5-Aminolevulinic acid (5-ALA) is a natural amino acid and a precursor of heme and chlorophyll. Exogenously administered 5-ALA is metabolized into protoporphyrin IX (PpIX). PpIX accumulates in cancer cells because of the low activity of ferrochelatase, an enzyme that metabolizes PpIX to heme. High expression of 5-ALA influx transporters, such as peptide transporters 1/2, in cancer cells also enhances PpIX production. Because PpIX radiates red fluorescence when excited with blue/violet light, 5-ALA has been used for the visualization of various tumors.
1. Metabolism of 5-ALA in Viable Cancer Tissues
5-ALA is a natural amino acid and precursor of hemoglobin and chlorophyll
[1]. The metabolic pathway of 5-ALA and the mechanism of PpIX accumulation in living cancer cells are shown in
Figure 1. Exogenously administered 5-ALA is introduced into the cell via the action of transporters, such as peptide transporters (PEPTs) 1 and 2. Endogenous 5-ALA is produced from glycine and succinyl-CoA in the mitochondria via the enzymatic reaction of 5-ALA synthase (
ALAS) [2]. Two 5-ALA molecules are condensed by ALA dehydratase (ALAD) to generate porphobilinogen (PBG) in the cytoplasm
[2]. Four PBG molecules condense to produce hydroxymethylbilane (HMB) via the porphobilinogen deaminase (PBGD) reaction
[2]. In living cancer cells, HMB is converted to uroporphyrinogen III (UPG III) by uroporphyrinogen III synthase (UROS)
[2]. UPG III is converted to coproporphyrinogen III (CPG III) through decarboxylation of all acetate groups
[2]. CPG III enters mitochondria via ATP-binding cassette transporter B6 (
ABCB6). In the mitochondria, it is converted to protoporphyrinogen (Ppgen) and then to fluorescent PpIX
[2]. PpIX is converted to non-fluorescent heme via the action of ferrochelatase (FECH), which inserts ferrous iron
[2]. Moreover, heme negatively regulates
ALAS activity in some ways, such as by repressing
ALAS gene expression and degrading
ALAS mRNA
[3][4].
Figure 1. Metabolic pathways of 5-aminolevulinic acid (5-ALA) and mechanism of protoporphyrin IX (PpIX) accumulation in a viable cancer cell. PEPT 1/2, peptide transporter 1/2; ALAS, aminolevulinic acid synthase; ALAD, ALA dehydratase; PBG, porphobilinogen; PBGD, porphobilinogen deaminase; HMB, hydroxymethylbilane; UROS, uroporphyrinogen III synthase; UPG III, uroporphyrinogen III; UROD, uroporphyrinogen decarboxylase; CPG III, coproporphyrinogen III; ABCB6, ATP-binding cassette transporter B6; CPOX, coproporphyrinogen III oxidase; Ppgen, protoporphyrinogen; PPOX, protoporphyrinogen oxidase; FECH, ferrochelatase; ABCG2, ABC superfamily G member 2.
When 5-ALA is administered exogenously to humans, it is rapidly metabolized to heme in normal cells, but in cancer cells, owing to changes in the activity of enzymes in the heme synthesis pathway and/or transporters of 5-ALA and its metabolites, the heme precursor PpIX is selectively accumulated
[5][6][7][8]. As anaerobic metabolism in cancer cells is more active than in normal cells, termed the Warburg effect, the tricarboxylic acid cycle is downregulated, resulting in a lack of electrons required to reduce ferric ions to ferrous ions
[5]. This leads to the decreased activity of FECH, which transforms PpIX into heme. Therefore, PpIX is selectively accumulated in cancer cells
[5][9]. Furthermore, it has been reported that the 5-ALA influx transporter PEPT1/2 is highly expressed in tumor cells, resulting in enhanced PpIX accumulation
[10][11][12]. Recently, overexpression of PEPT2 was reported to be related to stronger PpIX fluorescence intensity (FI) in grade 2/3 gliomas; modulation of the function of PEPT2 might thus improve fluorescence-guided resection in grade 2/3 gliomas
[12].
The activity of ABC superfamily G member 2 (ABCG2), which is used to discharge PpIX, also affects the PpIX FI (
Figure 1)
[11].
Figure 2 shows the effect of Ko143, a specific inhibitor of ABCG2, on PpIX FI in human breast cancer cells and xenografts
[13]. The combined exposure of cultured MCF-7 and MDA-MB 231 cancer cells to 5-ALA and Ko143 boosts PpIX-FI compared to that in cultured non-cancer MCF10A cells, suggesting an improvement in the accuracy of 5-ALA PDD (
Figure 2A)
[13]. Increased FI attributed to the combined administration of 5-ALA and Ko143 was also observed in cancer cells acquired by fine needle aspiration in a murine tumor model (
Figure 2B)
[13]. In addition, clinically used ABCG2-interacting kinase inhibitors, such as lapatinib, gefitinib, sunitinib, and PD169316, have also been reported to increase 5-ALA induced PpIX-FI in triple-negative breast cancer cell lines
[14].
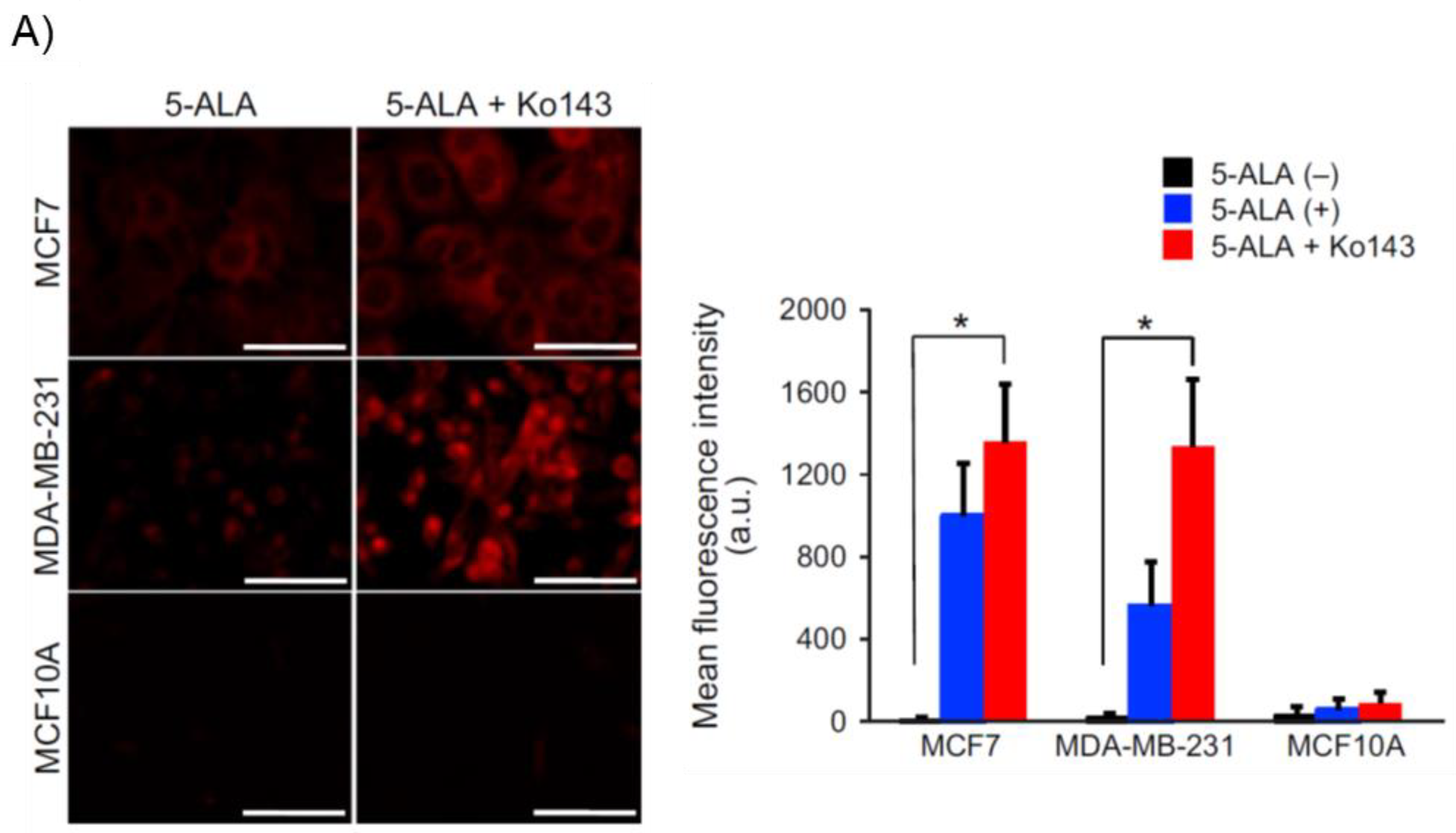
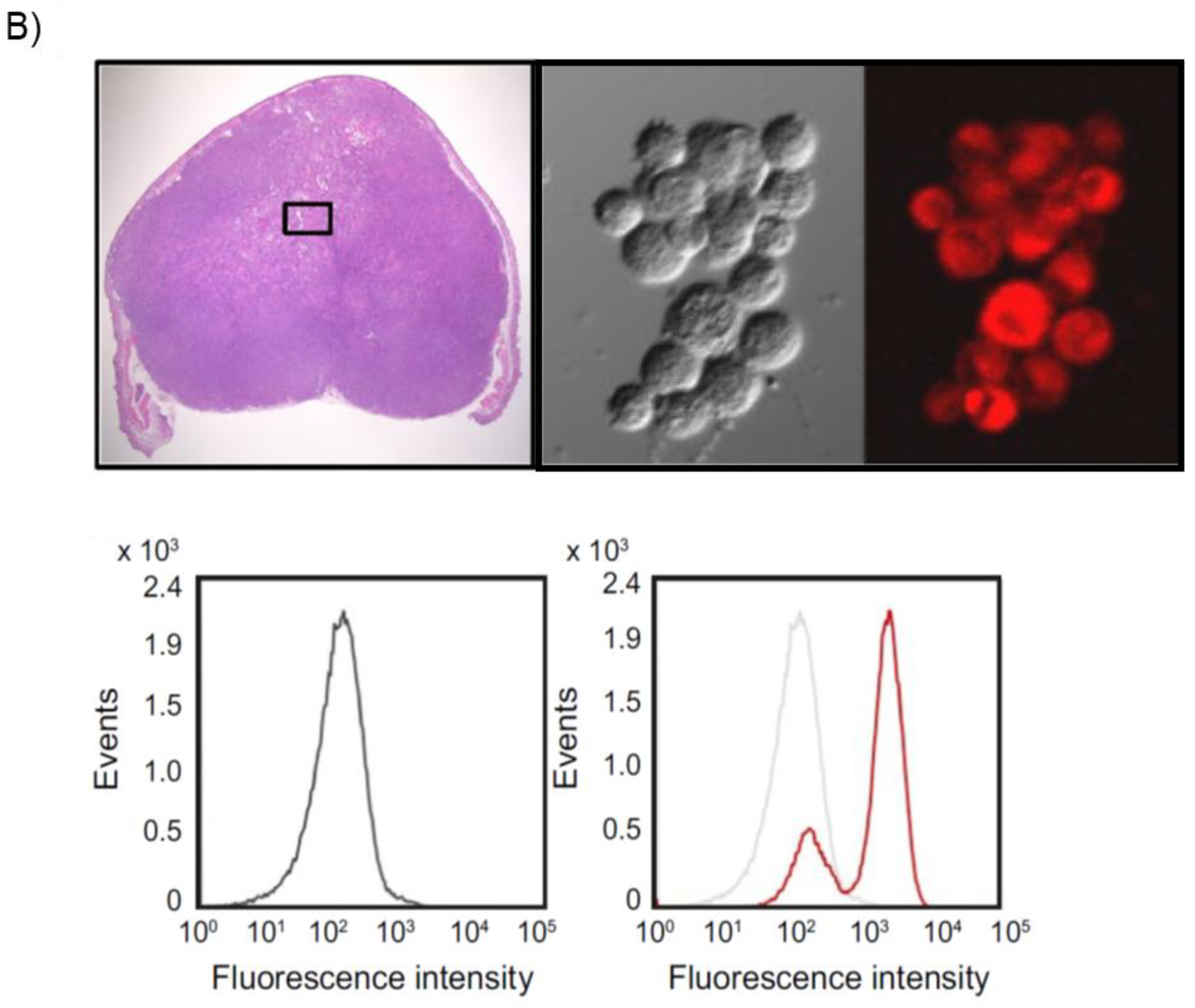
Figure 2. Enhancement of protoporphyrin IX (PpIX)-fluorescence intensity (FI) with an ABC superfamily G member 2 (ABCG2) transporter inhibitor Ko143 in human breast cancer cells and xenografts. “Reprinted/adapted with permission from Ref.
[13]. 2019, John Wiley & Sons Ltd”. (
A) Evaluation of PpIX fluorescence in cultured breast cancer cells (MCF7 and MDA-MB-231) and non-cancer cells (MCF10A) after exposure to 5-aminolevulinic acid (5-ALA; 5 mmol/L for 2 h) with or without Ko143 (1 μmol/L). Confocal fluorescence images of PpIX (left panels). Bars: 50 μm. Comparison of mean FI of PpIX (right panel). All error bars represent the SD (
n = 3). *
p < 0.05. (
B) Fluorescent analysis of fine needle aspiration (FNA) samples obtained from human breast cancer xenografts of MDA-MB-231 cells in nude mice. A hematoxylin and eosin image of a subcutaneous xenograft is shown (upper left panel). Differential interference contrast (upper middle panel) and PpIX fluorescence (upper right panel; excitation: 440 nm, emission: 615–645 nm) images of MDA-MB-231 cells collected through FNA after incubation with 5-ALA (5 mmol/L for 2 h) and Ko143 (1 μmol/L). Flow cytometry analyses of the cells with (lower right panel) and without (lower left panel) incubation with 5-ALA and Ko143 are shown. The black and red lines denote the control nontreatment group and the group treated with 5-ALA and Ko143, respectively. The horizontal axis shows PpIX intensity.
ABCB6, another transporter found in the outer mitochondrial membrane, was also reported to affect PpIX FI in malignant cells. Specifically,
ABCB6 is required for the transport of CPG III from the cytoplasm to the mitochondria (
Figure 1)
[8][15]. Zhao et al. investigated the expression of
ABCB6 in surgical specimens of human gliomas
[16]. The results showed that the levels were significantly elevated in human gliomas relative to those in normal brain tissues and that they corresponded to the World Health Organization (WHO) histologic grade
[16].
ABCB6 mRNA expression in distinctly fluorescent tumor tissues was higher than that in faintly fluorescent tumors, suggesting that
ABCB6 might be responsible for PpIX accumulation in glioma cells
[16].
Exocytosis, mediated by dynamin 2, a cell membrane-associated molecule, has been reported to influence PpIX accumulation (
Figure 1). Kitajima et al. showed a causal relationship between dynamin 2 expression and PpIX excretion in neoplastic cells using a panel of 39 human cancer cell lines from nine different tissues
[17]. Moreover, dynamin inhibitors significantly suppress PpIX excretion and increase its intracellular levels
[17].
2. Metabolism of 5-ALA in Tumor Necrotic Tissues
The metabolic pathway of 5-ALA in tumor necrosis in metastatic LN disease in patients with advanced esophageal cancer has recently been reported
[2]. Tumor necrosis is a feature that often occurs in advanced solid tumors and has been proposed to be a sign of poor prognosis for various types of tumors
[18]. In tumor necrosis, 5-ALA administration predominantly results in the production of uroporphyrinogen I (UPGI), but not UPGIII, via a non-enzymatic pathway, probably because of the lack of UROS enzyme activity (
Figure 3A)
[2].
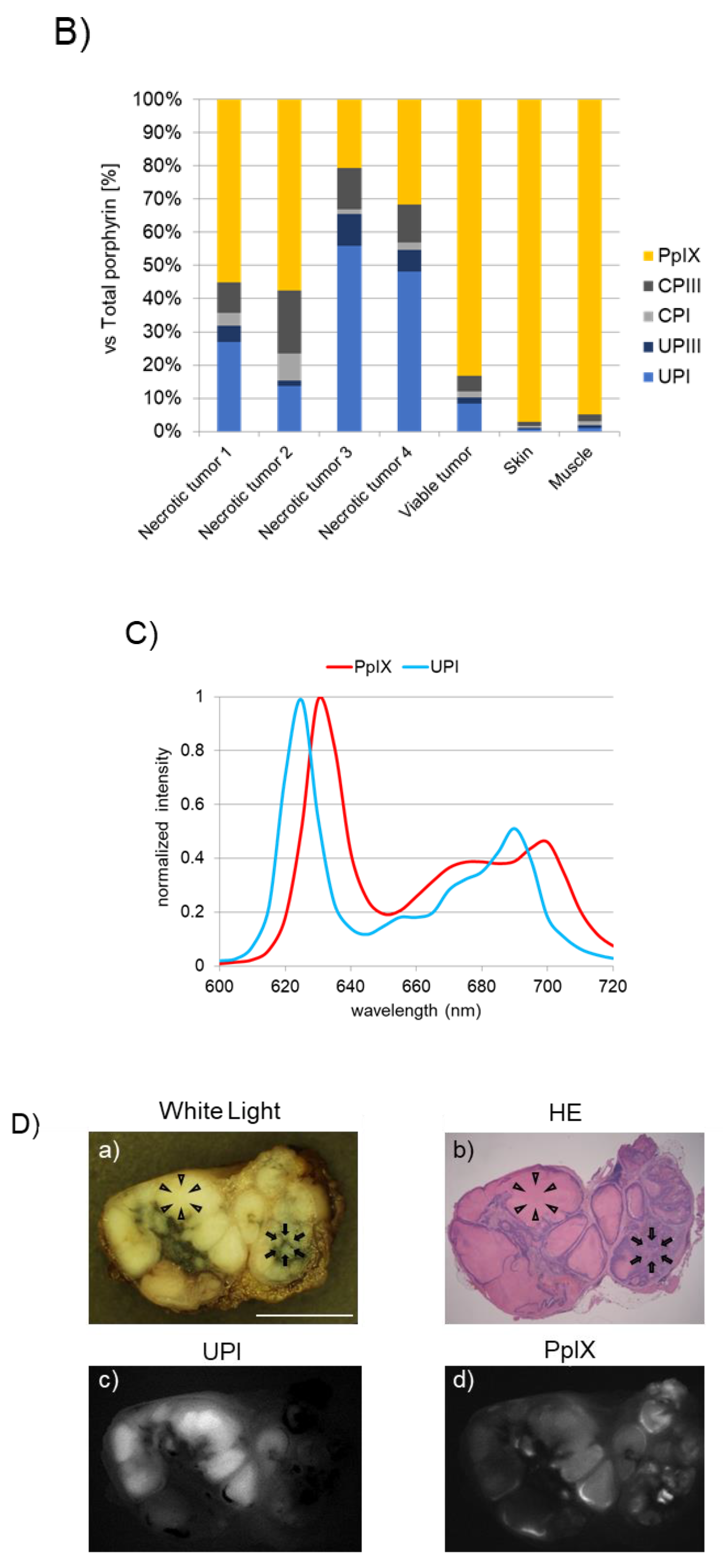
Figure 3. Accumulation of uroporphyrin I (UPI) in necrotic tumor tissues after administration of 5-aminolevulinic acid (5-ALA). “Reprinted/adapted with permission from Ref.
[2]. 2021, MDPI”. (
A) Metabolic pathways of 5-ALA in viable and necrotic tumor tissues. Black and gray arrows represent mainstream and non-mainstream routes, respectively. UPI, UPIII, CPI, and CPIII are produced from corresponding porphyrinogens via autooxidation and/or photocatalysis. PBG, porphobilinogen; PBGD, porphobilinogen deaminase; HMB, hydroxymethylbilane; UROS, uroporphyrinogen III synthase; UPGIII, uroporphyrinogen III; CPGIII, coproporphyrinogen III; PpIX, protoporphyrin IX; UPIII, uroporphyrin III; CPIII, coproporphyrin III; PPp, photo-protoporphyrin; UPGI, uroporphyrinogen I; CPGI, coproporphyrinogen I; CPI, coproporphyrin I. (
B) High-performance liquid chromatographic measurement of a subcutaneous B88 squamous cancer murine model. 5-ALA hydrochloride (250 mg/kg body weight) was administered intraperitoneally six hours before the experiment. The abundance ratio of each porphyrin to the total dose of porphyrin in necrotic and viable tumors and normal skin and muscle tissues excised from the murine model is depicted. Porphyrins including PpIX, CPIII, CPI, UPIII, and UPI were measured. (
C) Fluorescence spectra of UPI and PpIX pure chemicals. (
D) Imaging analyses of an excised metastatic lymph node (LN) consisting of necrotic and viable tumor cells in a patient with esophageal cancer. 5-ALA (15–20 mg/kg body weight) was orally given prior to surgery. White-light image (
a), hematoxylin–eosin (HE)-stained image (
b), and spectral unmixed fluorescence images of UPI (
c) and PpIX (
d) are shown. The LN was cut in half. Necrotic (arrowheads) and viable tumor tissues (arrows) were observed. Note that tumor necrosis and viable tumor tissue could be visualized with UPI and PpIX images, respectively (
c,
d). Scale bar, 5 mm.
Figure 3B shows the analytical results of high-performance liquid chromatography in a subcutaneous squamous cell cancer murine model, where the abundance ratio of each porphyrin to the total dose of porphyrin was described
[2]. UPI was found in necrotic tumors, whereas PpIX was detected principally in viable tumors, skin, and muscle tissues. UPI was readily produced from UPGI via autooxidation and/or photocatalytic reactions (
Figure 3A)
[2]. The fluorescence spectra of pure UPI and PpIX showed main peaks at approximately 620 nm and 635 nm, respectively (
Figure 3C)
[2].
Figure 3D shows the analytical results of metastatic squamous cancer with necrosis in a resected human LN. The locations indicated by arrows and arrowheads show viable and necrotic tumor lesions, respectively (a and b in
Figure 3D)
[2]. Unmixed fluorescence images of UPI and PpIX enabled the distinction of necrotic lesions from viable lesions (c and d in
Figure 3D)
[2]. Fluorescence peaks of tumor necrosis and living tumors were observed at 620 nm and 635 nm, respectively (data not shown)
[2]. In summary, in viable tumor tissues, voluminous PpIX is produced in viable tumor tissues because the UPGIII/CPGIII axis of the heme synthesis pathway is active (upper panel of
Figure 3A)
[2][19][20]. In contrast, in tumor necrosis, the spontaneous non-enzymatic route from HMB to UPGI precludes the one from HMB to UPGIII, leading to increased production of UPGI, a precursor of fluorescent UPI (lower graph of
Figure 3A)
[2][21][22]. In the field of brain surgery, an association between false-positive 5-ALA-induced red fluorescence and radiation necrosis has been reported
[23][24]. Although future studies are needed, UPI-derived red fluorescence may be a candidate for the origin of red fluorescence in radiation-associated necrosis.