Nutritional interventions may highly contribute to the maintenance or restoration of human health. Grapes (Vitis vinifera) are one of the oldest known beneficial nutritional components of the human diet. Their high polyphenol content has been proven to enhance human health beyond doubt in statistics-based public health studies, especially in the prevention of cardiovascular disease and cancer.
1. Introduction
Vitis vinifera grapes are extremely rich in bioactive components
[1]. Grape marc is a mixture of grape seeds and skins, which remain as a by-product of the wine production process, making up 20–25% of the grape’s weight
[2]. Grape seeds contain fats, proteins, carbohydrates, and 5–8% polyphenols. The grape seed is rich in extractable phenolic antioxidants such as phenolic acids, flavonoids, proanthocyanidins, and resveratrol, and the grape skin is abundant in anthocyanins
[3]. Grape marc also contains a large amount of lipids, proteins, indigestible fibers, and minerals
[1][2].
Around 1.000 kg of grapes is used to produce 750 L of wine. By way of comparison of start- and end-product masses, this means that about 60% of the grape harvest mass will become agricultural waste
[4]. As an example, in 2017, Chinese grape production was 13,083,000 tons and South African grape production was 2,032,582 tons
[5]. Hence, there is a huge untapped potential in the use and extraction of active substances from grape seeds, skins, and pomace.
Polyphenols
Polyphenols are so-called secondary metabolites of plants, biologically active compounds in order to enhance plants adaptation to environmental conditions, for example balancing oxidative stress
[6]. Polyphenols are plants’ active substances consisting of more than one phenolic group. In food, more than 15 classes of polyphenols can be found
[7]. The polyphenols are largely flavonoids that can be further subdivided into 13 subclasses where more than 8000 components have been described. Flavonoids are the largest and most-studied group of phenols. Their seven main subclasses are flavones, flavonones, flavonols, isoflavones, anthocyanidins/anthocyanins, flavanols (or catechins and procyanidins), and chalcones
[7]. Another group of flavonoids not included in this list are proanthocyanidins, also known as procyanodins, condensed tannins, or oligomeric procyanidins
[7]. High molecular weight (from 500 D up to 20,000 D) polyphenols are plant tannins
[8]. Polyphenols can generally be subdivided into hydrolyzable tannins (tannic acid esters with glucose or other sugars)
[9], phenylpropanoids (lignins, flavonoids)
[10][11][12], and condensed tannins
[13]. Polyphenol compounds, especially procyanidins, contribute to the bitter and astringent taste of juices shaping the aroma of wines
[14]. The coloring agents from the grape skins are considered “generally recognized as safe” (GRAS) and are utilized as food colorants
[14].
In grapes, flavonoids are mainly found in the seeds, fruit skins, and stems. Between 60 and 70% of the total recoverable polyphenols in grapes are in the seed, which accounts for 5–8% of the weight of the seed
[15]. Hundreds of polyphenolic compounds are present in wine, which influence the taste, color, and flavor of the wine
[14]. The extractable phenolic antioxidants account for 10–11% of the dry weight of the grape marc. The polyphenolic composition of marc is varietal. Red grapes are richer in proanthocyanidins, while in white grapes they are scarcely present. The composition of polyphenols depends on the grape variety, the weather, the place of cultivation, and the maturity of the grapes
[16]. The largest and best-known constituents of polyphenols are flavonoids
[17] (
Figure 1). The vast majority of polyphenols in grape seeds are flavonoids
[18]. The classification of polyphenols and the characteristic functions of each molecular class are summarized in
Table 1.
Figure 1. General structure of polyphenols.
Table 1. Main groups of polyphenols.
The main polyphenolic constituents in grape seeds are catechins (catechin, epicatechin, procyanidin
[19]). Except for epicatechin, they are found in the outer, soft layer of the grape seed. The most physiologically important compounds of polyphenols isolated from grape seeds are summarized in
Table 2.
Table 2. Most important physiologically active compounds of the polyphenol fraction isolated from grape marc (grape seeds and grape skins).
Source |
Compound Name |
Classification |
Structural Formula |
Function |
Grape seed and skin |
Cyanidin |
Anthocyanidin |
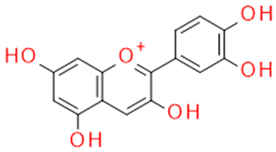 |
Oxygen radical sequestration |
Catechin/Epicatechin |
Catechins flavan-3-ol |
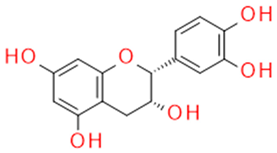 |
Anticancer Antisclerotic Antidiabetic Free radical sequestration |
Quercetin |
Flavonol |
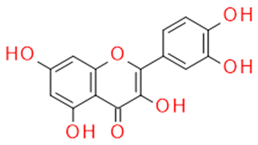 |
Anti-inflammatory Antiallergic Anticancer Antioxidant |
Whole grapes |
Resveratrol |
Fitoalexin Stilbene |
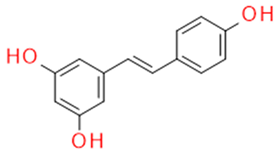 |
Antioxidant Antimicrobial Anticancer Anti-inflammatory Blood glucose lowering |
Rutin |
Quercetin-3-rutinozide, flavonoid |
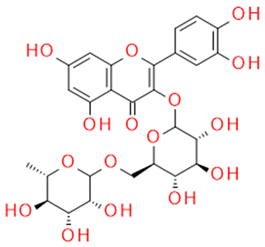 |
Anti-inflammatory Vasoprotective Blood clotting inhibitor Antidiabetic |
2. Basic Physical and Chemical Properties of Polyphenols
2.1. Physical Properties
The most important physical properties of the main monomeric components of polyphenols, such as catechol, epicatechin (EC), and epicatechin-(3-O)-gallate (EGC) monomers are summarized in Table 3. Properties relevant for the separation of the components:
Table 3. Physical properties of the main monomeric components of polyphenols.
Molecular weight: Based on the differences in molecular weight, it is possible to separate fractions by gel chromatography or membrane filtration.
Solubility: Several polyphenols are water-soluble, and many are lipid-soluble. In general, catechins are fat soluble and procyanidins are water-soluble. This allows their relatively easy separation by extraction.
2.2. Chemical and Biochemical Properties of Polyphenols
Hydrogen donor: Polyphenols have numerous hydroxyl groups, acting as hydrogen donor antioxidants, and scavenging singlet oxygen. Therefore, they can be classified as reducing agents. They form chelates with metals. They bind free radicals and stop radical chain reactions
[20].
Stability: The antioxidants that can be extracted from grape seeds are very sensitive to oxygen, light, acidic, or alkaline environments, and variably sensitive to heat
[21].
Polyphenol-protein interactions: The polyphenols in grape seed extract can form strong, specific bonds with protein binding units (e.g., proline-rich proteins). This binding is used in the extraction of polyphenols and the extraction of plant proteins (gluten removal). They can also inhibit or activate enzymes in grapes that protect the fruit from microbial attack. The interactions between polyphenols and proteins can be covalent, ionic, hydrogen-bonded, or hydrophobic. Many proteins can precipitate polyphenols
[22].
2.3. Analysis of Polyphenols
Several simple and inexpensive methods for the analytical determination of antioxidants have been developed (
Table 4). Antioxidant activity can be determined simply by the FRAP method (FeCl
3 and using triazine)
[23], and total antioxidant content can be determined by András Boór’s determination of 2,4,6-Tris(2-pyridyl)-s-triazine
[23], total polyphenol content can be determined by Folin Ciocalteu reagent
[23]. Free radical scavenging activity can be measured using 1,1-Diphenyl-2-picrylhydrazine
[23]. The anthocyanin content in hydrochloric acid ethanol can be determined spectrophotometrically (550 nm)
[24], and leucoanthocyanins can be determined spectrophotometrically after heating with a hydrochloric acid-butanol mixture containing ferrous sulfate (II) in a 40:60 ratio
[24]. Determination of catechol content in alcohol diluted solution reacted with sulfuric acid vanillin at 500 nm spectrophotometrically is simple
[25], and resveratrol content can be determined directly in HPC by the Kállay-Török method
[26] (
Table 4).
Table 4. Methods used for the determination of antioxidant content.
Title |
Method |
Materials Needed |
Literature |
Antioxidant activity determination |
FRAP method |
FeCl3, triazine |
[23] |
András Boór total antioxidant content |
|
2,4,6-Tris(2-pyridyl)-s-triazine |
[23][27] |
Determination of total polyphenol content |
|
Folin Ciocalteu Reagent, Gallic acid, Na2CO3, Methanol |
[23] |
Free radical scavenging activity (antiradical activity) |
|
1,1-Diphenyl-2-picrylhydrazine |
[28] |
Determination of anthocyanin content |
Dilution at 550 nm with 96% ethanol containing 2% HCL at 2% v/v, followed by spectrophotometry |
|
[24] |
Determination of leucoanthocyanins |
spectrophotometrically after heating with a 40:60 mixture of hydrochloric acid and butanol containing ferrous sulphate |
|
[24] |
Determination of catechin content |
reacted with sulphuric acid vanillin in an alcohol-diluted solution at 500 nm by spectrophotometry |
Vanillin |
[25] |
Resveratrol content determination |
directly to HPLC |
|
[26] |
3. In Vivo Investigations of Grape Seed Extract and Its Components
The number of in vivo investigations using a wider range of immunohistochemistry to examine various molecular mechanisms of action is growing exponentially (Table 5).
Table 5. In vivo experiments for investigations of healing effects of grape seed extract and its components in different diseases.
By using MTT assay, flow cytometry, and immunoblot analysis, lipophilic grape seed proanthocyanidin (LGSP) was assessed for its anti-prostate cancer activity against the PC3 cell line in vitro. A mouse xenograft model generated from PC3 was used to test LGSP’s anti-prostate cancer impact in vivo. In tumor tissues, immunostaining tests for Ki67 and cleaved caspase 3 were carried out. By triggering apoptosis, LGSP had a potent inhibitory effect on PC3 cell proliferation
[29].
Treatment with LGSP caused G1 phase cell cycle arrest in PC3 cells, which was further validated by increased expression of the tumor suppressor p21 and p27 and decreased expression of cyclin D1 and CDK 4. Additionally, it was demonstrated that LGSP-induced apoptosis is caspase-dependent by the activation of cleaved fragments of caspases 3, caspase 9, as well as PARP. LGSP boosted the release of cytochrome c in the cytoplasm upstream of the caspase cascade. In PC3 cells following LGSP administration, the Bcl-2/Bax ratio likewise fell
[29].
Considering tumor research, LGSP inhibited the proliferation of PC3-derived mouse xenografts and induced apoptosis
[29].
Nude mice with a human liver cancer cell (HepG2)-derived xenografts were treated with grape seed proanthocyanidins (GSPs). According to the findings, GSPs triggered autophagy, and inhibiting autophagy caused an increase in apoptosis in HepG2 cells. Since stimulating the phosphorylation of mitogen-activated protein kinase (MAPK) pathway-associated proteins, p-JNK, p-ERK, and p-p38 MAPK, and decreasing the expression of survivin, GSPs at 100 mg/kg and 200 mg/kg significantly inhibited the proliferation of HepG2 cells in nude mice without manifesting toxicity or autophagy
[30].
Grape seed procyanidin reverses the change in pulmonary hemodynamics in the cigarette smoke-induced pulmonary arterial hypertension model applied in rats. According to mean pulmonary arterial pressure, pulmonary vascular resistance, right ventricular hypertrophy index, wall thickness, and wall area data grape seed procyanidin decreases the inflammation by the PPAR-γ/COX-2 pathway
[31].
In monocrotaline-induced pulmonary arterial hypertension rats, mean pulmonary arterial pressure, pulmonary vessel resistance, right ventricular hypertrophy index, percentage of medial wall thickness, percentage of medial wall area, and lung weight of wet and dry tissue ratio all decreased. The expression of endothelial nitric oxide synthase in lung tissue and plasma NO levels were raised up; the Ca
2+ level in pulmonary arterial smooth muscle cells (PASMC) was lowered; the transcription of inflammatory factors including myeloperoxidase, interleukin (IL-1, IL-6), and tumor necrosis factor-alpha (TNF-alpha) was down-regulated in lung tissue; the nuclear factor-B pathway was also inhibited
[32].
GSP improves locomotor recovery, decreases neuronal apoptosis, increases neuronal preservation, and manages microglial polarization in rats with spinal cord injuries (T9 vertebral laminectomy). Microglial polarization may be regulated by the TLR4-mediated NF-B and PI3K/AKT signaling pathways. These in vivo investigations are based on Locomotor Recovery Assessment; Terminal Deoxynucleotidyl Transferase dUTP Nick-End Labeling (TUNEL) Assay; Annexin V-FITC/PI Assays; NO assay and immunofluorescence staining: NeuN, GFAP, CD86, CD206, p-NF-κB-p65, p-AKT
[33].
Neuroprotective effect of red grape (
Vitis vinifera) seed and skin extract (GSSE) was determined in a mice model of Parkinson’s disease induced by neurotoxin 6-hydroxydopamine (6-OHDA), which causes oxidative damage and mimics the degeneration of dopaminergic neurons observed in Parkinson’s disease (PD). It was found that GSSE was effective in protecting dopamine neurons from 6-OHDA toxicity by reducing apoptosis, the level of reactive oxygen species (ROS), and inflammation; reducing the cleaved caspase-3 activity that helps inhibit 6-OHDA-induced mDA neuron death in a cellular model of PD; decreases ROS production induced by 6- OHDA in ESC-derived DA neurons; decreases phospho-NF-kB p65 activation induced by 6-OHDA in dopaminergic neurons; rescues motor deficits induced by 6- OHDA; prevents the loss of midbrain dopaminergic neurons (mDA) in a 6-OHDA mouse model of PD; prevents the loss of SOD1 level induced by 6-OHDA lesion. The biomarkers were for immunostaining: MAP2, AB5622, r tyrosine hydroxylase, caspase-3, and phosphorylated NF-kB p65
[34].