Dopamine regulates emotional behaviors, including rewarding and aversive behaviors, through the mesolimbic dopaminergic pathway, which projects dopamine neurons from the ventral tegmental area to the nucleus accumbens (NAc). Protein phosphorylation is critical for intracellular signaling pathways and physiological functions, which are regulated by neurotransmitters in the brain. Previous studies have demonstrated that dopamine stimulated the phosphorylation of intracellular substrates, such as receptors, ion channels, and transcription factors, to regulate neuronal excitability and synaptic plasticity through dopamine receptors. Recent advances in proteomics techniques have clarified the mechanisms through which dopamine controls rewarding and aversive behaviors through signal pathways in the NAc.
1. Introduction
Dopamine was discovered as a neurotransmitter in the brain by Dr. Arvid Carlsson in 1957
[1]. It plays important roles in a number of brain functions, including motor function, motivation, learning, and rewards
[2][3][4][5]. Dysfunctions in the dopaminergic system underlie various neuropsychological diseases, including Parkinson’s disease, schizophrenia, drug addiction, attention deficit hyperactivity disorder (ADHD), post-traumatic stress disorder, major depression, and restless legs syndrome
[6][7][8][9][10][11][12][13].
There are several dopaminergic pathways in the brain. Two major pathways are the nigrostriatal pathway and mesolimbic pathway, which project dopamine neurons from the substantia nigra to the dorsal striatum, and from the ventral tegmental area (VTA) to the ventral striatum, including the nucleus accumbens (NAc). Nigrostriatal dopamine neurons play an important role in motor functions, and their dysfunction causes Parkinson’s disease
[6]. Accumulated evidence has revealed that dopamine transmission from the VTA to NAc is critical for controlling emotional behaviors, including rewarding and aversive behaviors
[14][15]. Emotional behaviors contribute to the better survival of organisms. For example, learning and memory related to rewards and aversion allow animals to efficiently obtain food or to escape from danger, in line with the theory of “survival of the fittest”.
Classical conditioning is a kind of simple reward-associated learning
[16]. Extracellular dopamine levels increase in the NAc when a reward or reward cue is delivered
[17]. Cue-evoked dopamine is essential for reward-associative learning, as demonstrated by both the pharmacological inhibition of dopamine receptors
[17] and the optogenetic inhibition of VTA dopamine neurons
[18]. Cue-evoked dopamine also promotes conditioned responses during learning
[19].
In contrast with the phasic firing response of VTA dopamine neurons to rewarding stimuli, their reactions to aversive stimuli are not homologous. The firing of some VTA dopamine neurons is activated, whereas the others are transiently suppressed. The inactivation of VTA dopamine neurons elicited by the optogenetic inhibition of dopamine neurons
[20] or the activation of VTA GABAergic interneurons
[21] has previously been shown to induce aversive behavior. Aversive behavior induced by the inactivation of VTA dopamine neurons is mediated by dopamine D2 receptors in the NAc
[20]. Therefore, reductions in dopaminergic activity are associated with aversive behavior.
Dopamine exerts its function through dopamine receptors, which are a class of G-protein-coupled receptors. There are five types of dopamine receptors: D1–5. D1 and D5 are coupled to Gs, the stimulation of which increases the intracellular concentration of the second messenger cyclic adenosine monophosphate (cAMP) through adenylate cyclase (AC), thereby activating cAMP-dependent protein kinase (PKA). On the other hand, D2, D3, and D4 are coupled to Gi, the activation of which results in the inhibition of the cAMP/PKA signaling pathway
[22]. Therefore, D1 and D5 receptors are called the D1-like receptor family. D2, D3, and D4 receptors are called the D2-like receptor family. In addition, D2 receptors are known to transduce the signal via noncanonical G protein–independent interactions with β-arrestins
[23]. Arrestin recruitment to D2R has been shown to mediate an antipsychotic effect in several studies
[24], and to mediate locomotion, but not incentive motivation, in another study
[25]. In this entry, researchers focused on the canonical phosphorylation signaling pathway. Recent advances in proteomics techniques have enabled researchers to clarify the novel mechanisms through which dopamine controls both rewarding and aversive behaviors through protein phosphorylation in the NAc.
2. Functional Model for Dopamine D1 Receptors Expressing Medium Spiny Neurons (D1R-MSN) and Dopamine D2 Receptors Expressing Medium Spiny Neurons (D2R-MSN) in Rewarding and Aversive Behaviors
In the NAc, approximately 95% of neurons are medium spiny neurons (MSN) receiving dopaminergic regulation from the VTA
[26][27]. There are two types of MSN—dopamine D1 receptors expressing D1R-MSN and dopamine D2 receptors expressing D2R-MSN. Dr. Hikida and co-workers expressed the tetanus toxin light chain, which is a bacterial toxin that cleaves the synaptic-vesicle-associated VAMP2 protein and abolishes neurotransmitter release from synaptic vesicles, in D1R-MSN and D2R-MSN
[28]. The cell-type-specific inhibition of D1R-MSN with the tetanus toxin resulted in diminished rewarding behavior, while that of D2R-MSN led to diminished aversive behavior. These findings indicate that the activation of D1R-MSN regulates rewarding behavior, while that of D2R-MSN encodes aversive behavior. Striatal tonic dopamine release sustains basal extracellular dopamine concentrations in the NAc. Rewarding stimuli may induce phasic dopamine release to increase dopamine concentrations, and aversive stimuli inhibit tonic dopamine release to decrease dopamine concentrations
[15][29]. Although dopamine was previously regarded as a volume transmitter that signals slowly and inaccurately, a recent study revealed that striatal dopamine secretion was mediated by sparse active-zone-like release sites supporting the dynamic function of dopamine
[30][31]. According to the nature of D1R and D2R, a model has been proposed to explain how the active state shifts between D1R-MSN and D2R-MSN, regulating rewarding and aversive behaviors, respectively (
Figure 1)
Figure 1. Dopamine concentrations function as a switch in the active state shift between D1R-MSN and D2R-MSN to regulate rewarding and aversive behaviors, respectively. (a) At the concentration of dopamine under basal conditions, D1R-MSN and D2R-MSN are both inactive. (b) When the concentration of dopamine is high, D1R-MSN is activated, consequently leading to rewarding behavior. Under pathophysiological conditions, the dopamine hyperfunctional state may be associated with schizophrenia and drug addiction. (c) When the concentration of dopamine is low, D2R-MSN is activated to induce aversive behavior. Under pathophysiological conditions, the dopamine hypofunctional state may be associated with Parkinson’s disease, attention deficit hyperactivity disorder, and restless legs syndrome. D1R, dopamine D1 receptor; D2R, dopamine D2 receptor; A1R, adenosine A1 receptor; A2AR, adenosine A2A receptor; MSN, medium spiny neuron; Gs, stimulatory G protein; Gi, inhibitory G protein; AC, adenylate cyclase; cAMP, cyclic adenosine monophosphate; PKA, protein kinase A.
D1R is coupled to Gs, the stimulation of which activates PKA to trigger D1R-MSN. On the other hand, D2R is coupled to Gi, the activation of which results in the inhibition of the cAMP/PKA signaling pathway and, in turn, the suppression of D2R-MSN. D2R has a markedly higher affinity for dopamine than D1R
[32], and the Kd values of D1R and D2R are around 200 nM and 10 nM, respectively
[33]. Therefore, basal dopamine concentrations, which have been reported to be around 10 nM
[34], are insufficient to activate D1R and induce the activation of D1R-MSN, but are adequate to activate D2R and suppress D2R-MSN activity (
Figure 1a). Rewarding stimuli markedly increase extracellular dopamine (e.g., cocaine intake has been shown to increase dopamine levels to more than 280 nM
[35]), which is followed by the activation of both D1R and D2R. The stimulation of D1R induces the activation of D1R-MSN, whereas that of D2R suppresses D2R-MSN activity (
Figure 1b). Aversive stimuli reduce extracellular dopamine to levels (e.g., electric footshock has decreased dopamine levels to less than 10 nM
[36]) at which neither D1R nor D2R is activated. Furthermore, D1R-MSN expresses the adenosine A1 receptor (A1R)
[37], which couples to the Gi protein, and D2R-MSN expresses the adenosine A2A receptor (A2AR)
[37], which couples to the Gs protein. Basal extracellular adenosine concentrations are sufficient to tonically activate A1R and A2AR
[38]. Therefore, D2R-MSN is activated due to the disinhibition in the D2R signal (
Figure 1c). With the cooperation of adenosine, dopamine concentrations function as a switch for controlling the active state shift between D1R-MSN and D2R-MSN, thereby regulating rewarding and aversive behaviors, respectively
[39]. A recent study showed dopamine terminals in the ventral NAc medial shell are excited, but other NAc regions are inhibited by aversive stimuli
[40]. Although changes in the dopaminergic activity seem to be different in the subregion of NAc after aversive stimuli, this model may be applicable for NAc regions, except for the ventral medial shell.
3. PKA and PKA Substrates
Rewarding and aversive behaviors in rodents are monitored by conditioned place preference (CPP) and passive avoidance tests, respectively. The inhibition of PKA in the NAc blocks amphetamine-induced place preference
[41] and its activation in D1R-MSN enhances cocaine-induced place preference
[42]. The inhibition of PKA in D2R-MSN blocks foot shock-produced avoidance, whereas its activation increases foot shock-produced avoidance
[43]. These findings strongly demonstrate that PKA plays an important role in D1R-MSN and D2R-MSN to regulate rewarding and aversive behaviors, respectively.
The PKA-dependent phosphorylation of receptors, ion channels, transcription factors, and other proteins accounts for the structural and functional plasticity regulated by dopamine
[44] (
Figure 2).
Figure 2. The PKA-dependent phosphorylation of receptors, ion channels, transcription factors, and other proteins accounts for structural and functional plasticity regulated by dopamine. D1R, dopamine D1 receptor; Gs, stimulatory G protein; AC, adenylate cyclase; cAMP, cyclic adenosine monophosphate; PKA, protein kinase A; DARPP-32, dopamine- and cAMP-regulated phosphoprotein of 32 kDa; PP1, protein phosphatase-1; NMDAR, NMDA receptor; AMPAR, AMPA receptor; STEP, striatal-enriched protein tyrosine phosphatase; Brd4, bromodomain-containing protein 4; DGLα, diacylglycerol lipase-α; rpS6, ribosomal protein S6. “?” indicates unknown PKA substrates.
4. Phosphoproteomic Analyses Revealed New Phosphorylation Signals Downstream of Dopamine Receptors in Emotional Behaviors
Major efforts have been made to identify the target substrates of PKA so as to understand the modes of action of dopamine, and a few of its substrates, including DARPP-32, GluA1, and NR1, have been reported. The PKA-mediated phosphorylation of DARPP-32 indirectly accumulates phosphoproteins through the inhibition of protein phosphatase PP1. However, it remains unclear about the proteins directly phosphorylated by PKA. To identify the PKA substrates that are responsible for the function of dopamine, a Kinase-Oriented Substrate Screening (KiOSS) method, a novel comprehensive phosphoproteomic analysis, has been developed. To provide information on the phosphorylation signals identified by KiOSS methods, as well as those previously reported in the literature, researchers developed a novel online database, KANPHOS (https://kanphos.neuroinf.jp).
According to previous studies and researchers' KANPHOS data, the stimulation of D1R by dopamine increases intracellular cAMP concentrations through AC, and this is followed by the activation of PKA. PKA phosphorylates Rasgrp2 and Rap1gap to induce the activation of Rap1, which promotes the MAPK pathway. MAPK phosphorylates Kcnq2 to increase membrane excitability. MAPK also phosphorylates Npas4 and MKL2 to facilitate the gene expression, accounting for synaptic plasticity regulated by dopamine. The stimulation of A2AR without the inhibition of D2R also increases intracellular cAMP concentrations through AC, and this is followed by the activation of PKA. The activation of Rap1 induces the MAPK pathway to regulate synaptic plasticity. The stimulation of M1R induces the activation of PKC, and this is followed by the phosphorylation of Kcnq2, which accounts for the increased membrane excitability. Therefore, phosphorylation signals downstream of D1R are involved in D1R-MSN-mediated rewarding behavior, and phosphorylation signals downstream of A2AR and M1R without the inhibition of D2R are involved in D2R-MSN-mediated aversive behavior (Figure 3).
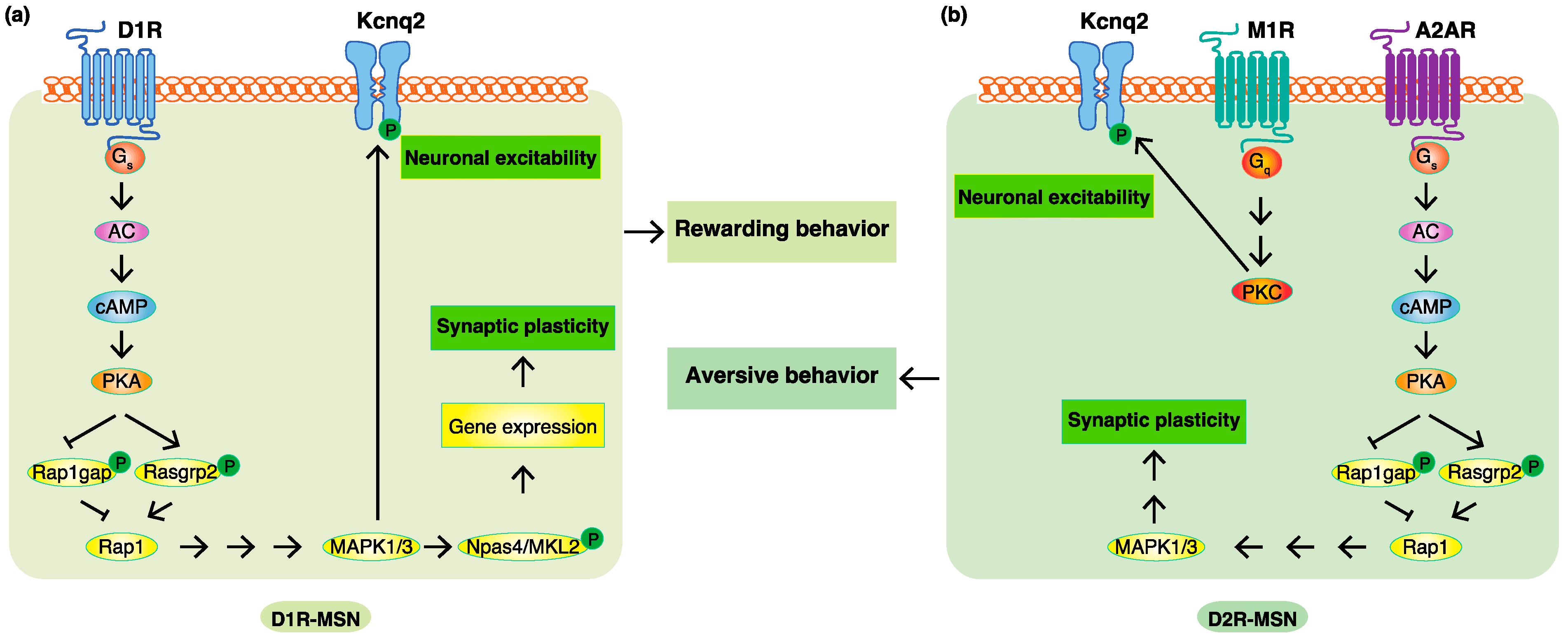
Figure 3. Phosphoproteomic analyses revealed new phosphorylation signals downstream of do-pamine receptors in emotional behaviors. (a) In D1R-MSN, the stimulation of D1R by dopamine increased intracellular cAMP concentrations through AC, and this was followed by the activation of PKA. PKA phosphorylates Rasgrp2 and Rap1gap to induce the activation of Rap1, which pro-motes the MAPK pathway. MAPK phosphorylates Kcnq2 to increase membrane excitability and phosphorylates Npas4 and MKL2 to facilitate the expression of the genes accounting for synaptic plasticity, consequently leading to rewarding behavior. (b) In D2R-MSN, a decreased dopamine concentration cancels the suppressive effects of D2R. The stimulation of A2AR without the inhibi-tion of D2R increases intracellular cAMP concentrations through AC, and this is followed by the activation of PKA. PKA phosphorylates Rasgrp2 and Rap1gap to induce the activation of Rap1, which promotes the MAPK pathway involved in synaptic plasticity. Acetylcholine/M1R signaling activates PKC to promote the phosphorylation of Kcnq2, which is involved in membrane excita-bility. These changes consequently lead to aversive behavior.