Theories concerning the origin of life on Earth began with captivating myths, prevalent in all cultures, describing how the heavens, Earth, and life were created by powerful supernatural beings. The ancient Greeks were the first of the cultures to leave a written history suggesting a materialistic origin, arguing that life evolved out of the essential elements; water, earth, air, and fire. Over the last 2000 years, however, most of humanity has preferred mysticism over materialism and has accepted either the monotheistic Judaeo-Christian-Islamic conception of an omnipotent supernatural being who breathed life into clay models, or to an eternal universe with life of no ultimate origin.
With the European Renaissance beginning in the 15th century came a re-invigoration of the art of inquiry and observation, known as “science”, first championed by the ancient Greeks. Materialistic ideas of the origin of life once again became popular, for example, the re-adoption of the ancient Greek idea that something as developed as a worm, a fly, or a frog could emerge from mud, dirty clothes, or a puddle of dirty water; the idea of “spontaneous generation” which remained prevalent until the middle of the 19th century.
Even these currently popular, materialistic, and more science based theories for the origin of life, however, lack axiomatic foundations based in physical law and instead settle for describing only the chemical synthesis of as many fundamental molecules of life (common to all three life domains; archea, bacteria, eukaryote) as possible, as efficiently as possible, and from an as limited set of precursor molecules as possible. All such theories, however, share a similar characteristic of their mystical counterparts; they rely on the unsettling premise that Nature found an apparently unique, almost miraculous, chemical reaction set 3.9 billion years ago, endowing these molecules with Darwinian like characteristics of reproduction with small variation and selection based on either efficacy of molecular precursor sequestration or molecular stability. Suffice to say that no such fortuitous chemical reaction set has yet been discovered.
Today, how the fundamental molecules of life could have been synthesized from simpler common precursor molecules, before the existence of the complex bio-synthetic pathways, is considered basically a solved problem in origin of life research. In fact, many different chemical and photochemical routes, under different chemical/physical environments, to the production of these molecules, from what would have been commonly available precursor molecules, have been discovered over the past 70 years of experimentation since the first results of Stanley Miller.
Life, however, is much more than a simple collection of fundamental molecules arranged in a particular pattern. This can be seen most clearly from the fact that a recently dead organism contains all of the molecules and their arrangement of a living organism, but there is an obvious difference between a recently dead animal and one which is alive, displaying a certain spirit or vitality to it. The collection of molecules in the living organism manifests macroscopic dynamical processes such as; mobility, metabolism, homeostasis, replication/proliferation, and the ability to evolve and adapt to different environments. Clearly, there is something more to be explained concerning the origin of life than the mere description of efficient chemical reaction routes to the fundamental molecules.
Boltzmann saw the process of life as a struggle of organisms to obtain and maintain their organization, and his analysis showed that this could only come at the expense of disorganization in the environment. In particular, Boltzmann realized that the organization of material in living organisms is allowed by the disorganization of the energy given off by the Sun and received by Earth. The words “organization” and “disorganization” are used loosely here to refer to the probability distribution of certain conserved quantities of Nature inherent to material; energy, momentum, angular momentum, and charge, over microscopic degrees of freedom of the material. The “microscopic degrees of freedom” refer to ways of storing energy, momentum, angular momentum, and charge at the microscopic level (for example, molecular translation, rotation, and vibration). When these conserved quantities are distributed over fewer degrees of freedom, researchers say that the system is “organized” and has low entropy (for example the kinetic energy of a hammer before it hits a piece of metal), and when distributed over many degrees of freedom, researchers say the system is “disorganized” and has greater entropy (after the hammer crashes into the metal its kinetic energy of motion is converted into heat—you can actually do the experiment and feel the metal heat up). What researchers call the “entropy increase” for this event is a measure of the greater distribution of the initial hammer kinetic energy over many more microscopic degrees of freedom which correspond to the atomic vibrations and electron motions in the metal, and eventually over the microscopic degrees of freedom of the environment, the surrounding air and ground.
It will be shown in this entry that the relevant conserved quantity involved in the dissipative structuring at the origin of life is energy, in particular, incident photon energy, and that the relevant microscopic degrees of freedom involved in the dissipation of this energy are molecular and involve electronic excitation, molecular vibration and molecular reconfiguration (e.g., new covalent bonding between the atoms), leading to heat (molecular vibrations giving photon emission at longer wavelengths). These microscopic material degrees of freedom are places where the low entropy incident photon energy is absorbed, redistributed, and then re-emitted as higher entropy radiation to the environment.
If the thermodynamic dissipation theory for the origin of life proves to be the correct theory, then, given particular, but probably common, environmental conditions on planets of hot K-type, G-type, and hotter stars (having an important UVC component), there will be, in fact, a thermodynamic imperative for an origin of life similar to human own based on carbon. In this case, the origin of life would not have been a single fortuitous chemical event constrained to perhaps a few “lucky” planets, but rather a thermodynamic imperative and would arise on many, if not most planets, of these types of stars (Figure 1).
In this case, human would certainly not be alone in the universe, because of the specific physical requirements for carbon based molecular dissipative structuring (e.g., UVC light limited to wavelengths greater than 205 nm, high temperature, neutral atmosphere, neutral solvent pH, etc.), developed complex lifeforms based on carbon, such as human know them today on Earth, may be relatively scarce. However, the first steps of life, which researchers' theory predicts would correspond to the “self-organization” of organic pigments, should be occurring on the surface of every planet circling any star which has at least some continuous light in the 205 to 285 nm UVC region and shielded by an atmosphere from wavelengths shorter than about 205 nm which can sequentially ionize carbon based pigments and thereby destroy them.
2. The Thermodynamic Dissipation Theory of the Origin and Evolution of Life
The proposed thermodynamic dissipation theory suggests that life arose as dissipative structuring of UVC pigments on the surface of Earth’s oceans to augment the global entropy production through UVC photon dissipation. In fact, any dissipative structure, also known as an “irreversible process”, originates and persists for only this fundamental physical reason; to distribute the conserved quantities of Nature (in this case energy) over ever more microscopic degrees of freedom, i.e., to produce entropy.
Currently, living organisms, known as phytoplankton (microorganisms such as cyanobacteria and diatoms), and other organic compounds such as free floating viruses, RNA and DNA fragments, pigments, lipids, and other organic molecules can be found in high density at the ocean surface microlayer. This set of microorganisms and molecules absorb an important part of sunlight and convert this light into heat which is then transmitted to the surface water. If the ocean surface was devoid of this organic material, only the infrared and the far ultraviolet light would be absorbed strongly by the surface water
[15], with the rest of the solar spectrum being reflected to space or transmitted to deeper ocean depths. The existence of this thick soup of microorganisms, organic molecules, and water at the surface thus allows not only the absorption of the infrared and far ultraviolet light, but also the visible and the near ultraviolet parts of the solar spectrum (essentially the whole solar spectrum). The heat of photon dissipation at the surface then turns liquid water into vapor (evaporation) which can then participate in the water cycle, a second coupled dissipative process, further increasing the shift of the incident light towards the infrared, i.e., increasing global entropy production
[16].
Evaporation produces clouds which are blown inland and cause rain on land. Minerals washed out of the rocks by the rain foment the growth of terrestrial plants that absorb sunlight better than bare sand and rock through organic pigments such as chlorophyll
[14]. Some of the minerals washed out of rocks on land end up in rivers which eventually flow into the oceans where these nutrients stimulate greater phytoplankton growth. The absorption of sunlight through organic molecules at the ocean surface thus promotes the water cycle and the water cycle in turn stimulates the growth of life on both land and the ocean surface, thereby stimulating even more evaporation
[16]. This is an example of auto-catalytic coupling of different irreversible thermodynamic processes (photon absorption in phytoplankton and plants with the water cycle). In the coupling of different irreversible processes, the “product” of one process acts as a generalized thermodynamic force for another irreversible process, and, as such, is a form of more complex dissipative structuring where each irreversible process is only one part of a much greater dissipative system (e.g., the biosphere
[17]) leading to even greater global photon dissipation. The coupling of the dissipation of light by organic molecules on the Earth’s surface with the water cycle accounts for more than 63% of all the entropy generated by the Sun-Earth interaction
[18]. Most of the rest of the entropy production is due to light absorption on atmospheric molecules (e.g., water vapor, ozone, and water soluble organic dust) which, in fact, all owe their existence primarily to life.
Life, then, removes a restriction to entropy production on Earth by absorbing and dissipating light more efficiently and over a much wider spectral region, where, in fact, the Sun is most intense. Given that the dissipation of photons is the most relevant thermodynamic work performed by life today, it is very likely that this was also its function 3.85 billion years ago, a date for which there is evidence in the form of the ratio of carbon isotopes (life prefers to incorporate the lighter carbon isotope into its structures) that life originated
[19].
Due to a lack of oxygen and ozone (before the invention of oxygenic photosynthesis), Earth’s atmosphere at the time of the origin of life allowed the free passage of light within wavelengths between approximately 205 and 285 nanometers (nm), which is in the ultraviolet (long wavelength part of the UVC). At the same time, there was less near UV and visible light (between 320 and 700 nm) due to the fact that the Sun’s radius was smaller at the origin of life than today
[20]. Photons in this long wavelength (205 to 285 nm) part of the UVC region were ideally suited for dissipative structuring because they have enough energy to promote the breaking and remaking of covalent bonds of carbon based precursor molecules (like HCN and CO2 in water
[13]), but not enough energy to successively ionize, and thereby destroy, these molecules.
HCN is a common molecule found throughout the cosmos and its production during the Hadean and Archean on Earth was probably a result of the solar Lyman alpha line (121.6 nm) photo-lysing N2 in the upper atmosphere which then attacks CH or CH2 to form HCN
[21], or the UV (145 nm) photolysis of CH4 leading to a CH∗ radical which attacks N2
[21]. HCN and its hydrolysis product formamide are now recognized as probable precursors of many of the fundamental molecules of life, including nucleic acids, amino acids, fatty acids
[22], and even simple sugars
[23][24]. As early as 1875, E. Pflüger suggested that life may have followed from “cyanogen compounds”
[25]. The ubiquity of different chemical and photochemical routes from HCN to the fundamental molecules discovered over the last 60 years has led to the suggestion of an “HCN World”
[26][27] occurring before the postulated “RNA World”
[28].
It is conceivable, then, that life began as pigments dissipatively structured from HCN in water under UVC light to absorb and dissipate photons in this spectral region. The eventual dissipative structuring of the nucleobase adenine, and the related energy storage molecule adenosine-triphosphate (ATP), would allow dissipative structuring to occur under the higher intensity, but lower energy, visible wavelengths, by using, not one, but various visible photons together to transform molecules through the chemical potential which would be stored in ATP. This would allow still more complex biosynthetic pathways to emerge based on visible photons, such as a primitive photosynthesis, leading to the dissipative structuring of still more pigments, now in the visible, and giving rise to new pathways and catalysts for photon dissipation, eventually leading to complex ecosystems, corresponding to the organization of material in a form very efficient for photon dissipation on Earth's surface
[14].
It must be emphasized that in researchers' thermodynamic perspective of life, biological evolution is not independent from other abiotic irreversible processes in the environment and that all coupled biotic-abiotic evolution is directed by the same thermodynamic imperative of increasing photon dissipation, or, in other words, entropy production.
2.1. Evidence for the Theory
RNA, DNA, and most of the fundamental molecules of life, efficiently absorb and dissipate UVC photons within a spectral region of about 205 to 285 nm, just the wavelengths of the Archean atmospheric window (see Figure 2).
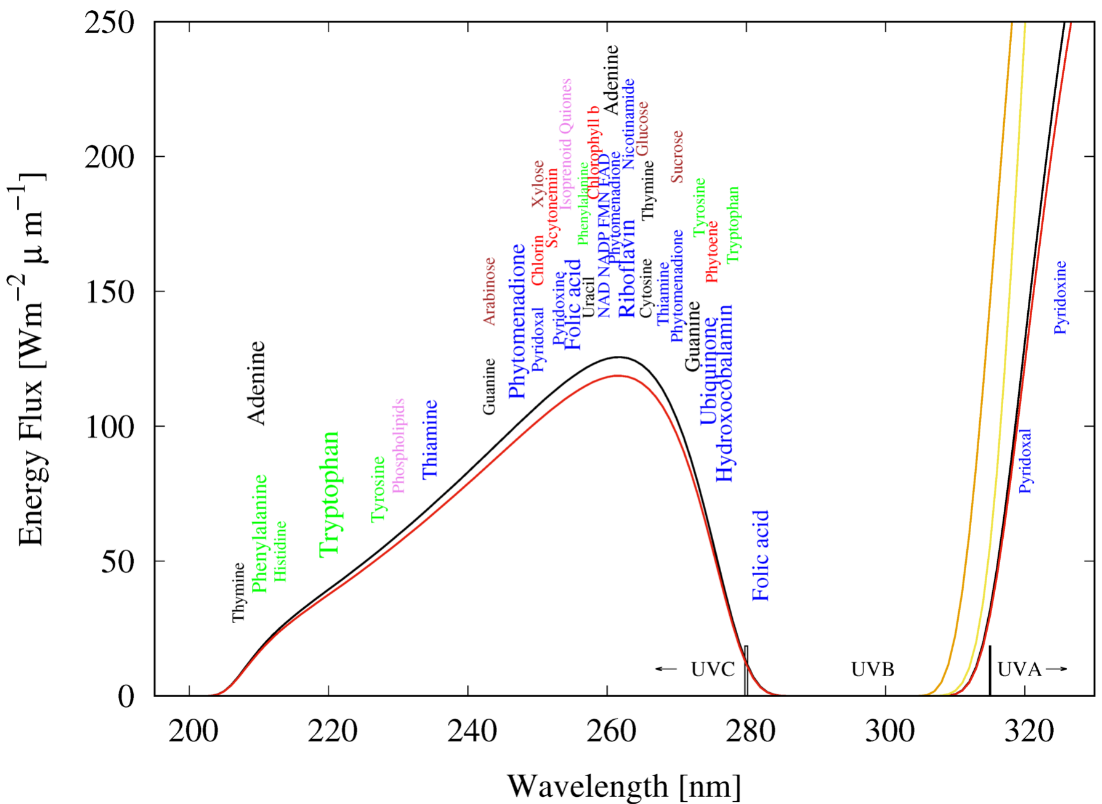
Figure 2. The spectrum of light available in the different UV regions at Earth’s surface before the origin of life at approximately 3.85 Ga and until at least 2.9 Ga (curves black and red, respectively) during the Archean. CO2 and probably some H2S were responsible for absorption at wavelengths shorter than ∼205 nm and atmospheric aldehydes (common photochemical products of CO2 and water) absorbed between about 285 and 310 nm
[29], approximately corresponding to the UVB region. Around 2.2 Ga (orange curve), UVC light at Earth’s surface had been extinguished by oxygen and ozone resulting from organisms performing oxygenic photosynthesis. The yellow curve corresponds to the present surface spectrum. Energy fluxes are for the sun at the zenith. The names of the fundamental molecules of life, nucleic acids (black), amino acids (green), fatty acids (violet), sugars (brown), vitamins, co-enzymes and co-factors (blue), and pigments (red) are plotted at their wavelengths of maximal absorption (the font size of the letter roughly corresponds to the relative size of their molar extinction coefficient, i.e., how well they absorb). Note that these wavelengths of maximum absorption coincide well with the Archean UV surface spectrum. Adapted from Michaelian and Simeonov
[11].
On exposure to UVC light, the nucleobases of RNA and DNA (adenine, guanine, cytosine and thymine or uracil) and also many of the other fundamental molecules of life, are electronically excited (collective excitation of electrons) and decay extremely rapidly (<2 pico seconds, i.e., 2×10−12 s) through a process known as internal conversion, which converts the electronic excitation energy due to the absorbed photon rapidly into heat (vibrational motion of the atoms). The nucleobases do this by morphing into a very specific geometry once in their electronic excited state, and this allows the vibrational states superimposed on the electronic excited state to couple with the vibrational states superimposed on the electronic ground state. Such a result, as viewed from the potential energy surface, is known as a conical intersection (see Figure 3).
Figure 3. Conical Intersection (CI) for adenine (one of the nucleobases) showing the degeneracy of the electronic excited state with the electronic ground state after a UVC photon absorption event (blue arrow) which induces a nuclear coordinate deformation, known as pyrimidilization, from its original structure in the Franck-Condon (FC) region. In this deformed state, the vibrational states of the excited potential energy surface coincide in a region (CI) with the vibrational states on the ground state. Conical intersections provide rapid (sub-picosecond) dissipation of the original electronic excitation energy into heat. The quantum efficiency (q) for this dissipative route is very large, making adenine photochemically stable but, more importantly, very efficient at photon dissipation. Another common form of coordinate transformation associated with conical intersections are proton transfers within the molecule or with the solvent. Based on data from Andrew Orr-Ewing
[30], Roberts et al.
[31], Kleinermanns et al.
[32] and Barbatti et al.
[33]. Taken with permission from Michaelian
[13].
This extremely fast dissipation of the electronic excitation energy through such a conical intersection makes RNA, DNA, and the other fundamental molecules of life (which also have them), extremely efficient at photon dissipation and stable against further photo-reactions that could modify their structure. This is because reactions occur preferentially in the excited state and the lifetimes of these excited states for molecules which have a conical intersections are simply too short for a further reaction to occur.
The extremely rapid de-excitation of the fundamental molecules also means, by the Heisenberg uncertainty principle ΔEΔt≥ℏ, that they also absorb over a large wavelength region (i.e., ΔE is large since Δt is small; here E is the energy, t is time, and ℏ=h/2π where h is Planck’s constant) (see Figure 4). As can be imagined, this has important consequences for the “thermodynamic selection” of these particular molecules with conical intersections over other possible configurations because even electronic excitation by light of significantly different wavelength will also dissipate rapidly through the same conical intersection.
The strong absorption of the fundamental molecules over the UVC spectral region arriving at Earth’s surface during the Archean (Figure 2), the fact individual fundamental molecules absorb over exactly the same region (compare Figure 2 and Figure 4), and the fact that many of the fundamental molecules also have a conical intersection for the rapid dissipation of the absorbed energy into heat, is strong evidence that the fundamental molecules were microscopic dissipative structures, i.e., originally UVC pigments.
Figure 4. Absorption spectrum as a function of temperature for a 25 base-pair double helix DNA sample. An increase in absorption occurs with denaturing as the temperature is raised, known as the “hyperchromic effect”. The lower curves (right y-axis) are the spectrum differences taken about a 3 degree temperature bin at the specified temperature. Because of the Heisenberg uncertainty principle ΔEΔt≥ℏ, strong absorption occurs over a wide region of wavelength (ΔE large) since most of the de-excitation is very rapid (Δt small) through the conical intersection. This fundamental molecule of life absorbs strongly over most of the region that was arriving at Earth’s surface during the Archean (compare with
Figure 2). Taken with permission from Michaelian and Santillán
[34].
There also exists empirical evidence suggesting selection for traits optimizing UV exposure for particular amino acids complexed with their RNA or DNA cognate codons or anticodons, particularly for those amino acids displaying the strongest stereochemical affinity to DNA and RNA. Such amino acids are, for example, the aromatic amino acids, which could have acted as auxiliary antennas to DNA and RNA for greater UVC light dissipation, and amphipathic (having one part hydrophilic and the other part hydrophobic) amino acids acting as anchors for DNA and RNA to the ocean surface where light intensity is maximal. This has led researchers to suggest
[35] that UVC photon dissipation was the basis of the initial specificity in the amino acid—nucleic acid association during an early stereochemical era
[36]. If this were the case, the information content of DNA and RNA would have been initially related to optimizing UVC photon dissipation. Today, the collective information content of the DNA and RNA of all living organisms is related to optimizing photon dissipation for the biosphere, from the UVC, through the visible, and up to (and even beyond) the red-edge.
Perhaps the most convincing evidence of all, however, is that many photochemical routes to the synthesis of nucleic acids, amino acids, fatty acids and sugars from simple, presumably common, precursor molecules (such as H2O, HCN, and CO2) have been identified at these wavelengths (205–285 nm) and the rate of photon dissipation within the Archean atmospheric window generally increases after each incremental step on route to synthesis
[37][38], a behavior strongly suggestive of dissipative structuring.
In the following, researchers present a brief overview of the microscopic dissipative structuring of one class of fundamental molecules of life, the nucleic acids. More details, and the dissipative structuring of the other classes, e.g., the fatty acids, is given elsewhere
[13][38].
2.2. The Dissipative Structuring of the Nucleic Acids
In 1924, Alexander Oparin published a treaties on the origin of life
[39] in which he suggested production of life’s molecules from an atmosphere of reducing gases like hydrogen, ammonia, and methane, subjected to the heat and electric discharge from lightening. Similar ideas were also developed by John Haldane in 1929 who put forward the idea of a primordial organic soup
[40].
In 1953, Stanley Miller proposed to his graduate adviser Harold Urey that they test Oparin’s suggestion, and designed and conducted experiments
[41][42] by heating, and subjecting to electrical discharge, a reducing atmosphere containing ammonia, methane, hydrogen, and water and confirmed the production of 5 amino acids; glycine, α-alanine, β-alanine, aspartic acid and α-aminobutyric acid. Recently, Jeffrey Bada (a student of Miller) performed a reanalysis with modern equipment of Miller’s products (including some results obtained by using an atmosphere of H2S and CO2 preserved for all these years in glass flasks). Bada showed, in fact, that not only 5, but many of the 22 amino acids used by life were produced in the Miller experiments
[43]. Most of these α-amino acids are secondary products arising from HCN polymers after being hydrolyzed (reacting with water), a much simpler route to their production than the usually invoked Strecker synthesis (synthesis of amino acids by the reaction of an aldehyde with ammonia in the presence of potassium cyanide)
[44].
More recently, however, the scientific community has come to the consensus that the atmosphere at the beginning of the Archean, when life arose, was probably not as reducing as proposed in the Miller–Urey experiments. It is now suggested instead that the early atmosphere (after the end of “late lunar bombardment”, around 3.9 Ga) was produced by oxidized gasses coming from the magma of volcanoes, as evidenced from the degree of oxidation of metals preserved within sturdy zicron crystals of the era. Therefore, some other form of production of the fundamental molecules from a more neutral atmosphere was probably more likely (although induced lightening strikes on H2S and CO2 within gas and ash clouds coming from volcanic eruptions could, conceivably, be a distinct possibility for the production of the amino acids containing sulfur).
Experiments by Óro and Kimball in 1962
[45], as well as those by Ferris and collaborators
[46], were able to obtain not only simple amino acids, but also the RNA and DNA purine and pyrimidine nucleobases from HCN in water at high temperature. In 1966, Ferris and Orgel
[47] were able to obtain an even more copious production of the purine nucleobase adenine with HCN in cooler water exposed to UVC light (
Figure 5). The fact that many of these fundamental molecules have now also been found in meteorites, on the surface of comets, and even in the very cold interstellar gas and dust clouds, hints at the utility of UV light as a free energy source for their production under a myriad of different physical conditions. Which were, therefore, the chemical or photochemical routes that brought these first molecules to the surface of Earth?

Figure 5. The photochemical synthesis of adenine from 5 molecules of hydrogen cyanide (HCN) in water, as discovered by Ferris and Orgel (1966)
[47][48]. Four molecules of HCN are transformed into the smallest stable oligomer (tetramer) of HCN, known as cis-2,3-diaminomaleonitrile (cis-DAMN) (2), which, under a constant UVC photon flux isomerizes into trans-DAMN (sometimes labeled as iaminofumaronitrile, DAFN) (3) which may be further converted on absorbing two more UVC photons into an imidazole intermediate, 4-amino-1H-imidazole-5-carbonitrile (AICN) (7). Hot ground state thermal reactions with another HCN molecule or its hydrolysis product formamide (or ammonium formate) leads to the purine adenine (8). This is a microscopic dissipative structuring process which ends in adenine
[37], a pigment with a large molar extinction coefficient at 260 nm and a peaked conical intersection which promotes the dissipation of photons at the wavelength of maximum intensity of the Archean solar UVC spectrum (
Figure 2). Taken with permission from Michaelian
[13].
An important clue to answering this question was given in the Introduction, where researchers emphasized that life is much more than a simple collection of fundamental molecules arranged in a particular pattern. Life manifests a vitality as seen in macroscopic dynamical processes such as; proliferation, mobility, metabolism, homeostasis, increases in complexity, and the ability to evolve and adapt to different environments. Unraveling this dynamical aspect of life by exploring the non-equilibrium thermodynamics of open systems should help answer the question of "which were the actual routes to the fundamental molecules?".
Lars Onsager (Nobel Prize in Chemistry 1968) and Ilya Prigogine (Nobel Prize in Chemistry 1977), starting from the original idea of Boltzmann that the ordering of material in space and time (dynamical processes, or the vitality of life) could occur only with a concomitant increase in the probability distribution of energy over microscopic degrees of freedom in another connected space-time region (the environment), showed that dissipative structuring of material arises to foment this process in non-linear systems held under an external force keeping the system from reaching equilibrium.
Just as a non-linear equation has various possible solutions, non-linear relations between the forces and flows gives rise to multiple possible stationary states (structuring in space and time) for the system. Those states, structures, or processes that disperse more efficiently the conserved quantities of nature over the microscopic degrees of freedom of the material of the environment can be shown to be the most stable
[13], and thus researchers will most likely find open systems in these higher entropy producing states (see below). This further means that natural fluctuations in the environment, or within the system itself, could cause the system to leave its present state and evolve towards those more probable states that produce greater dissipation of the free energy incident from the environment.
An example of one such system in which two stable states exist for the system under the same imposed external force (in this case a temperature gradient) is the Bénard cell. This system consists of a liquid sandwiched between a lower plate at high temperature and an upper plate at lower temperature, and under the force of gravity (see Figure 6). At small temperature gradients, the system is in the linear regime and a static homogeneous state results with only conduction of heat through the liquid from the lower plate. As the temperature gradient is increased, the system arrives at the non-linear regime (between force—temperature gradient, and flow—of heat) and dissipative structuring of convection cells becomes physically possible (the upward buoyant force of hot liquid overcomes the opposing frictional force due to viscosity). In this regime, there exists two states for the system at the same value of the impressed external force (temperature gradient), one in which the hot liquid comes up through the center of the cells and the other in which it comes up around the edges of the cells. The two states have different entropy production or, in other words, a different rate of the dissipation into the environment of the incident free energy coming from the temperature difference between the two plates. The state in which the hot liquid comes up through the center of the cell is the one with the greatest heat flow and thus more resistant to material or temperature fluctuations, and thus more probable and more stable state, and it corresponds to the state of greatest entropy production. This, therefore, is the state in which researchers should expect to find Nature most frequently, and, in fact, experiment verifies this.
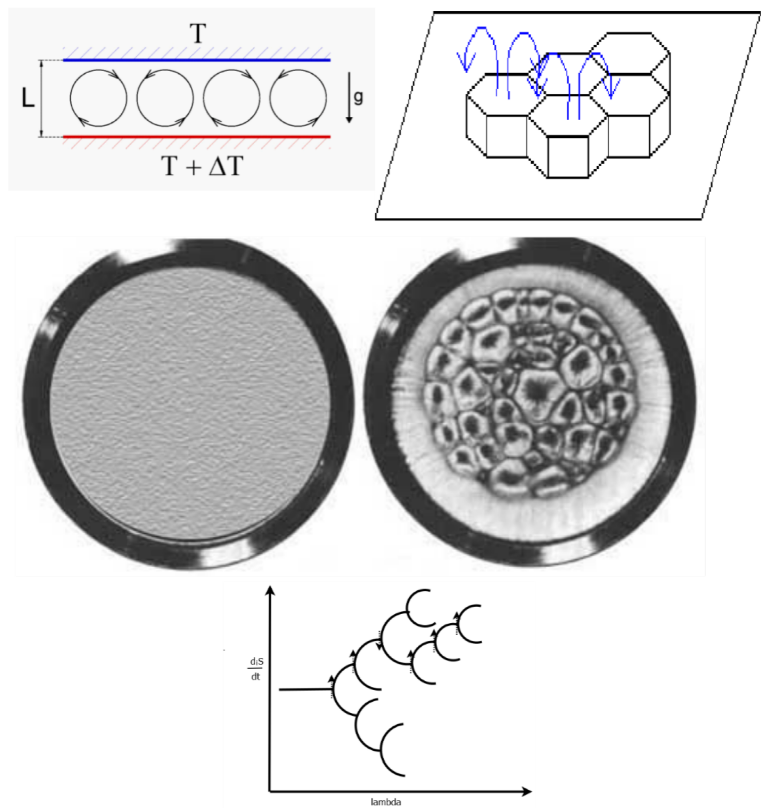
Figure 6. The Bénard cell apparatus consists of a hot lower plate and a cold upper plate with a liquid sandwiched between the two, with the system under the force of gravity. The onset of the dissipative structuring of the convection cells occurs as a result of an external force (temperature gradient) imposed over the system, and results from a non-linear relation between the flow of heat and the temperature gradient. The left part of the middle figure depicts the view from above for a linear relation between the force (temperature gradient) and the flow (heat flow) when the temperature difference over the system is small, below some critical value ΔTc and results in a homogeneous system (no spatial symmetry breaking) and there exists only conduction of heat from the lower to upper plate. The right part describes the non-linear situation when the temperature difference between the two plates is greater than some critical value ΔTc for which convection cells spontaneously arise (Bénard cells) and spatial symmetry breaking occurs. There are two stationary states allowed for this system once the bifurcation point (critical temperature gradient) is reached; one with the hot liquid coming up through the center, and the other with the hot liquid coming up around the edges of the approximately hexagonal cells. The greater entropy producing diS/dt state is the one with the hot liquid coming up through the center of the cells and this is the state of greater probability and thus stability, given internal and external fluctuations of the material flow and temperature. This is shown in the bifurcation diagram at the bottom (see Figure 3) which describes the direction taken by the system in terms of entropy production as given by the arrows. The general trend is towards greater entropy production, but this is not always the case.
Similar phenomenology, researchers argue, was true for the case of microscopic dissipative structuring of the fundamental molecules of life near the beginning of the Archean. The system probably consisted of precursor molecules such as HCN in water contained within a lipid vesicle under a UVC photon flux similar to that given in
Figure 2 (see
Figure 7). Many different states, corresponding to different concentration profiles of the different possible intermediate and final product molecules resulting from the photochemical reactions existed
[13]. Those molecular concentrations profiles which were the most efficient at dissipating UVC photons, i.e., having greater absorption over a greater wavelength region in the UVC, and a conical intersection for rapid dissipation into the infrared, i.e., characteristics most like a black-body
[14], are those states (concentration profiles) that are most stable and thus these are the most probable, or statistically selected in this non-equilibrium thermodynamic sense.
Figure 7. Fatty acid vesicle floating at the ocean surface microlayer, transparent to UVC light and permeable to the small molecules H2O, HCN and formimidic acid (Fa) but impermeable to the photochemical reaction products (e.g., ammonium formate (Af), AICN, adenine (A)) which are larger in size and have larger dipole moments. Concentration profiles of the intermediate and final product molecules which are most effective at dissipating the incident UVC photon flux are the most stable and therefore statistically selected in this non-equilibrium thermodynamic sense. Taken with permission from Michaelian
[13].
These final product molecules with conical intersections are more stable since, although the incident photons excite these molecules in the same way they do the intermediate molecules, the excited state lifetimes of the final product molecules are decreased. This is because these are the molecules with large quantum efficiency for de-excitation directly into heat through a conical intersection, instead of into some new configuration (implying greater stability under this UVC light which is necessary for their structuring). The excited state lifetimes of these final product molecules are thus so short that further reactions simply do not have time to occur (molecules are most reactive in their electronic excited state). Since their lifetimes are very short, the molecules return rapidly to the ground state and are ready to receive another photon, giving these final product molecules greater dissipative efficacy than the intermediates or original precursors. They are thus more stable in this non-equilibrium thermodynamic sense (less chance of suffering further chemical reconfiguration under the impressed UVC light), not in the equilibrium sense of having lower Gibb’s free energy. It is known, for example, that the natural nucleobases have conical intersections but they are not the lowest Gibb’s free energy tautomers, and that these later do not have conical intersections.
The microscopic details of exactly how dissipative structuring occurs for the nucleobase adenine from HCN in water under UVC light, are given elsewhere
[13][37].
Early life may thus be identified with a particular form of non-equilibrium structuring; microscopic dissipative structuring of carbon based molecules under UVC light leading to a concentration profile of product molecules particularly efficient at photon dissipation. The synthesized products, the fundamental molecules of life, are thus created as UVC pigments endowed with peaked conical intersections which gave them stability for long periods due to the reduced quantum efficiency for dexcitation through further photochemical reaction pathways. Unlike macroscopic dissipative structures such as hurricanes or convection cells, at normal Earth temperatures these microscopic dissipative structures remain intact even after the removal of the imposed UVC light potential driving their synthesis due to strong covalent bonding between atoms.
2.3. Proliferation and Selection
In auto- or cross-catalytic chemical reactions (those in which one of the reaction products acts as a catalyst for the reaction), and for systems kept out of equilibrium by a continuous supply of precursor reactant molecules to the system, the concentration of the product catalyst within the system can increase many orders of magnitude over the concentration expected for a similar system near equilibrium. Over 50 years ago, Ilya Prigogine
[8] demonstrated, through Classical Irreversible Thermodynamic (CIT) theory, that this result has its origin in the non-linear relationship between forces (chemical affinities divided by the temperature) and corresponding flows (the reaction rates) and results in system evolution towards greater entropy production. That Nature favors these auto-catalytic or cross-catalytic reactions can be imagined quite easily since a catalyst increases the rate of the reaction and thus if the reaction is auto-catalytic the rate of catalyst production will increase accordingly, establishing a positive feed back loop. This auto-catalytic reaction also increases the rate of dissipation of the chemical potential (entropy production), thereby reducing the amount of chemical potential available to competing reactions.
The situation is similar for the photochemical reactions in the dissipative structuring that led to the origin of life. If intermediate product molecules on route to the dissipative synthesis of the fundamental molecules act as catalysts for the photochemical or chemical reactions, then this would lead to their proliferation, as well as to that of their final product molecules. Those intermediate or final product molecules which are most rapid in dissipating the photon-induced excited state energy into heat will, by the Heisenberg uncertainty principle, absorb over a greater wavelength region (see Figure 4) and thereby reduce the available photochemical potential to other competing photochemical reactions. These photochemical reactions will therefore be the most probable (having largest quantum efficiency) and have greatest photon dissipation efficacy (entropy production). Efficacy for dissipation is therefore selected for, and this, along with proliferation, provides a mechanism for evolution which may be termed dissipative selection, or more generally, thermodynamic selection.
2.4. Nucleic Acid Polymers
Researchers have given a brief explanation of how the fundamental molecules of life were dissipatively structured into UVC pigments from HCN, CO2 and H2S in water under UVC light. Much more detail can be found in Michaelian
[13]. This “spontaneous” re-organization of covalently bound atoms in molecules under UVC light leading to dissipative UVC pigments is analogous to the formation of a convection cell, or hurricane, under a temperature gradient. Researchers also explained how these molecules proliferated over Earth’s surface because of their auto-catalytic and cross-catalytic nature in fomenting photochemical reactions leading to the same molecules, and researchers have suggested how intermediate and final product molecules dissipating more photons than others will be more probable (selected) under the incident photon flux. But, why and how did the nucleobases polymerize into RNA and DNA nucleic acids (
Figure 8), and why and how were these polymers faithfully replicated? If researchers' postulate of life’s origin being tied to dissipation is to be consistent, all processes, including the polymerization of the nucleotides into nucleic acid, and the replication of these, must also be attributable to increases in photon dissipation.
Figure 8. The nucleic acid polymer DNA. For polymerization of the nucleobases to be thermodynamically selected, it must have some photon dissipation enhancing characteristic. Bases are much more stable in polymer (less subjected to hydrolysis) but, more importantly, in polymer form one has a scaffolding which permits the attachment of molecules with UVC dissipation enhancing characteristics (for example, the amino acid tryptophan which may have acted as a UVC antenna). Image credit; Madprime (talk · contribs), CC0, via Wikimedia Commons.
It can be imagined that the nucleobases and other fundamental molecules formed within an out-of-equilibrium photochemical reaction system consisting of a fatty acid vesicle floating within the surface microlayer of a hot (∼80 °C
[49][50][51]) Archean ocean surface under the UV spectrum of
Figure 2 (see
Figure 7). Fatty acid vesicles form spontaneously on the ocean surface through minimizing the Gibb’s free energy and researchers have demonstrated elsewhere how the long-chain fatty acids themselves can be spontaneously dissipatively structured from CO2 in water under UVC light
[38]. The fatty acid vesicle is permeable at these high temperatures to small precursor molecules like HCN, CO2 or H2S, but impermeable to the larger products like the nucleobase adenine and ribose. Without going into the chemical details, since they have been presented elsewhere
[12][13][38], researchers assume that the nucleobases formed nucleosides with ribose sugar and could have been activated (phosphorylated) in the presence of phosphates under UVC light, forming the nucleotides, a scenario for which there is experimental evidence
[52][53].
Because of π-interactions between the aromatic rings of the nucleobases (a van der Waals interaction) and because of hydrophobic forces, there will be some natural stacking of the nucleotides one on top of the other in a water solvent, allowing phosphodiester bonds to form between a phosphate group and the 3′ and 5′ carbon atoms of two adjacent sugars to link and form the sugar-phosphate backbone of DNA or RNA (
Figure 8). Hydrolization of two phosphate groups gives enough free energy to overcome the barrier for formation of the phosphodiester bond. The condensation (dehydration) action of polyamidines (which are also components of HCN polymers) on nucleotides would also stimulate their polymerization
[44].
Once the bases are polymerized, they are significantly more stable than when they are isolated
[54], however, this alone cannot explain the polymerization since the Gibbs free energies are higher in the polymerized state. For consistency, researchers need an explanation from non-equilibrium thermodynamics related, again, to improvements in photon dissipation.
It is well known that some organic molecules can act as acceptor-quencher molecules to the electronic excitation energy of donor molecules if the acceptor and donor molecules are in close proximity
[55]. As mentioned above, the bases, as well as the polymers RNA and DNA, are superb quenchers, dissipating their electronic excitation energy within sub-picosecond times. They could, therefore, have acted as acceptors to quench the UVC induced excitation energy of other fundamental molecules of life having a large UVC absorption cross section but slower non-radiative decay times or large quantum yields for radiative decay such as fluorescence, both of which reduce the efficacy of dissipation. For example, the aromatic amino acid tryptophan absorbs strongly in the UVC but has a long non-radiative decay time of nanoseconds and a probability of getting trapped into an even longer lived triplet spin state of about 20% (leading to phosphorescence) compared to the probability for RNA and DNA doing the same of about 0.01%
[56][57]. Intercalation of tryptophan with single strand DNA, involving the stacking of the tryptophyl ring with the base ring, is known from monomer studies to lead to the total quenching of both the tryptophan and the base fluorescence
[58][59]. In double strand DNA, the quenching persists on intercalation and the double strand opens somewhat at the site of the tryptophan
[60].
The donor–acceptor complex of tryptophan together with RNA or DNA would have thus constituted a greater dissipating system than the sum of its individual molecular components and would therefore have been thermodynamically selected through a dissipation-replication mechanism to be described in the following section. Certain linear segments of native RNA and DNA having a particular base sequence do indeed have chemical affinity to tryptophan and to other UVC dissipating molecules. In fact, researchers have shown that codons and anti-codons (contiguous triplets of base pairs, each coding for a particular amino acid) of RNA and DNA, have strong chemical affinity to their cognate UVC antenna aromatic amino acids, as well as to other dissipation fomenting amino acids such as the amphiphilics (one part hydrophobic and the other part hydrophylic) which would anchor RNA and DNA to the ocean surface where the UVC light would be most intense
[36][61].
Polymerization of the bases would thus have provided a scaffolding for the attachment of other fundamental UV-C dissipating molecules, and this, through the donor-acceptor mechanism, would have led to a greater dissipating complex than the sum of its parts and so be thermodynamically selected. Besides leading to greater dissipation, since the affinity of the donor chromophore molecule (the aromatic amino acid) to the RNA or DNA acceptor is selective to only particular codons, this dissipation-replication mechanism would also have led to information accumulation in RNA and DNA (information for optimizing dissipation) and to a thermodynamic advantage, in terms of photon dissipation, to evolving a faithful replication mechanism
[61].
2.5. Polymer Replication
The nucleobases gained more stability once polymerized and served as scaffolding, and electronic excitation quenchers, for other fundamental molecules which acted as antennas, such as the aromatic amino acids, or anchors to the ocean surface, such as amphiphilic amino acids. A microscopic ecosystem of DNA-amino acid molecular complexes thus began to establish itself on Earth’s ocean surface to efficiently dissipate the UVC photons. How would only those complexes most efficient at dissipation be selected for replication and dispersal over the entire of Earth’s ocean?
Recall that DNA forms double helix chains that remain bound due to the hydrogen bonds that are formed between the nitrogenous bases and the stacking interaction between adjacent bases. When these polymers are in water and the temperature is increased, the double strands of DNA separate into single strands at a particular temperature known as its denaturing temperature. In general, the denaturing temperature of each DNA and RNA double strand depends on the amount of hydrogen bonds that it contains, and on the stacking interaction, and therefore, on the number of nitrogenous bases it has and the proportion of G-C base pairs (with 3 hydrogen bonds) compared to the number of A-T bonds (with 2 hydrogen bonds), and also on the pH and the amount of salt in the solvent. In general, for ocean-like environments, the denaturing temperature of intermediate length strands of double helix DNA is higher than about 75 °C and about 40 °C for double strand RNA.
As was previously mentioned, Earth’s average surface temperature at the time of the origin of life was about 80 °C, interestingly close to the denaturing temperature of intermediate length segments of DNA. With this fact in mind, it is now possible to imagine a physical mechanism, based on photon dissipation, for RNA and DNA replication once the temperature of the ocean surface dropped below their denaturing temperatures. researchers call this mechanism “Ultraviolet and Temperature Assisted Replication” (UVTAR) and consists in the following processes;
-
During the day, the absorption of UVC light by RNA and DNA and some visible light by organic matter floating on the surface of the sea, in addition to the strong absorption of infrared light by water, caused the sea surface temperature to increase above the denaturing temperature of the RNA or DNA, and thus double strand DNA would separate into two single strands. Currently, the diurnal temperature variation in the first 100 microns of the sea surface can be up to 5 °C, with maximal temperature reached during mid-afternoon. When RNA and DNA denature, there is about a 35% increase of the absorption and dissipation of the photons, known as the hyperchromic effect. Therefore, once UVC-induced denaturing starts at one end of the double strand, it tends to become auto-catalytic.
-
At night, as ocean surface temperature drops below the denaturing temperature of nucleic acid, the single strands would act as templates for the aggregation of complementary UVC activated fragments, permitting extension and leading to the formation of a new generation of double strands. As has been shown in the laboratory
[53], this could have be catalyzed by magnesium ions (Mg+2) instead of the enzyme polymerase that currently facilitates extension inside the contemporary cell.
This process is depicted in
Figure 9 and is similar to a technique commonly used in the laboratory to amplify (multiply) DNA known as the Polymerase Chain Reaction (PCR). Researchers' process, however, uses the absorption of UVC light rather than heat to denature. This mechanism of denaturing, UVTAR, is not hypothetical, researchers have shown experimentally that it occurs for DNA and researchers have measured its temperature dependence
[62].
Figure 9. Researchers' proposed mechanism for the enzyme-less reproduction of RNA and DNA assisted by the absorption and dissipation of the prevailing UVC light flux and the high temperatures of the ocean surface during the late Hadean or early Archean, which researchers refer to as “Ultraviolet and Temperature Assisted Reproduction (UVTAR) of RNA and DNA”. “Hyperchromism” refers to an increase (∼30%) in the absorption of photons at UVC wavelengths (∼260 nm) once RNA or DNA are denatured into single strands (see
Figure 4). This UVTAR mechanism includes a day/night (diurnal) warming and cooling cycle of the ocean surface due to the absorption of solar infrared light during the day (present day to night variations of surface water temperature are about 5 °C) and the heat of dissipation of the UVC photons in the nucleic acids themselves. Most denaturing of RNA and DNA would therefore occur in the afternoon when ocean surface temperatures were highest. Since the component of circular polarization of light at the ocean surface in the afternoon is opposite to that of the morning, greater denaturing in the afternoon would lead to selection of a particular chirality (see reference
[63]). Extension (i.e., using the separated single strand as a template for attracting activated nucleotides to produce the complementary strand) occurs overnight with the aid of Mg+2 ions and colder surface temperatures. Oligos which had stereochemical affinity to the 10 amino acids listed in the figure, which have photon absorption and dissipation fomenting characteristics
[61], would have had a greater chance of denaturing during daylight hours as the ocean surface temperature cooled throughout the Archean, and these, therefore, could be replicated overnight. This selection based on greater photon dissipation researchers have termed “thermodynamic selection”
[9][10][12][13]. This is an auto-catalytic replication mechanism because, (1) denatured strands absorbs more light (
Figure 4) so, once started, denaturing will increase the rate of denaturing, and (2) extension is template directed (a single strand acts as a template). The important aspect of this mechanism, however, is that replication is tied to photon dissipation, providing a thermodynamic imperative for proliferation. Taken with permission from Mejia and Michaelian
[61].
2.6. Evolution
The thermodynamic dissipation theory for the origin of life suggests that natural selection is really thermodynamic selection that favors coupled biotic-abiotic systems of greater photon dissipation (global entropy production). The physical-chemical attraction between aromatic and amphiphilic amino acids depicted in Figure 9 and their cognate DNA or RNA codons (giving rise to a stereochemical era), is an example of antenna-quencher association which foments replication of those complexes with efficient dissipation of the Archean UVC light.
It is therefore probable that the first information encoded in the RNA and DNA was that of the codons or anti-codons corresponding to these amino acids which promote dissipation. A greater photon dissipation rate would lead to a greater denaturing rate for the DNA or RNA that coded for these amino acids and thus to their greater replication rate, especially as the seas cooled throughout the Archean. Such thermodynamic selection at the level of molecular complexation leads to the evolution of ever greater dissipative systems.
2.7. The Homochirality of Life
Perhaps one of the most difficult facts to explain concerning the origin of life is the homochirality of the fundamental molecules. Molecules having an α-carbon atom come in two versions identical in all respects except for their chiral symmetry, which affects the strength of their absorption of circularly polarized light (Figure 10). For example, all DNA and RNA from living organisms absorb preferentially right-hand circular polarized light, while the amino acids absorb preferentially left-hand circular polarized light. Researchers say then that the DNA and RNA of living beings are right-handed and the amino acids are left-handed. Both versions of chirality of the molecule are produced equally in abiotic experiments, but only one chirality is found in Nature.
Figure 10. The two distinct chiral versions (left- and right-handed) of a given amino acid with functional group R. The isomers have identical Gibb’s free energies so both have equal formation and degradation probability under equilibrium conditions. However, left-handed amino acids, and right handed nucleic acids and ribose sugars, predominate in biology and this fact must be explained by any theory purporting to explain the origin of life. Image credit; NASA, Public Domain.
Theories developed to explain homochirality have relied on either an initial preponderance of one chirality, e.g., through a selective destruction of the other chirality, for example from the particular circular polarization of the high energy light that comes from one hemisphere of a pulsar, or the chance formation of the original fundamental molecules on chiral inorganic substrates, or some kind of a asymmetric mechanism for the amplification of an initially very small chirality asymmetry. However, no theory has, so far, convincingly explained how chirality was incorporated into living systems. This homochirality must have occurred very early in the evolution of life since incorporation of the wrong chirality nucleotide into a DNA or RNA single strand frustrates the extension of the complementary strand, thereby halting reproduction.
Researchers' theory offers a simple explanation of homochirality through the UVTAR mechanism for denaturing and reproduction of the nucleic acids (see
Figure 12) based on the fact that there is an important component of circularly polarized light at the ocean surface which is of one circular polarization in the morning and of the opposite in the afternoon. The ocean surface is, in fact, the region where one can find the greatest circular polarization of light on Earth
[63]. The UVTAR mechanism for denaturing is strongly temperature dependent, being more effective at higher temperatures
[62]. In the afternoon, the Archean sea surface would have been about 3–5 °C warmer and thus greater denaturing of RNA or DNA would occur in the afternoon compared to the morning, therefore leading to the preferential denaturing and subsequent replication of one chirality. A few thousand years of such an asymmetric replication process would be sufficient to arrive at effectively 100% single chirality (homochirality) for the nucleic acids
[63].
The stereochemical affinity of amino acids for nucleic acid is greater for opposite chiralities, for example, left-handed amino acids have greater affinity to right-handed RNA or DNA, and vice-versa. The circular dichroism, which measures the difference between the absorption of right- and left-handed circularly polarized light, increases for the complex of a right-handed DNA with a left-handed amino acid
[63]. These facts could be sufficient to explain the initial selection of left-handed chirality of the amino acids.
2.8. Conclusions
Dissipative structuring under light, as the fundamental creative force in biology, appears to have been ongoing, from the initial dissipation at the UVC wavelengths during the Archean by the fundamental molecules of life, to the dissipation of wavelengths up to the red edge (∼700 nm) by the organic pigments of today
[9][10][11]. Beyond the red edge, starting at about 1200 nm, water in the ocean surface microlayer absorbs strongly and dissipates photons into heat efficiently. There is, therefore, still a wavelength region between 700 and 1200 nm which remains to be conquered by future evolution of pigments. The simultaneous coupling of biotic with abiotic irreversible processes, such as the water cycle and ocean and air currents, culminating in an efficient global dissipating system known as the biosphere, increases further the efficacy of solar photon dissipation into the far infrared much beyond 1200 nm
[9][16][17].
Empirical evidence for selection in nature towards states of increased dissipation exists on vastly different size and time scales. For example, the increase in photon absorption and dissipation efficacy of a plant leaf over its life-cycle
[64], the proliferation of photon absorbing pigments over the entire surface of Earth, the correlation between ecosystem succession and increased dissipation
[65][66], and the general increase in biosphere efficacy in photon dissipation over evolutionary history, including, for example, the plant-induced increases in the water cycle
[17][67] and animal dispersal of nutrients required for pigment synthesis
[12]. There is also evidence for this at the microscopic scale, for example in the increased rates of energy dissipation per unit biomass of the living cell over its evolutionary history
[68].
The author hopes that the reader has been convinced that what has been presented are the non-equilibrium foundations of the origin of life and encourages the reader to delve into more detail of each process by referring to the cited literature.