Lateral flow immunoassay (LFI) is used in many applications, particularly in the field of food safety testing, including various pathogens, drug residues, food additives and other illegal additives, etc. The basic principle of LFI testing is the flow of a liquid test sample, with antibody-containing strips interacting with the analyte and discriminating the results by the accumulation of chromogenic substances. Although LFI is simple, inexpensive and portable, the results obtained are qualitative or at best semi-quantitative, which limits the wider use of LFI.
1. Fluorescence Resonance Energy Transfer (FRET)
FRET technology is based on energy transfer between two fluorophores without radiative excitation. Luminescent nanoparticles (NPs) can act as both donor and receptor emitters. The first fluorescent molecular material of the donor receives the energy and is excited, then converted to the second fluorescent molecular material of the acceptor. Thus, the molecular donor provides energy for the luminescent molecular material, and the receptor required for the luminescent molecular material to receive energy is located in the vicinity of 1~10 nm
[1] as shown in
Figure 1.
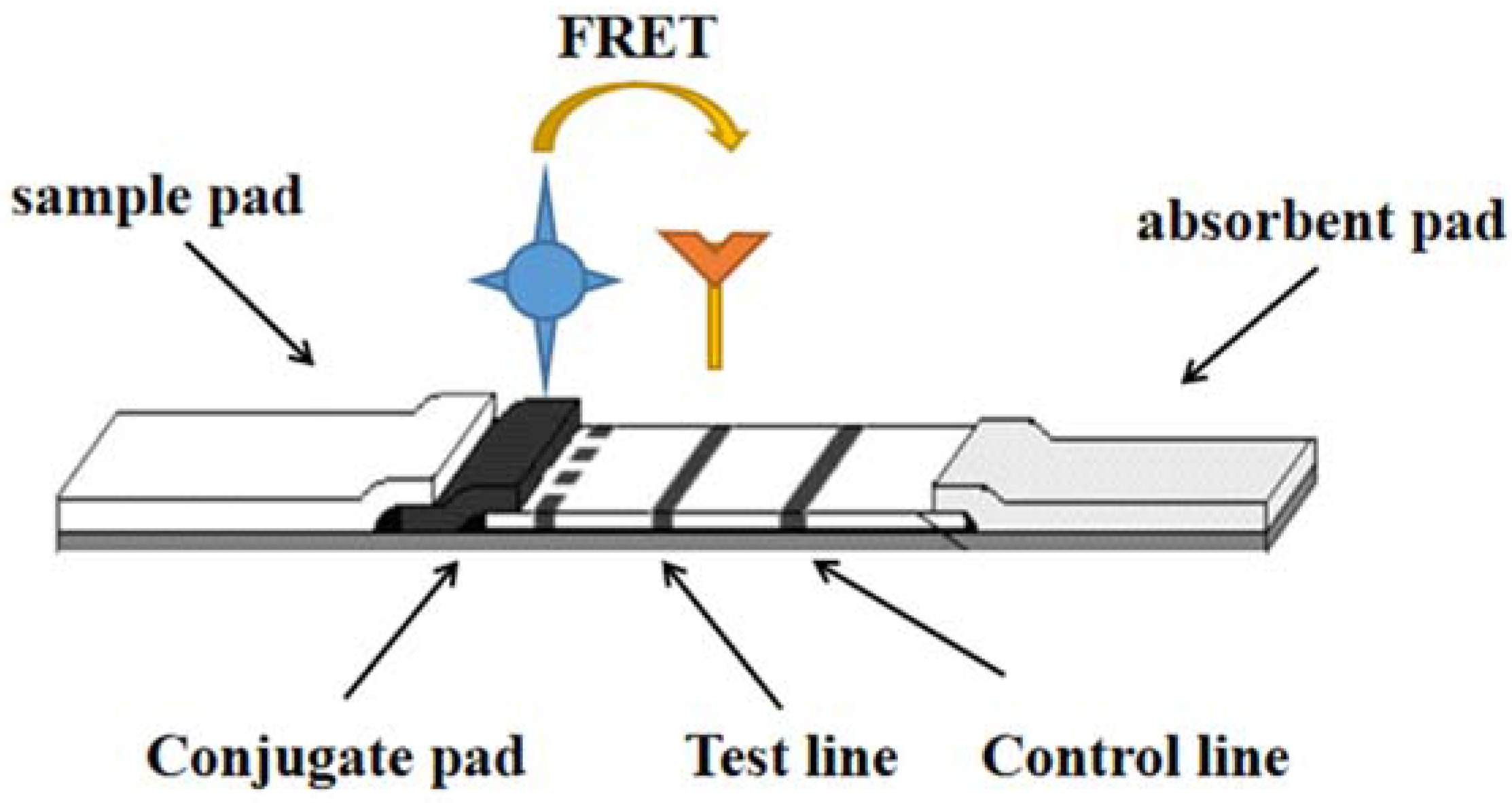
Figure 1. One of the detection principles of LFI–FRET.
Tonsomboon
[2] developed a fluorescent electrospinning fiber membrane that could bring mercury contamination to sub-ppb level within 60 s. The key to this user-friendly device lies in the development of a novel, organic NF06 mercury dye, which by exploiting the FRET mechanism, fluorescence enhancement-”turn-on” favorable results are presented, with a high Stokes shift (248 nm). The NF06 sensor was immobilized on cellulose acetate (CA)/polycaprolactone (PCL), and its surface chemistry was found to effectively enhance FRET operation.
Bisulfate is essential in the body’s digestive function. Therefore, fast and simple detection of bisulfite is critical. Wen
[3] reported a fluorescent probe (CPT) based on bisulfite (HSO
3−) with a high Stokes shift (162 nm). HSO
3- can be quantitatively detected by this probe with a minimum detection limit of 45 nm, and it is highly specific compared to other commonly used anions and biothiols. The sensing mechanism is nucleophilic addition reaction and is corroborated by high resolution mass spectrometry. The probe paper is simple to make and easy to use.
Mycotoxins are toxic to animals, plants and humans, because mycotoxins survive thermal processing and hydrolysis processes, so detection of mycotoxins is critical. Oh
[4] designed a FRET-based system for the detection of mycotoxins using antibody-antigen binding simultaneously, which can detect mycotoxins faster and more conveniently than other detection systems. In addition, the method can be attached to a nitrocellulose membrane, which allows the matrix effect in the sample to be more effectively counteracted, and can be used for the fluorescence measurement of Hematoxin A (OTA) in coffee samples. The system can complete the detection of OTA in 30 min and its detection limit (LOD) is 0.64 ng/mL. In the measurement results in the actually present samples, its LOD is 0.88 ng/mL, overcoming the matrix effect caused by the chromatographic properties of membrane capillary forces. Based on this, scholars can argue that the new system developed can be used as a powerful detection tool that can monitor food quality and environmental conditions, as well as sensitive diagnosis of harmful substances such as chemical reagents and microbial toxins.
2. Aptamer
Aptamers are oligonucleotide sequences with high specificity and a special affinity for target substances obtained by screening random oligonucleotide libraries by SELEX techniques. Compared with antibodies, aptamers have obvious non-immunogenicity, and they are low cost, high reproducibility, good stability and easily modify a variety of chemical groups. As shown in Figure 2.
Figure 2. The detection technology of LFI–Aptamer.
Zhao
[5] developed and designed a lateral flow adaptive sensor for detecting the aflatoxin B based on the application of the fluorescent dye Cy5 as an aptamer marker and competition between aflatoxin B and aptamer complementary DNA. This method is the first time that the aptamer complementary strand was used as the detection line (T line) for aflatoxin type-B.
Researchers have designed a sandwich transverse flow strip detection method (LFSA). The content of rongalite in food was detected by AuNPs modified with gold nanoparticles
[6]. More specifically, the biotin-labeled first-level A09 aptamer is fixed to the streptavidin envelope, and the second-level B09 aptamer binds to AuNPs as a capturing probe and a signaling probe, respectively. By recording the color changes in the LFSA control and test lines, the system can successfully directly detect aluminates in food samples at low concentrations of 1 μg/mL.
3. Surface Enhanced Raman Spectroscopy (SERS)
SERS is a new technology based on molecular detection and characterization. It depends on the enhanced Raman scattering of molecules near the hot spots of a SERS active surface. Spectroscopy or Raman scattering has become a non-destructive testing technology. Its excellent characteristics are sensitivity, rapidity, accuracy, fingerprint information and so on. Raman scattering is an inelastic scattering, which is a kind of scattering between media and photons. The spectrum can reflect the structural characteristics of the investigated samples. For heavy metals such as gold and silver, the enhancement effect of rough surface is called the SERS effect. Wang et al.
[7], based on the working principle, strategy and point-of-care testing (POCT) application of the SERS test strip, designed horizontal and vertical flow test strips. SERS-based POCT and field analysis test strips need to be equipped with a portable high-power Raman spectrometer equipped with a high-power narrow-spectrum Raman bandwidth laser, so as to transform laboratory analysis into practical analysis applications, as shown in
Figure 3.
Figure 3. The detection technology of LFI–SERS.
β-Conglycinin is an important nutrient substance in soybeans, and eating too much may cause food allergies. Xi et al.
[8] studied a sandwich lateral flow immunochromatography detection strip, which was mainly established by high-affinity mouse monoclonal antibody (3D11 mAb), to quickly and accurately detect soybean allergen β-Conglycinin. 3D11 mAb binds to a rabbit polyclonal antibody to form a band. The LOD of this test strip was 1 μg/mL. In addition, the quantitative detection of β-glycine was achieved by using the chemical molecules p-aminothiophene and colloidal gold as Raman-enhanced signals. This study determined that β-glycine concentrations work between 160 ng/mL and 100 μg/mL.
Based on the cheap, simple, fast and portable LFI strip and SERS technology, an ultra-sensitive detection method for antibiotics in milk was established. Immunoprobes are prepared by colloidal gold (AuNPs) with anti-neomycin (new monoclonal antibody) and coating competition between the Raman antiprobe molecule 4-aminothiol (patient) and free NEO and antigen (NEO-OVA), resulting in changes in the number of immunoprobes immobilized on the paper-based material. To quantify NEO, the Raman intensity of PATP on the lateral flow test line needs to be determined. The LOD and semi-inhibitory concentration values were 0.216 pg/mL and 0.04 ng/mL, respectively. This method does not cross react (CR) with other compounds and has high specificity. Recoveries of milk added to new samples ranged from 89.7% to 105.6%, and a relative standard deviation (RSD) of 2.4–5.3% (n = 3). The results showed that the proposed method has high sensitivity, specificity and stability, and can detect various antibiotic residues added in milk samples
[9].
4. Quantum Dots (QDs)
“Quantum dots (QDs)”, conjugated with high-fluorescence probes, are essential for detection and long-term fluorescence imaging of some kinds of cellular processes
[10][11]. QD is one of the most promising immunochromatography markers. The quantum dots used have different diameters (1–100 nm); it is possible to generate luminescence covering the entire visible region through a single near ultraviolet excitation source
[12][13]. It is worth noting that each quantum dot is characterized by a narrow emission spectrum. Hence, quantum dots can be used as novel molecular imaging probes, and are also considered a remarkable reagent against viral infection. In addition, collaboration on potentially biocompatible vectors could help with interdisciplinary research and enable clinical approaches to combat viruses, so the function of QDs can be as carriers/labeling drugs or drug carriers. As shown in
Figure 4. Multicolor QDs have been used in a variety of fields, including biological imaging
[14], fluorescence-based ELISA
[15][16], flow cytometry
[17], etc.
Figure 4. The detection technology of LFI–QDs.
5. Electrochemical Sensor Strips
While significant advances have been made in sensing technology, the design, installation, and application of self-test equipment remains limited to users and specialized laboratories. Decentralized analytical equipment is very helpful for people’s production and life, so that people can easily analyze, evaluate and predict various environmental and food samples, which can greatly improve people’s living conditions, especially for people living in remote or resource-poor areas
[18].
Ding
[19] outlined the role of paper-based potentiometric and voltammetry in heavy metal detection. Paper-based 3D sensors are made by bonding and folding different layers of a piece of paper, and the objects of its use depend on the type of sensor, for example it can be used for chromatography, electrochemistry and colorimetric processes
[20]. Nguyen
[21] introduces the latest developments in high-sensitivity electrochemical paper-based lateral-flow assays (LFAs), which has many unique advantages because of the versatility and simple optical setup of electrochemical LFA.
A paper-based 3D sensor is bonded and folded from different layers of paper. Because it combines multiple preparation means, integrates different sensor components, and connects two detection methods, it is more user-friendly in small collections. The object of use of a paper sensor depends on the type of sensor, e.g., it can be used for chromatography, electrochemistry and colorimetric processes. In addition, in recent years, these sensors have been studied extensively and applied in many aspects, such as food safety detection, disease diacrisis and environmental monitoring. Another study describes a paper-based electrochemical sensor based on a thiol-terminated poly(2-methacryloyloxyethylphosphorylcholine) (PMPC-SH) that self-assembles on a screen printed electrode (SPE) modified with gold nanoparticles
[22]
6. Biosensor Strips
Biosensors are small devices that convert the recognition of biomolecules (DNA/RNA, antibodies, proteins, whole cells, etc.) into signals (electrochemical, optical, piezoelectric, nanomachinical, mass sensitive, etc.) and are considered powerful tools for detecting target molecules or products in environmental and food samples. Biosensors are divided into many types, among which fluorescence and colorimetric sensors are relatively simple and intuitive to observe the number of samples, and can determine the sequence of target DNA through fluorescence. Biosensors play an important role in food detection, with many advantages such as portability, specificity, low cost and short time, which can simplify and automate detection methods
[23]. As shown in
Figure 5.
Figure 5. The detection technology of LFI–Biosensor.
A phage-based dipstick biosensor has now been developed and tested to detect a variety of foodborne pathogens in food samples
[24]. A four-target nitrocellulose-based lateral flow immunoassay biosensor has also been developed in a form of dry reagent strip to interpret double-labeled double-stranded amplicons in thermally stable triple-ring-mediated isothermal amplification analyses
[25].
Immunological approaches are among the tools that have the opportunity to develop easy-to-manipulate biosensors. Vitamin B12 in food is one such analyte that needs to be urgently tested. The study describes a dipstick-based immunochemiluminescent (immuno-CL) biosensor for detecting vitamin B(12) in energy-replenishing beverages
[26]. This is a form of direct competition that involves fixing vitamin B(12) antibodies to nitrocellulose membranes (NC) and then treating them with vitamin B(12) and vitamin B(12) alkaline phosphatase complexes to promote competitive binding. After system optimization, its detection limit is 1 ng mL
−1. The study performed a sensitive, accurate and rapid screening of vitamin B (12) in a beverage sample capable of replenishing energy. Immune CL-based dipstick technology is appropriate for detecting a variety of targets in food and environmental samples.