Legionella pneumophila is the causative agent of Legionnaires’ disease, causing fever and lung infection, with a death rate up to 15% in severe cases. In the process of infection, Legionella pneumophila secretes over 330 effectors into host cell via the Dot/Icm type IV secretion system to modulate multiple host cellular physiological processes, thereby changing the environment of the host cell and promoting the growth and propagation of the bacterium. Among these effector proteins, SidE family proteins from Legionella pneumophila catalyze a non-canonical ubiquitination reaction, which combines mono-ADP-ribosylation and phosphodiesterase activities together to attach ubiquitin onto substrates. Meanwhile, the activity of SidE family proteins is also under multiple modulations by other effectors.
1. Introduction
Gram-negative bacterium
L. pneumophila was identified in 1976 at the annual convention of American legion, which caused a serious pneumophila, resulting in a lethality rate of 15.9%
[1]. It has been reported that the pathogenic bacteria
L. pneumophila has a versatile arsenal of effectors, keeping its virulence by expressing over 330 individual effectors through the Dot/Icm secretion system
[2][3]. Moreover, further studies of pathogenic strategies revealed that after entering the cytoplasm of the host cell, the bacterium avoids its lysosomal-mediated degradation by escaping the endosomal-trafficking pathway and establishes
Legionella-containing vacuoles (LCV)
[4]. These specialized membrane-bound organelles are rich in nutrients and without lysosome hydrolases, providing
Legionella with an ideal environment for its intracellular replication
[5]. During the formation of LCVs, many post-translational modifications (PTMs) are involved, removing chemical moieties from protein residues, or attaching modifying groups to target protein, which mediates numerous physiological processes by their unique biochemical activities. Up to now, over 400 different types of PTMs have been identified in eukaryotic cells such as phosphorylation, glycosylation, acetylation, ADP-ribosylation and ubiquitination
[6][7][8]. Among these, ubiquitination, a ubiquitous post-translational modification, which regulates a variety of physiological processes in eukaryotic cells, such as protein homeostasis, cell cycle, immune response, DNA repair and vesicle transport, has been studied for several decades
[9].
The function and mechanism of canonical ubiquitination has been already well established. It occurs through a series of enzymatic reactions. First, the ubiquitin activating E1 consumes ATP and activates the C-terminal carboxyl group of ubiquitin and forms a thioester bond with cysteine at the active site of ubiquitin conjugating enzyme E2. Then, ubiquitin ligase E3 transfers ubiquitin from E2-Ub to a specific substrate. Finally, an isopeptide bond is formed between the carboxyl group of glycine at position 76 of ubiquitin and the ε-amino group of Lys or the α-amino group of Met1 of a substrate protein
[10][11]. Intriguingly, owing to the key role of ubiquitination in the life of eukaryotic cells, many pathogens have derived a series of effector proteins targeting the host ubiquitination process during the long-term evolution with host cells, to construct a conducive internal environment for the reproduction of pathogens
[12][13].
L. pneumophila as the pathogen causing pneumonia, also derived numerous effector proteins to modulate host ubiquitination and the most striking example of these to date is the SidE effector family
[14][15][16]. The SidE family contains four highly conserved members SidE, SdeA, SdeB, and SdeC that mediate a noncanonical ubiquitination system to facilitate the optimal
Legionella virulence. While the importance and the inherent understanding of canonical ubiquitination has been known for a long time, the atypical ubiquitination catalyzed by the SidE family shows an unprecedented aspect in this area, which has attracted a lot of attention.
2. SidE Family Effectors Catalyze a Non-Canonical Ubiquitination Process
The non-canonical ubiquitination by SidE family proteins differs from the canonical ubiquitination system in several aspects, including structure characteristics of enzymes, energy consumption and the number of reaction enzymes or steps. Firstly, for structural features, SidE family members are large proteins (approximately 1500 residues), which contain a DUB (deubiquitinase) domain, a PDE (phosphodiesterase) domain, an mART (mono-ADP ribosyltransferase) domain and a C-terminal domain (CTD) (
Figure 1a,b)
[17][18]. Secondly, for the energy source, nicotinamide adenine dinucleotide (NAD
+) is required by the mART domain of SidE, which is a putative catalytic motif typically found in bacterial toxins
[19][20]. Thirdly, this non-canonical ubiquitination is catalyzed only by one protein in an all-in-one mode rather than the three steps mode of canonical ubiquitination. Finally, Arg42 of ubiquitin and primarily a serine residue of substrate are linked by a phosphoribosyl moiety, so this type of ubiquitination is also named PR (phosphoribosyl)-ubiquitination. Recent studies also found that SdeC-mediated PR-ubiquitination also modifies tyrosine residues in host proteins
[21].
Figure 1. Overall structure of SdeA. (a) Domain diagram of SdeA (1–1499), SdeA contains four domains, DUB (blue), PDE (orange-yellow), mART (green) and CTD (golden-yellow), from N-terminus to C-terminus. (b) Two views of overall structure of SdeA (231–1190) colored as in a. (c) Structure of SdeA PDE domain. (d) Structure of SdeA mART domain, α-helical lobe and β-sandwich lobe are marked, and the “plug loop” was also labeled.
2.1. The Structural Features of SidE Family
The SidE family protein contains four domains, including DUB, mART, PDE and CTD domains, and each one has its independent function or regulates another. The DUB domain, comprising ~200 residues in the N-terminus of SidE, was first characterized to have deubiquitinase activity, with a preference for Lys-63 Linked polyubiquitin chains
[22]. The PDE domain spans residues approximately 200–600, which is formed by two lobes: a larger helical core lobe containing the catalytic pocket and a smaller cap lobe covering from the top
[23] (
Figure 1c). Structural comparison revealed that human SAMHD1, the dNTP hydrolase related to innate immune response, is the closest structural homologue in mammals of SdeA
[24]. The SdeA mART domain contains a typical Rossmann fold and shows the conserved characteristics among all known mART toxins in bacteria
[19]. Two-lobe structures constitute the SdeA mART domain, one with an N-terminal α-helical lobe and the other with a C-terminal β-sandwich lobe (
Figure 1d).
2.2. The Novel-Ubiquitination Machinery of the SidE Family
As mentioned above, ubiquitination as an important protein PTM, was well studied for decades
[25]. However, in 2016, SdeA protein in
L. pneumophila was identified to be capable of performing a non-canonical ubiquitination by itself
[20]. In contrast to the conventional ATP-driving E1-E2-E3 cascade (
Figure 2a), the ubiquitination catalyzed by SdeA effector requires NAD
+ as energy
[26]. Overall, it is strikingly different between the three-enzyme systems and the all-in-one ubiquitination machinery SidE. While SidE family protein comprises four domains, only the enzymatic activities of the PDE and mART domain are involved in the ubiquitination process. The SidE ligase machinery was divided into two distinct parts, Ub activation and Ub-substrate ligation, which was catalyzed by the mART and PDE domain respectively (
Figure 2b)
[26].
Figure 2. The mechanism diagram of E1-E2-E3 conventional ubiquitination and SidEs PR-ubiquitination. (a) Ubiquitin activating enzyme E1, ubiquitin conjugation enzyme E2 and ubiquitin ligase E3 are working together for the conventional type of ubiquitination. (b) SidE family proteins could catalyze the whole PR-ubiquitination reaction by itself, mART and PDE domain involved the first and second step respectively. NAM, nicotinamide.
First, the mART domain exhibits ADP-ribosyl transferase activity, using nucleotide cofactor NAD
+ as energy, leading to ADP-ribose group covalently added to Arg42 of Ub forming ADPR-Ub
[26] (
Figure 2b). ADP-ribosylation is also one of the most important types of protein PTMs, discovered in bacterial pathogen
Corynebacterium diphtheria originally and in the eukaryotic cell subsequently, which regulate various cellular processes, including tumorigenesis and DNA repair
[27][28].
Second, the SidE PDE domain recognizes and binds the ADPR-Ub produced by the mART domain, exhibits phosphodiesterase activity to cleave the phosphoanhydride bond in ADPR-Ub and produce phosphoribosylated ubiquitin (PR-Ub)
[23]. Meanwhile, in the presence of a substrate protein, the SdeA PDE domain utilizes a substrate binding cleft (constituted by N404, Q405, M408, L411 and S428), juxtaposed with the catalytic site, to position serines of the substrates for ubiquitination. During the reaction, a transient SdeA H277-PR-Ub intermediate was first formed and subsequently nucleophilic attacked by the OH group of the target serine of the substrate. Finally, PR-Ub was transferred to serine residues in target proteins, with the release of AMP
[29] (
Figure 2b).
3. Activity of the SidE Family Was Strictly Modulated by Many Effectors
3.1. SidJ Interacts with Calmodulin to Modify SdeA
The ubiquitination activity of SdeA has a relatively strong toxic effect on host cells. However, this excessive toxic effect is not conducive to the proliferation of
L. pneumophila. In 2015, the
L. pneumophila effector protein SidJ was found to inhibit the toxicity of SdeA in the host
[30]. In the subsequent study, it was proved that SidJ suppresses the ubiquitination activity of SdeA in vivo
[31]. However, it was still unknown why SidJ can inhibit the activity of SidE family proteins only in the host cell at that time. In the process of exploring this question, calmodulin (CaM), the Ca
2+ binding protein in eukaryotic cells, appears to participate in the regulation of SdeA by interacting with the
L. pneumophila effector protein SidJ. Then, four independent studies revealed that SidJ and calmodulin form a stable complex, catalyzing a distinct PTM to the key catalytic residues of the SidE family protein, turning SidE into the “inactive state” (
Figure 3).
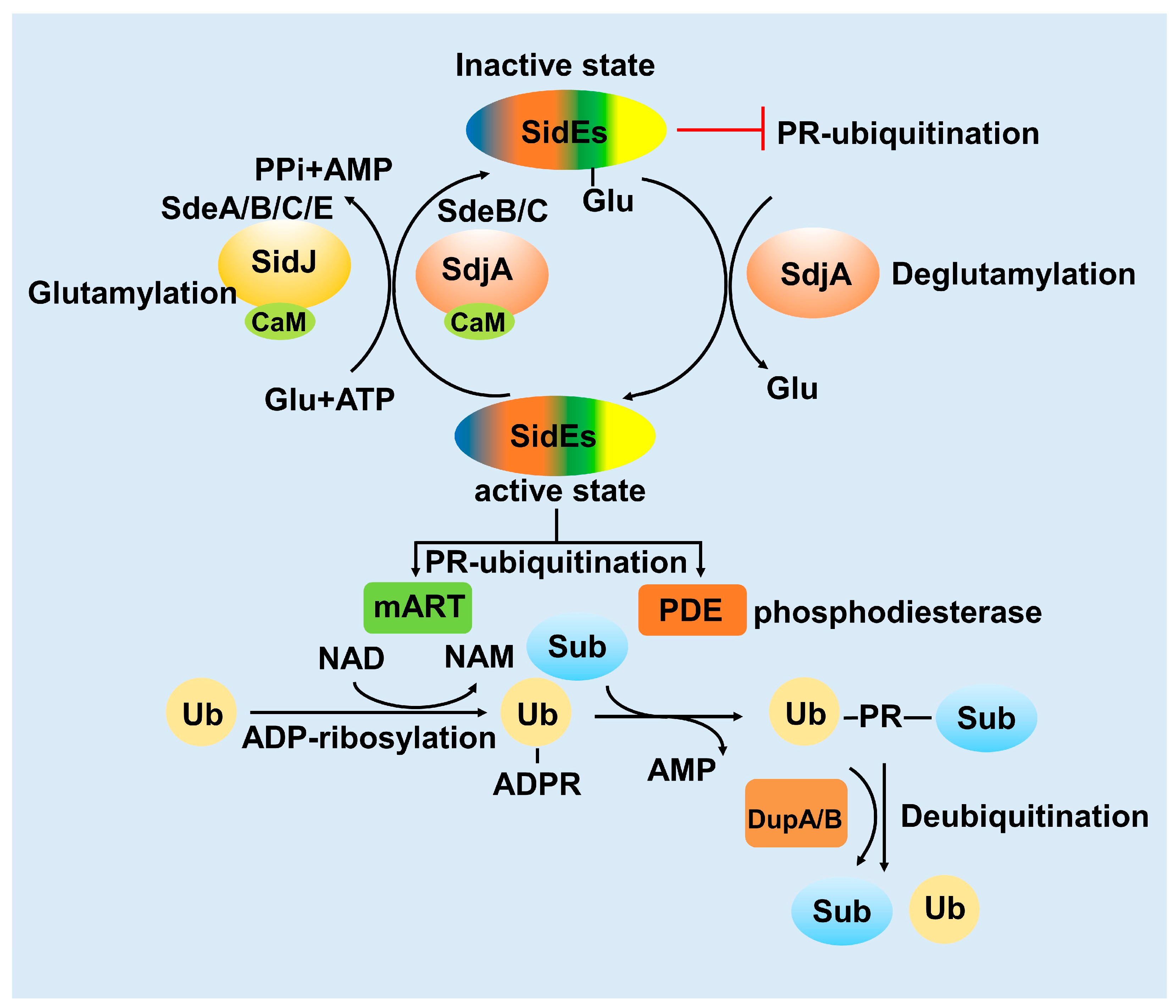
Figure 3. The modulation model of SidE family proteins. SidEs catalyze PR-ubiquitination by their mART and PDE domains. SidJ-CaM and SdjA-CaM mediate glutamylation to inactivate SidEs (SidJ-CaM inactivate SdeA/B/C/E, SdjA-CaM inactivate SdeB/C). The inactivated SidEs-Glu could be reactivated again by SdjA, named deglutamylation. The PR-ubiquitination substrates could be cleaved by DupA/B, releasing substrates and Ub again.
3.2. SdjA Reverses the Glutamylation Modification of SdeA
Remarkably, the modification mode of SidJ-CaM towards SdeA unveils an archetypal example that the pathogenic bacterial effector protein catalyzes glutamylation, modulating the PR-ubiquitination mediated by the SidE family protein in the host cell
[32]. E860 is the key catalytic residue of the SdeA mART domain, which is polyglutamated by the SidJ-CaM complex, indicating that SidJ-CaM displays specificity towards this residue. Furthermore, Vincent et al., solved the cryo-EM structure of SdeA-SidJ-CaM intermediate complexes, proving that the kinase-like site of SidJ adenylates the active-site Glu in SidE in the presence of ATP and Mg
2+, forming a stable intermediate complex. At the same time, the insertion loop in the active site of the SidJ kinase domain accommodates the donor Glu near the acyl-adenylates site, facilitating the reaction of glutamylation
[33].
3.3. DupA and DupB Function as Deubiquitinases for PR-Ubiquitination
Conventional ubiquitination is a reversible process, the substrate of ubiquitination can be re-cleaved into ubiquitin and substrate by deubiquitinating enzymes
[34]. The novel ubiquitination mediated by the SidE-effector proteins involves the formation of thioester bonds between substrates serine hydroxyl and ubiquitin
[26]. In this process, the PDE domain of SidE can cleave ADPR-Ub to generate AMP and PR-Ub in the absence of substrates
[26].
4. Multiple Host Proteins Targeted by SidE Family Effectors
4.1. The Effects of SidE Family Proteins on Endoplasmic Reticulum
L. pneumophila is an intravacuolar pathogen, utilizing a type IVB secretion system (T4SS) to translocate effector proteins into the host cytosol to establish LCV, an endoplasmic reticulum (ER)-associated organelle
[35][36]. However, these bacterial effector proteins are unable to form an LCV themselves which means that they need to make use of the substances or protein substrates in the host cell for this process. Endoplasmic reticulum is a continuous omental system, a cystic, vesicular, and tubular structure organelle formed by a single membrane, which is in charge of the production and movement of proteins and other molecules
[37]. Endoplasmic reticulum could be classified into the perinuclear, ribosome-associated ER sheet and tubular ER and the tubular ER is a vast network of cylinders that are enriched with some structural ER membrane proteins, such as reticulon family proteins
[38].
4.2. The Effects of SidE Family Proteins on the Golgi Complex
In the early stage of infection,
L. pneumophila exploits effectors, such as SidE family proteins, to manipulate Rab1 and other ER-related proteins to intercept the versicles to the LCV
[39]. Actually, the downstream process after ER vesicles fusing to the Golgi complex is also disturbed. Recently, the relationship between PR-ubiquitination and the Golgi complex has received increasing attention. The Golgi complex, the cystic structure apparatus formed by the elementary membrane, is the component of the eukaryotic endomembrane system, which functions as the PTM factory for protein modification, classification and translocation
[40]. The vesicles from the endoplasmic reticulum could fuse with the Golgi membrane, delivering the inclusions into the Golgi lumen, where the newly synthesized peptide chains continue to complete their modification and packing
[41].