2. Transcriptional and Translational Control of Protein Synthesis
The biosynthesis of proteins in cells and tissues is controlled at both the transcriptional and translational levels, but the global mechanism that allows them to carry out their functions does not exclusively involve the mere number of proteins
[2] and depends on the different steps of control.
2.1. Genetic and Epigenetic Control
Genetic control is affected by genomic or genic alteration, including modifications to the chromosome structures and gene sequences. Basically, the alteration leads to the production of either normal or altered proteins both in quality and/or amount, also by impairment between the codifying and controlling sequences. The perturbations of the epigenetic control change the heritable state of gene expression and chromatin organization, without modifying nucleotide sequences (i.e., histone modifications, DNA methylation and/or acetylation, and loss of imprinting-LOI)
[3][4]. As a therapeutic approach, the induction of the re-expression of epigenetically silenced TSGs could provide a means to suppress cancer outcome and to increase responsiveness to new drugs
[3].
2.2. Translational Control
Translational control guarantees the correct correspondence between the mRNA code and protein sequence. A ribosome-associated protein quality control implements a series of “protocols” to allow the biogenesis of functional proteins
[5][6].
3. Post-Translational Modifications
The process of protein synthesis must be integrated and balanced. One of the most-studied mechanisms of protein modulation is driven by a growing amount of moiety acting in the process described as post-translational modification. Post-translational modifications (PTMs) are dynamic to allow the cell to rapidly counteract extracellular stimuli or insults and rapidly restore the basic condition to maintain homeostasis. In general, since most PTMs are reversible, they act as a switch from the normal to active state of the target and/or vice versa. In this light, post-translational machinery is essential in preventing the development of cancer. TSGs activation lies in the modulation of various PTM states of the TSGs themselves and their transcriptional and non-transcriptional targets and effectors for cell survival and proliferation control
[7][8]
Along with single regulatory modification, different PTMs can act both in parallel and sequentially
[9][10][11][12]. The combining of a myriad of single and/or multiple spatially and timely orchestrated modifications can govern a target protein’s life span and activity, their distribution within the cell compartments and consequently the protein interaction rate, ultimately leading to the appropriate outcome
[13][14].
So far, following the canonical biological approach and the newest growing web resources, researchers can count a few hundred kinds of modifications occurring at 15 of the 20 amino acids present in protein sequences and tens of thousands of modification sites have been identified across the whole proteome
[15]. The proteome-wide analysis of single PTMs and their crosstalk is now possible by using the bio-informatics approach
[16][17][18]
3.1. Types of PTMs
Conceptually the PTM machinery consists of “writers”, “erasers”, and “readers”. Writers (i.e., kinases, methyltransferases, and acetyltransferases) mediate the transfer of a modifying group of different complexity (from a chemical group to a complex polypeptide chain) onto protein substrates. Erasers (i.e., phosphatases, demethylases, and deacetylases) are responsible for the reversibility of the PTMs by mediating their removal from protein substrates. Readers act between writers and erasers since they detect and decodify the signaling written on labeled target proteins leading to protein activation/inactivation or further modifications.
Basically, PTMs can be divided into two large groups: the first provides for the addiction of chemical groups—with a wide range of complexity—at specific amino acids; the second involves the formation of a covalent linkage to a monomeric or polymeric protein moiety.
Along with phosphorylation, as the mostly diffused PTM, the first group includes acetylation, methylation, glycosylation, and some others. The second mostly observed PTM, ubiquitination, is included in the second group that counts also on SUMOylation and NEDDylation. According to different substrates and context, phosphorylation can determine the activation state of enzymes and receptors, also controlling their stability, interaction, and downstream pathways
[19]. Ubiquitination acts in the modulation of protein stability, and manages fundamental biological processes, such as cell cycle, immune responses, apoptosis, and cancer. Albeit structurally similar to ubiquitination, SUMOylation pathways do not induce target degradation and have a pivotal role in the preservation of genomic integrity, transcriptional regulation of gene expression, and signal transduction
[7].
The mechanism of modification for the first group is a single or a few-step enzymatic reaction. The process engaged for the second group of modification is quite complex and deserves more details.
3.2. PTMs Crosstalk
PTMs crosstalk may occur both intra- and interprotein
[20][21]. It takes place on the same residue, as well as at proximal or distal sites in a sequential mechanism in which one depends on the other
[17], and it is observed between the same or different types of PTMs
[14][22][23][24][25].
The interaction between PTMs can be a positive (or activating) crosstalk, and a negative (or inactivating) crosstalk, based on both direct and indirect competition
[14][23][26]. Thus, PTM perturbation may possibly induce disease development and progression, and especially induce a predisposition to carcinogenesis
[27].
3.3. PTMs in the Control of Oncogenes and TSGs Activity
Among oncogenes and TSGs, researchers will focus on four of the most important and surely impactful players in controlling cell homeostasis and health (Table 1), namely:
Table 1. The four homeostasis knights’ genes.
Functional description, types of oncogenic alteration and the main cancer sites. The Cancer Genome Atlas (TCGA) was accessed and profiled through cBioPortal (http://cbioportal.org/ (accessed on 13 October 2022). Data were from the TCGA Pan-Cancer Atlas project.
- -
-
RAS proteins as molecules integrating receptor signaling along pathways that control cellular growth;
- -
-
c-MYC as a transcription factor acting as a key player in the development of many human cancers;
- -
-
RB, as the first tumor suppressor gene identified;
- -
-
p53, as the master gene in the preservation of genetic integrity, so far called “the guardian of the genome”.
4. p53
p53 represents a paradigmatic example of PTM crosstalk-mediated control. Even if it was originally described as a tumor suppressor, up until today researchers recognize p53 as the more powerful controller of cellular homeostasis, acting through cell cycle arrest, apoptosis, DNA repair, cellular senescence, and other metabolic processes
[28][29][30][31]. That is why currently, researchers name p53 as the “guardian of the cell”
[32], rather than the “guardian of the genome” as they previously did
[33]. Without considering the complex genetic and epigenetic control, reversible and irreversible PTMs surely represent the smartest way to dynamically modulate the wide potential of p53. p53 acts both as a monomeric and homotetrameric complex, exerting transcriptional and non-transcriptional functions, respectively
[32][34]. The p53 structure is well-known and consists in a multidomain protein made of six functional domains.
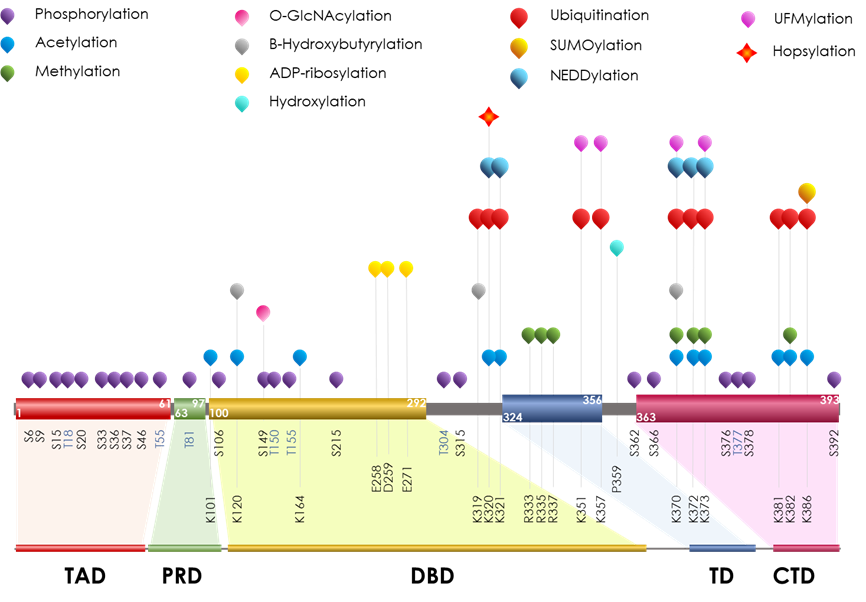
Figure 1. Schematic representation of p53 protein domains with the main PTMs. p53 is a 393 aa long protein whose functional domains are: N-terminal Trans Activation Domain (TAD, aa 1-61), Proline-Rich Domain (PRD, aa 64-92), central DNA-Binding Domain (DBD, aa 101-292), Tetramerization Domain (TD, aa 325–356), and an intrinsically disordered C-Terminal Domain (CTD, aa 363-393). Given its regulatory function, the CTD is strongly affected by PTMs. The specific PTMs are functional to the role of the domain involved. All the six p53 domains and relative linker sequences undergo PTMs: phosphorylation, acetylation, methylation, O-GlcNAcylation, ADP-ribosylation, hydroxylation, β-hydroxybutyrylation, ubiquitination, SUMOylation, NEDDylation, UFMylation and Hopsylation are reported and labeled as indicated in the legend. The localization and the modified aas are reported. The higher PTM frequency in the last third of sequence implies that the picture is not drawn to scale. The real size of each domain is shown at the bottom.
The characterization of each functional domain, with the modification sites within, was widely and deeply studied in past years and allowed researchers to consider PTM crosstalk as a key mechanism to balance p53 functional output [35].
Recently, other than SUMO and NEDD, a novel ubiquitin-like protein has been identified. HOPS/TMUB1 (Hepatocyte Odd Protein Shuttling/Trans Membrane Ubiquitin-Like Protein 1, hereafter referred to as HOPS) [36] is able to impact the control of p53 stability by inhibiting its recruitment to the proteasome and lengthening its half-life [37]. Firstly, HOPS has been shown to interact and control the stabilization and the nucleolar localization of the tumor suppressor p19Arf, also acting in its functional binding to the onco-suppressor nucleophosmin (NPM) [38]. Given the nature of HOPS interaction to p53 and other targets, it has been referred as HOPSylation, since it acts through the HOPS UBL domain. HOPSylation appears to occur at Lys320 and is reported to be engaged in ubiquitination, NEDDylation and acetylation, and is involved in the management of p53 cellular distribution. Functionally, HOPS interaction to p53 leads to the control of its apoptotic potential upon DNA damaging stresses, impairing p53 localization as a critical event for specific functional outcomes [37][39].
Emerging research shows that p53 can be a substrate for UFMylation. Analogously to other UBLs, the ubiquitin-fold modifier (UFM) is a recently identified ubiquitin-like protein [40][41]. UFMylation occurs at CTD both at lysine sites, and is reported to be interested in other Ub or UBL conjugations (K370 and K373), and two novel ones (K351 and K357). Since p53 UFMylation and other ubiquitin-like modifications compete for the same lysine sites in p53, they act in modulating the stability, by interfering with p53 ubiquitination-mediated proteasomal degradation and possibly in controlling other functional outcomes [42].
5. Conclusions
The fine tuning of protein expression and function is a key step in cellular homeostasis and healthy state preservation. Alterations affecting such events including protein biosynthesis and turnover are at the origin of a wide spectrum of pathologies and diseases, including cancer.
The PTMs represent a powerful mechanism to rapidly modulate specific protein amounts in cells, thus controlling their localization, function, and intracellular dislocation. Since they play a critical role in the control of cellular homeostasis and there are negative outcomes for the alteration of proper protein modifications, their role in cancer progression is definitively assessed.
For this purpose, in the past years, more significant evidence has been produced to suggest their use in new therapeutical approaches in the field of oncologic diseases. So far, a number of new molecules (i.e., ubiquitination pathway inhibitors/modulators) have been developed for clinical use and are now available in current medical practice.