. Governments, businesses, communities, and individuals must work together to prioritize and implement sustainable energy policies, promote research and development about innovative technologies, and engage in responsible energy consumption habits.
Embracing the sustainability concept focusing on energy is not merely an option but an imperative for the well-being of current and future generations. By committing to sustainable energy practices, we can create a cleaner, healthier, and more prosperous world while safeguarding the planet’s natural resources for the benefit of all life forms. It is a collective responsibility and a beacon of hope for a brighter and more sustainable future.