Micro-computed tomography (micro-CT) is a promising novel medical imaging modality that allows for non-destructive volumetric imaging of surgical tissue specimens at high spatial resolution.
1. Introduction
Micro-computed tomography (micro-CT) constitutes a novel non-destructive ex vivo medical imaging modality that enables volumetric imaging of intact surgical lung tissue specimens at high spatial resolution and is thereby capable of providing microscopic insight into alveolar anatomy and pathology
[1]. Due to these favourable properties, micro-CT has been used in research settings and helped to advance the understanding of lung histopathology
[2]. However, the technology also has significant potential for clinical application
[3].
Similar to CT imaging in radiology, micro-CT imaging relies on an X-ray source and detector assembly in combination with computational image reconstruction algorithms to acquire a series of 2D planar, cross-sectional digital images of the imaging target, which can be computationally processed to generate 3D volumetric models
[4]. In contrast to the CT devices used in radiology for in vivo imaging, micro-CT devices used for ex vivo imaging operate at a much smaller scale and with a higher radiation dose, which allows for the acquisition of digital images of lung tissue specimens at micrometre resolution, thereby enabling the diagnosis of pulmonary diseases that manifest itself at the microanatomic scale
[5][6]. Within the hospital, micro-CT devices are usually situated in the pathology department, where the surgical tissue specimens are prepared and subsequently imaged with the assistance of specialized medical imaging engineers and technicians
[7][8][9][10]. Given its favourable imaging characteristics and rapid image acquisition speed, micro-CT has great potential for clinical applications across a wide spectrum of pulmonary pathologies, including chronic restrictive and obstructive lung disease as well as lung cancer
[6][11][12].
2. Micro-CT in Pulmonary Diseases Diagnosis
Micro-CT has been used for the quantitative assessment of the pulmonary microanatomy, and in the context of a wide range of lung diseases. The imaging modality has been successfully utilized for high-resolution 3D visualization and morphometric analysis of lung lobules, capillary alveoli, and terminal bronchioles, providing the ability to measure pathological alterations in restrictive and obstructive lung diseases. Determination of positive surgical margins in lung cancer and understanding transplantation-related disorders are two further common clinical applications of micro-CT imaging [1][13][14] (Figure 1).
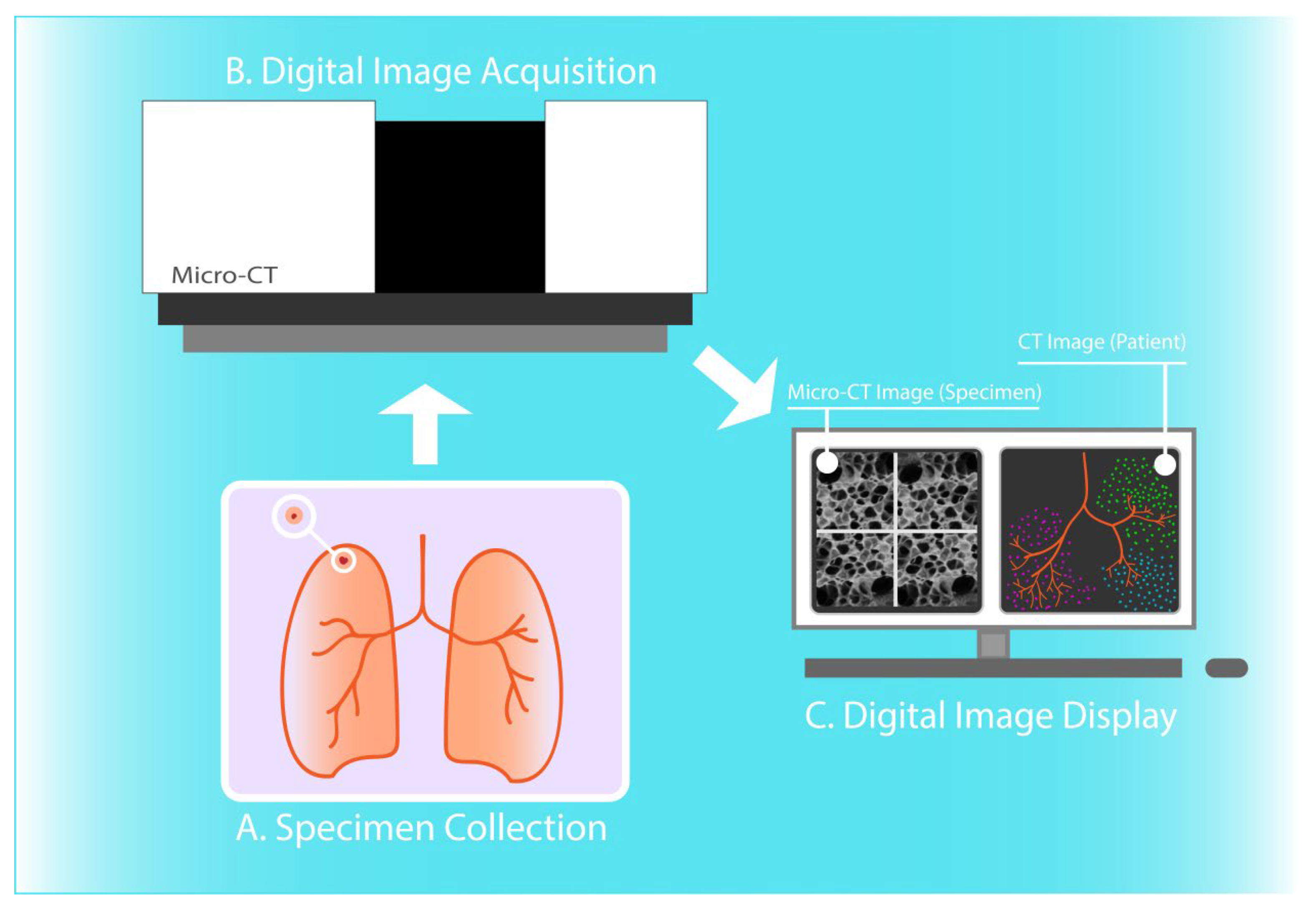
Figure 1. Micro-CT imaging of human lung tissue specimens. Resected lung tissue specimen (A) is scanned through micro-computed tomography (micro-CT) scanner (B) and 3D images of high spatial resolution are generated after reconstruction (C). Herein, 3D micro-CT images of terminal bronchioles and pulmonary acini are displayed (C left), in comparison with illustrated CT-derived low attenuation cluster analysis of the airway tree (C right).
2.1. Visualization and Quantitative Analysis of the Lung Microanatomy in Chronic Obstructive and Restrictive Pulmonary Disease
In some application areas, micro-CT has enabled novel insights into the 3D structural alterations in pathological lung specimens. In chronic obstructive pulmonary disease (COPD), for example, micro-CT imaging has made it possible to quantify the loss of terminal bronchioles in early-stage COPD, which has been proposed to pave the way for the emphysematous destruction of the alveoli
[15][16]. These alterations are not visible with thoracic multi-detector CT scans used in radiology and understanding these 3D changes at the microscopic level could expand the knowledge of the pathogenesis of pulmonary emphysema
[17]. Micro-CT can also be used to acquire images and generate 3D models of the terminal airways and alveoli, allowing the performance of a “virtual bronchoscopy” through an alveolar duct
[18]. The produced 3D-imaging data sets acquired ex vivo in pathology could be used in combination with routine high resolution in vivo CT imaging in radiology, facilitating volumetric measurements of tissues from the meter to the micrometre length scale
[19]. In the future, the ability of micro-CT to identify pathological terminal and preterminal bronchioles through their anatomical relationship with emerging alveoli in (centrilobular or panlobular) pulmonary emphysema, could make it possible to correlate phenotypic with genetic profiles of patients with COPD and thereby allow a more targeted therapeutic approach
[17][20][21].
Furthermore, micro-CT imaging can be used along with micro Optical Coherence Tomography (micro-OCT), CT imaging, and slide microscopy to comprehensively assess pathological changes in IPF
[22][23][24]. Micro-CT is capable of detecting fibrosis, even in areas of the lung where CT yields normal findings
[25]. Micro-CT-scanned lung samples with minimal pulmonary fibrosis were found to have a significantly lower number of terminal bronchioles when compared to normal control specimens
[26][27]. Hence, micro-CT imaging could contribute to the understanding of the pathological process of lung fibrosis, by visualizing structures, such as fibroblast foci, and thereby locate novel therapeutic target sites
[28]. Moreover, micro-CT has been utilized to determine disease severity across different lung sites, since fibrosis is known to affect lungs with IPF heterogeneously, which contributes significantly to the investigation of gene expression discrepancies among lung samples at a different stage of fibrosis
[29]. Ultimately, a potential future in vivo use of micro-CT in humans could allow earlier diagnosis of fibrotic lung diseases in patients, and could supplement in vivo microscopy imaging modalities such as micro-OCT
[23][25][30].
2.2. Non-Destructive Imaging of Surgical Specimens for Lung Cancer Diagnosis
Micro-CT allows non-destructive 3D visualization and assessment of lung specimens routinely prepared in pathology, including fresh-frozen tissues as well as formalin-fixed, paraffin-embedded tissue blocks
[31][32]. The technology provides 3D spatial tissue context beyond the imaging plane of a physical tissue section specimen evaluated by optical light microscopy and makes it possible to perform micro-structural analysis of relatively large tissue volumes with high diagnostic accuracy, approaching and potentially even surpassing conventional histology
[1][21][33].
Micro-CT has also been employed successfully for the study and diagnosis of lung cancer. In particular, the technology facilitates the identification of lymphatic heterogeneity between normal and malignant lung tissues, giving insight into the lymphatic metastatic spread in lung cancer
[34]. Additionally, micro-CT can identify alveolar walls in lung cancer areas, with comparable accuracy to conventional histopathologic analysis using optical microscopy of hematoxylin and eosin (H&E) stained tissue section specimens. Therefore, the implementation of micro-CT for ex vivo or in vivo lung imaging could help clinicians circumvent the required preparation of histopathological slides, potentially reducing costs and processing time, as well as the need for diagnostic bronchoscopic or surgical lung biopsies
[35]. Micro-CT scanning of fresh surgical lung specimens has already been studied by Troschel et al. and was found to be feasible and potently compatible with the intraoperative setting
[36].
Apart from the ability to evaluate surgical margins and lesion size, high-resolution whole-lesion morphologic acquisition could also supplement slide-based histopathology diagnosis, for example by directing the pathologist toward histology sections that are most likely to influence intraoperative decision-making. It should also be noted that conventional histology sectioning could miss significant histopathologic features due to sparse sampling of the specimen. Micro-CT could also expedite the analysis, especially when performed after resection of specimens with metallic fiducial markers placed preoperatively. Optimization of the parameters affecting the perioperative use of micro-CT as well as larger scale studies could lead to the integration of micro-CT into the clinical workflow of lung cancer resection, which could significantly bolster intraoperative decision-making.
2.3. Evaluation of Lung Allograft Specimens upon Transplant Rejection or Prior to Transplantation
Interestingly, micro-CT has been also proposed as a tool for the investigation of transplantation-related disease characteristics and for the selection of candidate lung transplants. Firstly, micro-CT can effectively quantify terminal bronchioles and complement histopathology in the identification of pathological alterations in BOS and RAS
[37]. Also, in lung contusion and infection cases, micro-CT examination has revealed dense filling of the alveoli
[14]. Similarly, Verleden et al. combined the findings from 18F-FDG PET/CT and micro-CT scanning to show that regions with high SUVmax intensity demonstrate end-stage lung fibrosis, whereas low SUVmax corresponds to normal lung tissue without evidence of inflammation or fibrosis
[38]. These findings provide deeper insight into the mechanisms underlying lung allograft dysfunction phenotypes. Additionally, in another study by Verleden et al. parenchymal alterations consistent with emphysema could be identified only via micro-CT and not via gross slice or macroscopic CT examination
[14]. Therefore, micro-CT could assess and differentiate the cause of rejection in lung transplantation and could be potentially useful in estimating prognosis in redo transplantation candidates. Ultimately, micro-CT could complement the established strict criteria to select suitable donor lungs by measuring the extent of underlying lung injury, which could increase the quality and quantity of lung allografts.
2.4. Assessing COVID-19-Related Alterations of Lung Tissue
The emergence of severe acute respiratory syndrome coronavirus 2 (SARS-CoV-2) causing COVID-19 has resulted in hundreds of thousands of casualties worldwide, thereby prioritizing the COVID-19-related research for the rationalization of new treatment strategies. Micro-CT could emerge as another tool in researchers’ arsenal in studying the pathogenesis of the disease and assessing disease severity, as demonstrated in two recent preclinical animal-based studies that investigated the inflammatory response and the extent of lung injury induced by SARS-CoV-2 infection
[39][40]. Utilization of micro-CT for ex vivo imaging of infected human lungs could contribute to the expansion of the knowledge of the underlying pathogenesis, the discovery of novel imaging biomarkers, and the development of novel therapeutic approaches for preventing or alleviating lung tissue damage upon SARS-CoV-2 infection.
2.5. Example of a Potential Future Micro-CT Application in Lung Cancer Management
Out of the aforementioned applications of micro-CT scanning, its potential involvement in perioperative lung cancer management could be more extensively described. Specifically, besides the perioperative evaluation of tumour size, location, and resection margins within resection specimens, micro-CT could also facilitate identification and quantification of tumour spread through air spaces (STAS), all without compromising specimen integrity. The concept of STAS has first been described in 2008 as a negative prognostic indicator in lung adenocarcinoma
[41] and constitutes a post-surgical pathological diagnosis, officially recognized in 2015 as a pattern of invasion by the World Health Organization classification
[42]. Due to the poor postoperative outcomes for STAS-positive non-small-cell lung cancer surgeries
[43][44], recent research in the field has been orientated towards the association of STAS with preoperative CT features
[45][46][47]. Μicro-CT emerges as a potent alternative technology for obtaining volumetric measurements of the tumour and its three-dimensional micro-environment to inform and guide clinical decision making (i.e., type of surgery performed and perioperative management) and thus has the potential to revolutionize thoracic surgical oncology.