The war on cancer was announced in 1971 by then President of the USA, Richard Nixon, and continues today
[1]. Although huge amounts of money have been spent and much has been done for the purpose, it is still not enough to empty hospital corridors of people waiting for rescue. Although a lot of research has been conducted, a lot of experimental data has been collected and a lot of articles have been published, it is still not enough to fully understand the mechanism of cancer initiation and progression. There are still phenomena during cancer development that are incomprehensible and therefore considered paradoxical
[2]. Today, i.e., after 50 years, the war on cancer goes on, seemingly endlessly
[3][4]. Today, a lot of cancer theories have been established (for example, more than 20 theories are presented in
[5]), and new theories are introduced all the time, for example, the detached pericyte hypothesis
[5]. Pursuant to another interesting idea, the main driver of cancer development is repeated loss of synchronization between the circadian clock (CC) and the cell cycle
[6]. This loss of synchronization leads to arrest of the mitotic cell cycle, reprogramming, polyploidization and activation of the ploidy cycle
[6]. Then, through depolyploidization, Hayflick’s limit is renewed, cells regain synchronization between CC, and the cell cycle and mitotic cell cycle are activated
[6]. Other studies also indicate the importance of polyploidy during cancer development
[7][8]. To answer to the question, “what is the reason for cancer, and what is the mechanism of its development”, it is necessary to combine existing theories into one unified theory on the basis of which it will be possible to interpret all phenomena (including that the occurrence of which is considered paradoxical) observed during cancer transformation and development. Most of these theories have been developed to explain phenomena that cannot be explained using the somatic mutation theory (SMT). SMT has been the dominant theory in a study of carcinogenesis for at least sixty years and says that cancer arises from a succession of driver mutations and clonal expansions
[9][10]. These phenomena that cannot be explained using SMT (thus proving the weaknesses of SMT) are, among others, (a) causation of cancers by a chemical not known to damage genes, (b) lack of an inducing mutation (for example, in transgenic mouse tumors), (c) infectious causation of cancers and (d) regression of cancer to a benign tumor
[11].
The reversal of cancer cells towards an atavistic form of life was formulated by some researchers as the atavistic theory of cancer
[12][13][14][15][16][17][18][19][20][21][22][23]. It is well known that atavisms can occur experimentally without a mutational basis
[24]. This feature of atavisms is especially important in light of the presented weaknesses of SMT and developing new models. Developed atavistic models take into account that cancer onset is a kind of reversion to the quasi-unicellular ancestral phenotype
[24]. Evolution of life on Earth over a period of 4 billion years can be divided into two main subperiods. The first and longest subperiod of evolution was dominated by establishing mechanisms responsible for life of unicellular organisms. These mechanisms include mechanisms related to competition, survival and proliferation. Prokaryotic cells probably transitioned into unicellular eukaryotic cells between 2.0 and 1.4 billion years ago
[25]. This transition was the basis for the next transition, i.e., the transition to multicellularity. The transition to multicellularity followed the first subperiod and occurred between 1.5 and 0.5 billion years before the present era. During this second, shorter subperiod of evolution, the newer mechanisms (including the pathways) to support multicellular life (inter alia, cell collaboration and differentiation) were established. In accordance with the atavistic cancer model, these newer mechanisms, because they were less well established during the shorter evolution subperiod, are more susceptible to damage than the conservative mechanisms responsible for unicellular life
[26]. Damage to the mechanisms responsible for multicellular life can cause cancer transformation. Cancer transformation, according to atavistic cancer models, involves the switch (i.e., the transition) to atavistic unicellular life. This switch is characterized by the occurrence of the Warburg effect and activation of the atavistic mechanisms of competition, survival and proliferation typical of primitive unicellular organisms. A recently proposed exemplary atavistic model assumes that cancer onset and development can be described as a series of reversionary transitions. In accordance with this model (called the serial atavism model), cancer can be considered as not a single atavism with a multicellular-to-unicellular switch but as a sequence of atavistic reversions
[24]. Activation of atavistic mechanisms implies the activation of processes that belong to the most conserved and protected processes supported by a myriad of built-in redundant pathways
[26]. This may explain the metabolic flexibility in cancer cells that are able to upregulate their compensatory pathways following inhibition of the dominant metabolic pathway
[27]. This metabolic flexibility leads to the development of resistance to metabolic inhibitors and is therefore a real challenge in the treatment of cancer
[27]. Many attempts have been made to date to eliminate cancer cells, including chemotherapy, hormone therapy, hyperthermia, immunotherapy, photodynamic therapy, radiation therapy, stem cell transplant, surgery and targeted therapy. Striving to understand the universal mechanisms of cancer transformation and development is crucial, as these mechanisms should reveal new ways to eliminate cancer cells more effectively.
2. Molecular Fundamentals of Unified Cell Bioenergetics and Bioenergetic Disturbances
The phenomenon of cancer can be observed, studied and interpreted both from “the outside” and “the inside”. It is difficult to say which approach is better, although it should be noted that the conclusions drawn from both perspectives must be consistent.
Looking from “the outside”, a very large, genetically heterogeneous set of cells is usually visible
[28]. It is known that population genetics meets cancer genomics
[29]. The understanding of some population genetic aspects of cancer development can be supported by advances in molecular genetics
[29]. Moreover, interpretations of the mechanisms responsible for cancer development and the genetic heterogeneity of cancer cells can be made, for example, by generating phylogenetic trees and analyzing the length of phylogenetic tree branches. Observations from these analyses show that mutations accrue faster in some cancer regions than in others, as evidenced by the large variations in branch length
[28]. Interesting conclusions from this observation include that a malignant tumor (i.e., colorectal cancer as an exemplary examined malignant tumor) occupies a sharper fitness peak compared to that of a benign tumor (i.e., adenoma as an examined exemplary benign tumor) which evolves across an undulating fitness landscape
[28].
From “the inside”, i.e., from the perspective of the low-molecular level, a lot of different phenomena result from a huge number of metabolic pathways. For this reason, at first, it is important to select fundamental phenomena that can be considered as main bioenergetic drivers of life. The next attempt should be to combine these fundamental phenomena into one coherent whole in order to understand the universal bioenergetic mechanisms that drive life. Then, from the perspective of this unification, one can try to interpret more and more complex phenomena step by step.
The fundamental cellular phenomena that can occur during eukaryotic cell metabolism include the Pasteur, Crabtree, Kluyver and glucose effects. However, by examining the occurrence of each of these effects individually, it is extremely difficult to understand the more complex mechanisms that drive the life of multicellular organisms. A solution to take a step forward in understanding might be to unify all these fundamental bioenergetic effects into one generalized effect. According to unified cell bioenergetics (UCB), the unification of these fundamental effects is possible by examining the intramitochondrial level of energy-storing NADH molecules (i.e., mtNADH molecules)
[30][31]. In the eukaryotic cells, the Krebs cycle rotation is coupled with several reactions (i.e., reductions), from NAD to NADH
[32]. In accordance with UCB, mitochondria are charged with NADH molecules during the Krebs cycle, and NADH is discharged from mitochondria in the electron transport chain
[30][31][33][34][35]. In this light, overloading the cell with an excessive amount of food or malfunctioning oxidative phosphorylation (OXPHOS) can lead to serious bioenergetic problems related to the accumulation of huge amounts of high-energy molecules (especially NADH) in mitochondria
[30][31]. The accumulation of high-energy molecules, as a result overenergization of mitochondria, may occur due to the impermeability of the mitochondrial inner membrane to NADH
[36][37]. NADH accumulation occurs (as a consequence of the impermeability of the mitochondrial inner membrane to NADH) when the rate of charging mitochondria with NADH is higher than the rate of discharging mitochondria from NADH
[31]. Under these conditions, a gradual increase in NADH occurs. An increase in NADH causes an exponential increase in ROS
[38][39][40]. A high ROS level poses an additional problem for the cell, as it can result in severe oxidative damage to DNA, biolipid membranes and proteins
[3][41][42]. In order to protect mitochondria from overenergization, the cell stimulates aerobic fermentation. The occurrence of fermentation under good aerobic conditions is called the Crabtree effect
[31][43]. In light of unified cell bioenergetics, the Crabtree effect occurs when the intramitochondrial NADH amount exceeds the mtNADHnormal level (i.e., the normal level of intramitochondrial NADH)
[31]. The Crabtree effect precedes the occurrence of the Warburg effect
[30][44][45]. In accordance with unified cell bioenergetics, bioenergetic cell problems, especially overenergization of mitochondria by NADH molecules, can lead to cancer transformation and subsequent cancer development
[30][33]. Moreover, cancer cells remain overenergized after transformation and during cancer development (see
Section 3.5)
[30][33]. For this reason, cancer cells also have higher levels of ROS than their non-cancerous cells of origin. This conclusion was also confirmed by other researchers
[42][46][47][48][49][50]. Moreover, it is known that excessive levels of ROS cause cell death; therefore, cancer cells adapt multiple metabolic strategies (by adaptation of genome expression) to avoid excessive increases in ROS beyond the level that causes excessive oxidative stress and leads to cell death
[46].
3. Layered Model of Evolution of Cellular Functionalities
During the process of building complex and functional multicellular organisms, strong constraints preventing inappropriate atavisms (including uncontrolled cell proliferation) also had to evolve
[51]. This process did not necessarily remove the ancestral programs but rather prevented their expression
[52]. Multicellular programs (that evolved during this process) are believed to be oncosuppressive, and when working properly, they control unicellular programs
[53]. For this reason, malfunctions in the operation of multicellular programs due to cancer-promoting factors may cause the activation of unicellular-like programs that promote cancer
[53]. According to the systemic–evolutionary theory of cancer (i.e., SETOC, one of the newest theories about the origin of cancer), cancer is generated by the re-emergence of older cellular evolutionary subsystems (such as archaea-like and/or prokaryotic-like subsystems) that are characterized by uncoordinated behaviors
[53]. The possibility of activation of older, ancestral (primordial) evolutionary functionalities (i.e., according to the SETOC: unicellular-like programs) by cells of multicellular organism suggests that during evolution, older functionalities are stored, undergoing activity control by newer evolutionary functionalities (i.e., according to the SETOC: multicellular programs). These atavistic (or primordial) behaviors are preserved by cells as a phylogenetic memory
[53]. A phylogenetic memory is represented in a cell by a genome
[54]. Repair mechanisms enable this memory to have a very low error rate (i.e., 1 in 10
10 base pairs), which is incredibly accurate
[54]. For this reason, the stability of the phylogenetic memory that accumulates knowledge for millions of generations is very high
[54]. This indicates that during evolution, a hierarchical organization of cellular functionalities is established with very precisely stored and preserved ancestral functionalities.
In accordance with a model, cellular functionalities are located in layers, where new, more developed cellular functionalities are added to the more external layers. In this way, during the evolution, a layered structure of functionalities is created in which the outer layers contain new functionalities that can use, control (including deactivation) and extend the old functionalities that are stored (as a phylogenetic memory) in more internal layers. For example, the functionality of facultative switching between oxidative phosphorylation and aerobic glycolysis extends the less developed functionality of aerobic glycolysis
[55]. Huge disturbances (or destruction) of functionalities can lead to a loss of control over functionalities of more internal (i.e., atavistic) layers, i.e., such disturbances can lead to an uncontrolled reactivation of an evolutionarily older cell fate.
3.1. Layer of Cell Bioenergetic Functionalities, i.e., a Phylogenetic Memory Layer of Universal Cell Bioenergetic Functionalities
The layer of cell bioenergetic functionalities, as layer that contains functionalities of main bioenergetic engines of cell life and development, is localized in the innermost part of the model. The layer of cell bioenergetic functionalities includes the bioenergetic functionalities needed for life, including, functionalities responsible, inter alia, for the occurrence of the Pasteur, Crabtree, Kluyver and glucose effects (i.e., functionalities that constitute the functionalities of unified cell bioenergetics). Is should be noted that in accordance with the endosymbiotic theory, mitochondria are the early prokaryotic endosymbionts that entered a larger cell about 1.5 billion years ago (i.e., during the process of transition of prokaryotic cells into unicellular eukaryotic cells)
[56]. Because mitochondria constitute the main powerhouses the of cells, the other bioenergetic effects related to mitochondrial activity are part of the layer of cell bioenergetic functionalities.
3.2. Layer of Unicellular Functionalities, i.e., a Phylogenetic Memory Layer of Atavistic Functionalities
The activity of functionalities of the cell bioenergetic layer has to be controlled (i.e., integrated and synchronized by appropriate stimulation and inhibition) to meet the cell’s bioenergetic requirements. According to the proposed model, during evolution of unicellular organisms, functionalities of the unicellular layer were formed that used, controlled and extended the functionalities of the cell bioenergetic layer. This layer (i.e., the layer of unicellular functionalities) provides basic life functionalities, including competition, survival and proliferation of unicellular organisms. These functionalities belong to the most conserved and protected functionalities supported by a myriad of built-in redundant pathways
[26]. The plethora of built-in redundant pathways also indicates that these functionalities are very elastics and adaptive, i.e., they can remain active in a heavily altered environment, despite changes in the genome.
In accordance with the information presented in Table 1 in
[55], the other functionalities of the unicellular layer are related, among other factors, to (a) unregulated, rapid and aggressive angiogenesis; (b) unregulated cell proliferation; (c) the lack of a Hayflick’s limit; (d) relief from curfew and checkpoints; (e) wound healing that does not stop stem-cell-like behavior; (f) unregulated epithelial–mesenchymal transition (EMT) migration; aggressive invasion and metastasis; (g) aerobic glycolysis; and (h) unregulated and truncated cell-differentiation cascades.
It should be added that many of the developmental requirements for multicellular organization (including functionalities of cell adhesion, cell–cell communication and coordination, and programmed cell death) probably existed in ancestral unicellular organisms
[57].
3.3. Layer of Multicellular Functionalities, i.e., a Phylogenetic Memory Layer of Multicellular Advanced Functionalities
The evolution of multicellularity required the integration of single cells into new functionally, reproductively and evolutionary stable multicellular organisms
[58]. This process required new functionalities. According to the proposed model, during evolution of multicellular organisms, functionalities of the new layer were formed that could use, control and extend both the functionalities of the layer of unicellular functionalities and layer of cell bioenergetic functionalities (
Figure 1). This layer (i.e., the layer of multicellular functionalities) provides complex life functionalities to multicellular organisms, including extended functionalities of proliferation, cell–cell communication, coordination, integration, adhesion to neighboring cells, signaling to maintain adhesion and programmed cell death. These functionalities are presented in
Figure 1 as extended multicellular functionalities.
In accordance with the information presented in Table 1 in
[55], the other functionalities of the multicellular layer (that hide/control functionalities of the unicellular layer) are related, among other factors, to (hidden/controlled functionalities are written in square brackets): (A) normal well-regulated angiogenesis [unregulated, rapid and aggressive angiogenesis]; (B) well-regulated cell proliferation, along with signaling, to control proliferation [unregulated cell proliferation]; (C) Hayflick’s limit and functionality of p53 [no Hayflick’s limit]; (D) cell cycle checkpoints [relief from curfew and checkpoints]; (E) signaling bringing to an end wound healing [wound healing that does not stop stem-cell-like behavior]; (F) regulated release [unregulated epithelial–mesenchymal transition (EMT) migration, aggressive invasion and metastasis]; (G) facultative switching between oxidative phosphorylation and aerobic glycolysis [aerobic glycolysis]; and (H) normal, well-regulated cell differentiation [unregulated and truncated cell-differentiation cascades].
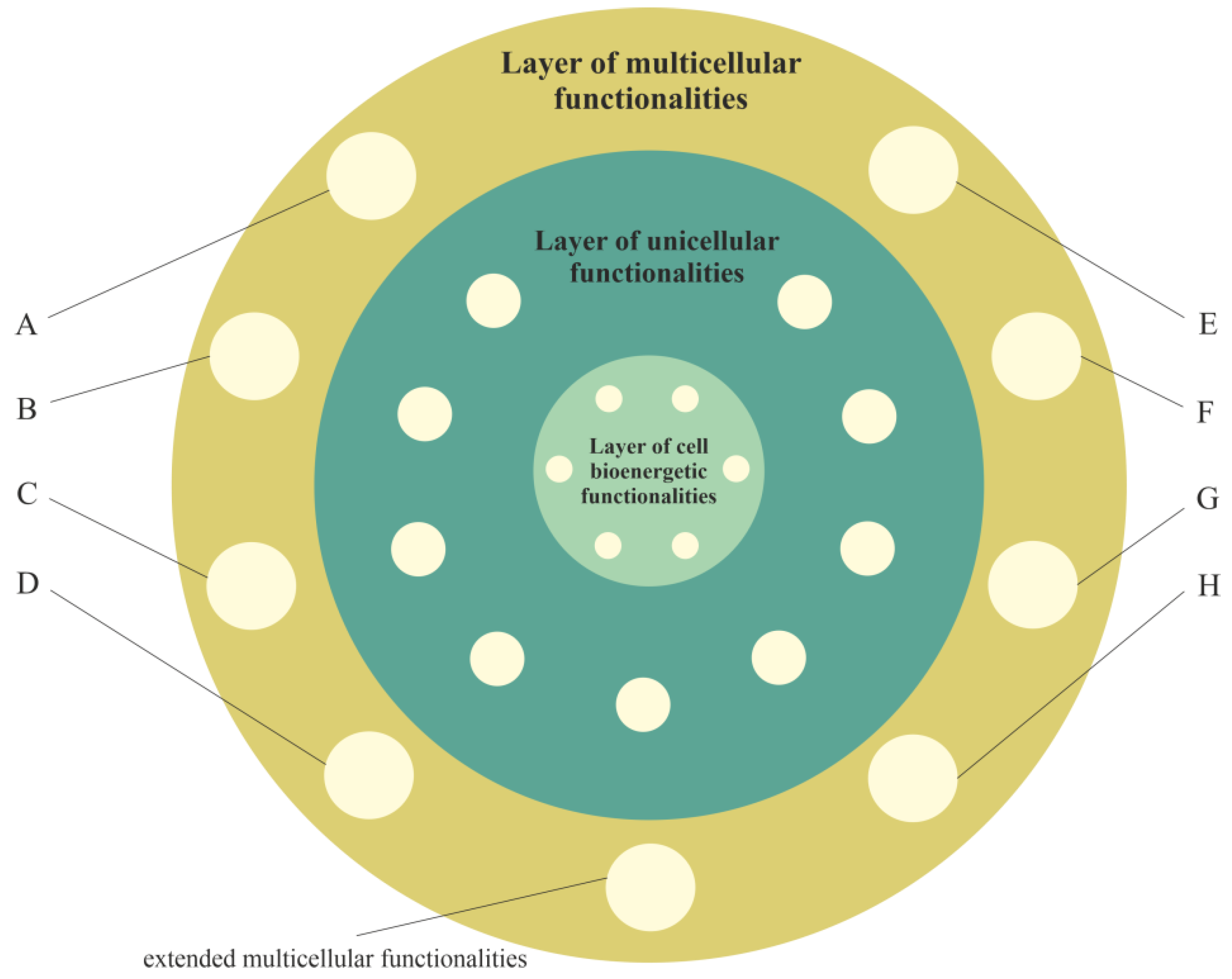
Figure 1. The layer of multicellular functionalities (i.e., multicellular layer) directly inherits from the layer of unicellular functionalities and indirectly from the layer of bioenergetic functionalities, i.e., functionalities of the third layer can use, control (including deactivation) and extend inherited functionalities. Selected functionalities of the multicellular layer include (A) normal well-regulated angiogenesis; (B) well-regulated cell proliferation, along with signaling, to control proliferation; (C) Hayflick’s limit and functionality of p53; (D) cell cycle checkpoints; (E) signaling bringing to an end wound healing; (F) regulated release; (G) facultative switching between oxidative phosphorylation and aerobic glycolysis; and (H) normal, well-regulated cell differentiation.
3.4. Cancer Transformation as a Loss of Control over Atavistic Functionalities
Functionalities of the multicellular layer are located in the most external layer of the layered model of evolution of cellular functionalities. In accordance with unified cell bioenergetics, bioenergetic cell problems, especially overenergization of mitochondria, can lead to cancer transformation [30][33]. Cell overenergization (and the related increase in ROS) is followed by adaptation of multiple metabolic strategies to solve this bioenergetic problem [46]. As a result, in light of the layered model of evolution of cellular functionalities, the cell’s response to a huge bioenergetic problem related to overenergization is propagation of disturbances in genome expression from the most internal (i.e., from the layer of bioenergetic functionalities) toward the more external layer (i.e., toward the layer of multicellular functionalities). Functionalities that are localized in the layer of multicellular functionalities, as the most complex and evolutionarily youngest (see Introduction), are the most sensitive to disturbances. The disturbance (or destruction, for example by, high ROS levels) of multicellular layer functionalities can result in a loss of control over functionalities of the unicellular layer, leading to cancer transformation, i.e., uncontrolled activity of atavistic functionalities (Figure 2).
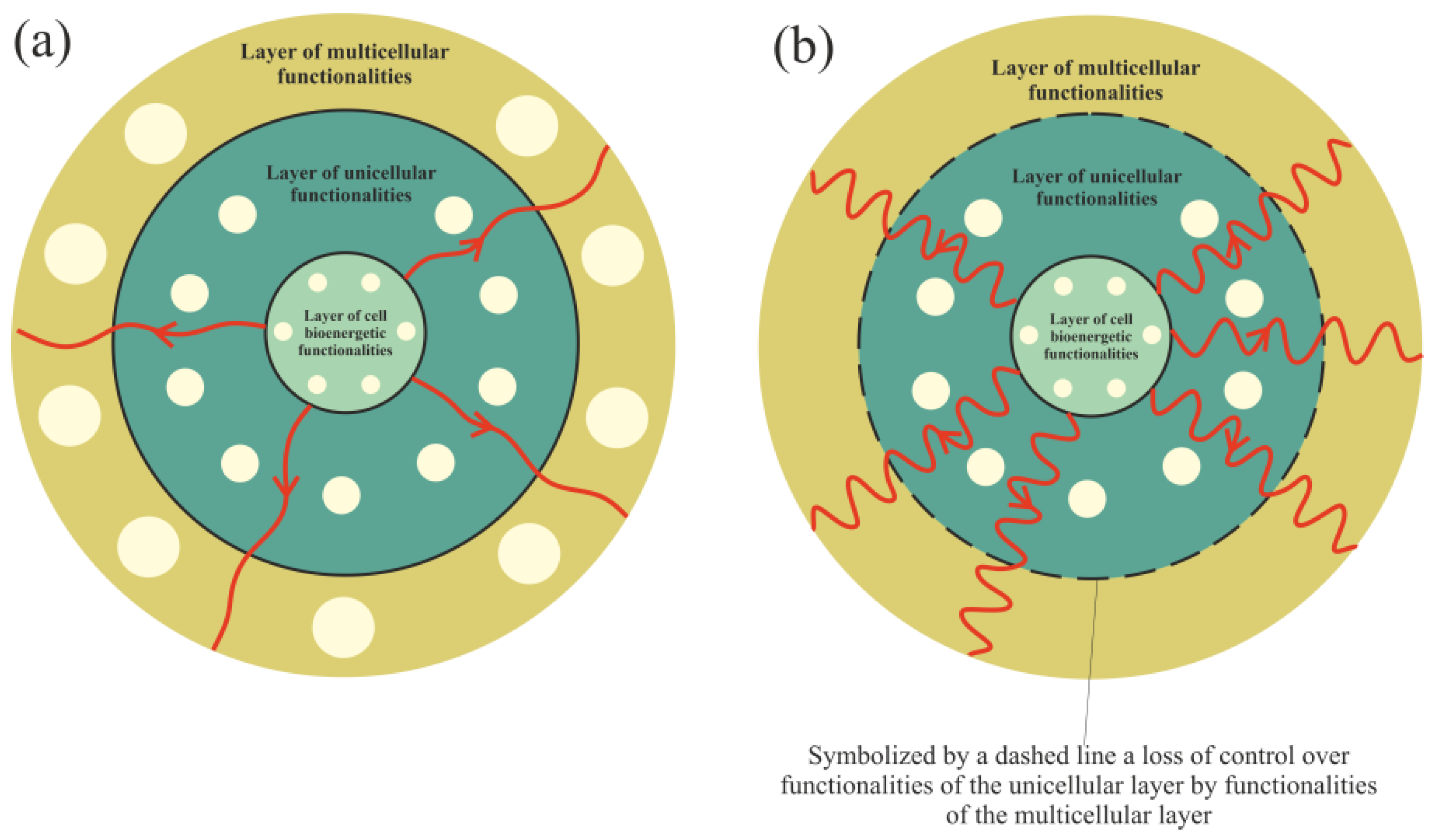
Figure 2. Propagation of disturbances in cell bioenergetics. (a) – Small disturbances cause small disturbances in functionalities of the unicellular and multicellular layers. (b) – Huge disturbances can cause a loss of functionalities of the multicellular layer (or huge disturbances in their operation), leading to a loss of control over unicellular layer functionalities.
This entry is adapted from 10.3390/ijms23074017