8-prenylnaringenin (8-PN) is a prenylated flavonoid, occurring, in particular, in hop, but also in other plants. It has proven to be one of the most potent phytoestrogens in vitro known to date, and in the past 20 years, research has unveiled new effects triggered by it in biological systems. These findings have aroused the hopes, expectations, and enthusiasm of a “wonder-drug” for a host of human diseases. However, the majority of 8-PN effects require such high concentrations that they cannot be reached by normal dietary exposure, only pharmacologically; thus, adverse impacts may also emerge.
1. Introduction
Hop (
Humulus lupulus L.) has been used as a preservative and flavoring agent in beer for centuries. This plant is rich in phenolic acids, flavonoids, proanthocyanidins, prenylated chalcones, and flavanones, as well as catechins with potential therapeutic applications
[1]. Historically, menstrual disturbances were commonly reported among female hop pickers
[2], and hop extract was reported to be efficient in reducing menopause-associated hot flushes in women. Moreover, hop baths have been used for treatment of gynecological disorders over the years
[3]. Therefore, a recurring suggestion was that hop may contain compounds with powerful estrogenic activities. The estrogenicity of hops was attributed to xanthohumol (XN) without any reported scientific data
[2]. Preliminary studies on estrogenic potency of hop showed contradictory results: some studies revealed high estrogenic activity
[4][5], while others found no or low estrogenic activity of hop
[6]. These discrepancies might have been due to the varieties of extracts and the nature of assays used
[5]. In 1999, Milligan et al. reported of a novel compound, 8-prenylnaringenin (8-PN), after a successful bioassay-guided fractionation of hop extracts, and provided the first evidence of its prominent estrogenic activity
[7]. In fact, this compound had been originally identified from hops as early as 1984
[8] but had been forgotten thereafter. Since Milligan et al.’s discovery, numerous studies have substantiated the high estrogenic potency of 8-PN. With accumulating evidence on the wide exposure of 8-PN to the general population, and of its exceptionally high potency in vitro, concerns have also arisen regarding the possible adverse effects to humans.
2. Occurrence, Sources of, and Exposure to 8-PN
Throughout the years, a wide variety of methods, predominantly mass spectrometry-based, have been developed for reliable quantitative determination of 8-PN and related prenylated flavonoids. They have enabled the analysis of these substances in diverse matrices and revealed that the main source of human 8-PN exposure was beer drinking when hop was used during the brewing process
[9]. Hops are added to beer in the form of dried hop or lipophilic extracts
[10]. Hop extracts or dried hops are obtained from female hop cons that are particularly rich in prenylated chalcones (XN, desmethyl-XN) and prenylated flavanones (6-PN, 8-PN)
[11]. The exact amount of each ingredient varies, because chalcones undergo isomerization during the brewing process, which results in the conversion of XN to isoxanthohumol (IX) and of desmethyl-XN to a racemic mixture 6- and 8-PNs (
Figure 1). Moreover, the cyclization of XN to IX may generate two enantiomers of IX, which can lead to two enantiomers of 8-PN
[12]. The concentration of XN may amount to 1% while 8-PN is more than 10 times less abundant
[13].
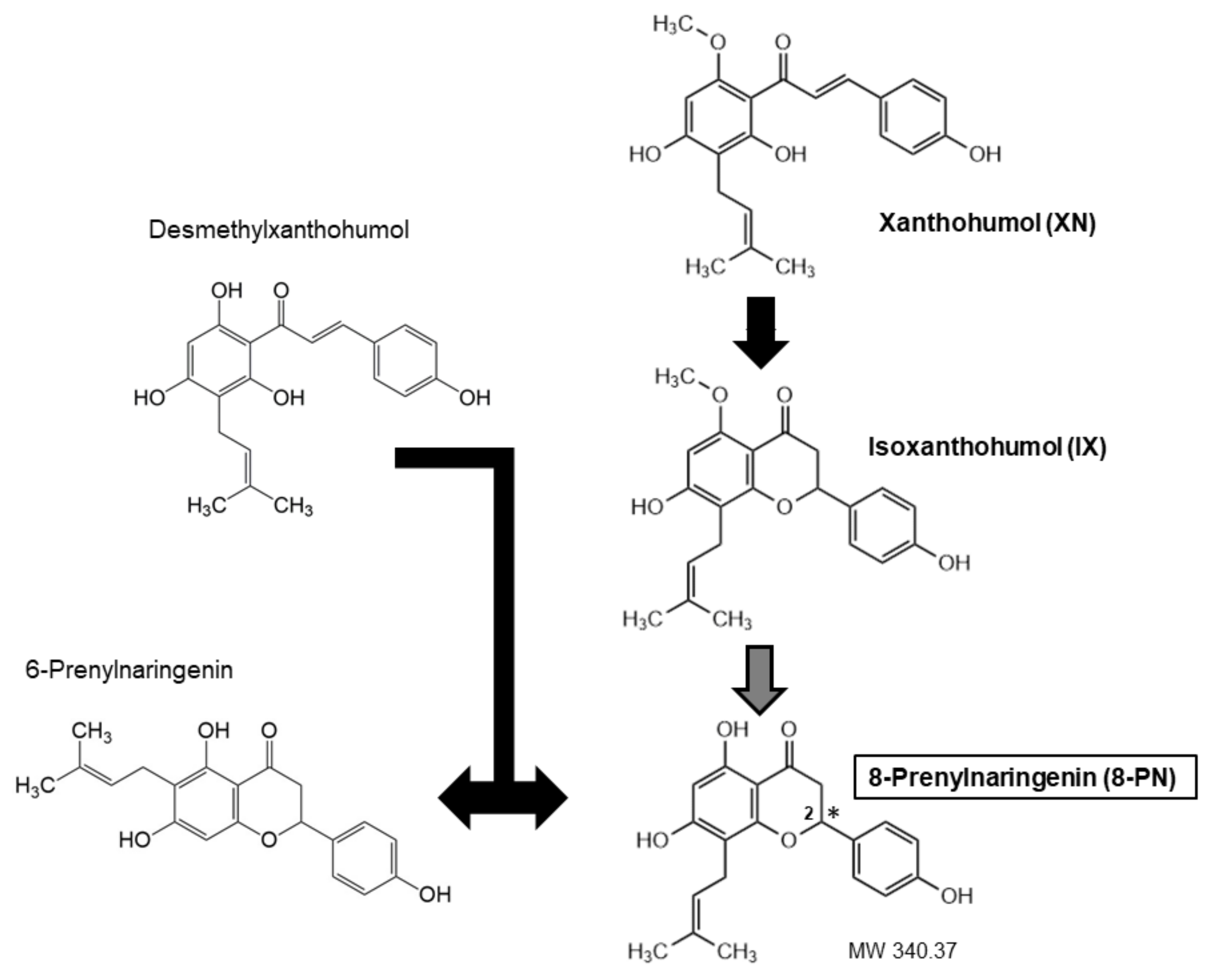
3. Methods Applied in 8-PN Identification and Quantification
The bulk of the methods described to date for 8-PN quantification have combined LC or HPLC separation with detection by mass spectrometry (MS), although a gas chromatography (GC)–MS method has also been published
[14][15][16][17][18][19][20]. The varieties of MS used include electron impact (EI), atmospheric pressure electrospray ionization (ESI), or atmospheric pressure chemical ionization (APCI) in either positive or negative ion modes
[14][21][22][23]. Apart from MS, HPLC methods with ultraviolet (UV) or UV/diode array detector (DAD) detection have been developed
[21][24][25][26]. Some modifications of these techniques include ultra-high-pressure LC–tandem MS (UHPLC–MS/MS), ultra-high-performance supercritical fluid chromatography (UHPSFC; with either UV or MS detection), and the application of secondary standards in HPLC–UV/DAD
[27][24]. Antibody-based methods have also found their way to 8-PN quantification. Polyclonal antibodies were used for the design of a radioimmunoassay (RIA), and monoclonal for devising an enzyme-linked immunosorbent assay (ELISA)
[28][29]. The most recent new technique described for 8-PN quantification is stable isotope dilution analysis (SIDA)
[30].
4. Pharmacokinetics of 8-PN
Studies with pure 8-PN on other aspects of its pharmacokinetics than biotransformation are scarce. After the ingestion of a single oral dose of 500 mg racemic 8-PN in 16 healthy young adults (8 women, 8 men), a maximum plasma concentration was attained at 1.6 h with large inter-individual (but not gender-related) differences in the height of the peak
[31]. Interestingly, at an equimolar dose, 6-PN peaked later (2.3 h) and its area under the plasma concentration–time curve remained much smaller. When three groups of eight healthy postmenopausal women were treated orally with 50, 250, or 750 mg racemic 8-PN, the compound was rapidly absorbed, leading to maximum serum drug concentrations 1.0–1.5 h after administration
[32]. Thereafter, drug serum concentrations decreased sharply, followed by a further increase in concentrations leading to a second peak, which occurred at 7–10 h. This was suggestive of marked enterohepatic recirculation, probably attributable to the prenyl group in 8-PN
[31].
In agreement with these in vivo reports attesting to effective gastrointestinal (GI) absorption of 8-PN, in monolayers of the human intestinal epithelial cancer cell line, (Caco-2), 8-PN also exhibited good absorption, probably via passive diffusion
[33].
5. Estrogenic Activity of 8-PN
5.1. In Vitro Studies
8-PN is one of the most potent phytoestrogens currently known. This has been confirmed by a variety of in vitro assays for estrogenic activity, including yeast-based screens expressing the human estrogen receptor (ER) and a reporter gene (usually luciferase, β-galactosidase or chloramphenicol acetyltransferase) under the control of estrogen-responsive sequences (ERE)
[5][34][35][36], human cell lines responsive to estrogenic stimulation
[5][7][37][38], and ER binding
[5][7][38][39]. Compared with established phytoestrogens, such as coumestrol, genistein, and daidzein, the estrogenic activity of 8-PN has generally proven to be 0.6–150, 8–150, and 50–1500-fold stronger, respectively
[5][7][36][39] (but see ERβ-specific data below), while concomitantly 5–250 times weaker than that of 17β-estradiol (E2)
[5][7][34][36][37][38][39][40][41]. The estrogenic activities of IX and 6-PN have been reported to be ~1% and <1% relative to 8-PN, respectively, while XN appears to be devoid of estrogenic properties
[7]. Likewise, the parent compound of 8-PN, naringenin, shows only very weak estrogenicity
[42][43].
5.2. In Vivo Studies
In in vivo conditions, the estrogenicity of 8-PN may be less prominent than in vitro. In juvenile male medaka fish, the positive control compound ethinylestradiol at the concentration of 0.34 nM induced sex reversal as indicated by the expression of the ovary-specific gene
figla and the estrogen-dependent gene
vitellogenin. However, 674 nM 8-PN (i.e., ~2000-fold concentration) failed to cause either of these effects
[41]. When assessed by uterine vascular responses in ovariectomized (OVX) mice after subcutaneous (sc.) administration, the estrogenic activity of 8-PN was only <1% compared with E2. It was even lower (<0.1%) based on mitotic activity of vaginal epithelia upon peroral treatment. Moreover, in contrast to E2, 8-PN failed to induce mitosis in uterine epithelium or increase uterus weight in OVX mice at oral doses of up to 16 mg/kg/day for 3 days.
[5]. This may be a species-dependent failure, because in OVX Wistar rats treated for 3 days with 10 mg/kg/day of either 8-PN or genistein, 8-PN proved to cause a greater elevation in uterus weight (~2 times the control weight) than genistein (~1.4 times the control weight)
[44].
6. Endocrine Roles of Estrogen and 8-PN
Secondary metabolites from plants, including 8-PN, have been considered as alternatives to the classic hormone therapy in women. Studies on the endocrine properties of 8-PN have demonstrated that this compound is a natural selective estrogen receptor modulator (SERM) because its effect spectrum is not fully identical with that of E2. As already referred to earlier, E2 induces cellular changes through nuclear and non-nuclear mechanisms, and ER exists in 2 forms, ERα and ERβ, which have multiple isoforms and exhibit distinct tissue expression patterns and functions
[45][46]. The classical nuclear mechanism of ER’s action typically occurs within hours, leading to activation or repression of target genes. However, estrogens can also induce rapid signals that act within seconds or minutes through extranuclear, membrane-associated forms of ERs as well as a G protein-coupled estrogen receptor (GPCR1; also called GPCR30)
[47].
6.1. ERα Tissue Expression
ERα is mainly expressed in endometrium, ovarian stroma, bone, mammary gland, placenta, pancreas, skeletal muscle, white and brown adipose tissues, and various neuroendocrine areas of the brain
[48][49][50]. Strikingly, ERα is abundantly present in the ventrolateral portion of the ventromedial nucleus, the arcuate nucleus and the paraventricular nuclei of the hypothalamus as well as the medial preoptic area, whereas ERβ is significantly less expressed in these locations
[51][52][53][54][55][56].
6.2. Energy Metabolism
6.2.1. Effects of 8-PN and Related Compounds on Energy Balance
8-PN treatment in drinking water at a dose of 10 mg/L/day for 20 weeks was reported to lead to inhibited body weight gain in OVX mice when compared with the control OVX group, an effect similar to that observed in estrogen-treated OVX mice. The lower body weight gain was accompanied by food intake reduction in 8-PN-treated OXV mice
[57][58].
6.2.2. Effects of 8-PN and Related Compounds on Lipid Metabolism
Provided in drinking water, 8-PN at the dose of 10 mg/L/day for 20 weeks ameliorated plasma lipid profile in a high-fat diet-induced type 2 diabetes mouse model. The changes triggered included reduced total cholesterol and triglyceride levels and enhanced HDL concentration and HDL/LDL ratio in plasma. In the same study, 8-PN activated AMPK signaling, thereby inhibiting SREBP-1c protein expression and its downstream lipogenic enzyme targets FAS and ACC
[57].
6.2.3. Effects of 8-PN and Related Compounds on Glucose Homeostasis and Insulin Sensitivity
Similar to E2, 8-PN has also been reported to exert an antidiabetic effect. It lowered plasma glucose levels and improved glucose handling in oral glucose and insulin tolerance tests in a type 2 diabetes mouse model. It was further shown to augment the protein abundance of the insulin-regulated signaling molecule AS160 in skeletal muscle, suggesting improvement of glucose uptake by this tissue
[58]. Insulin stimulates the PI3K/AKT signaling cascade which activates AS160 downstream, triggering GLUT4 translocation to cell membrane
[59].
6.3. Pituitary Function
6.3.1. Effects on LH and FSH Secretion
Similar to E2 at a concentration of 10
−9 M, 8-PN at a 1000-fold higher concentration directly suppressed LH release in a primary culture of rat pituitary cells. This response was significantly antagonized by the pure ER antagonist ICI 182,780 (Fulvestrant, Faslodex)
[57].
6.3.2. Effects on Other Pituitary Hormones
E2 or 8-PN at concentrations of 10
−9 and 10
−6 M, respectively, directly induced TSH release by a primary culture of rat pituitary cells
[57]. This response was significantly antagonized by the ER antagonist ICI 182,780. In the in vivo part, a single oral dose of E2 (15.5 mg/kg BW) or 8-PN (161.4 mg/kg BW) elevated the circulating TSH level in OVX rats.
7. Regulation of Bone Homeostasis
One of the common consequences of estrogen decline in menopause is loss of bone mass that may lead to osteoporosis. Similar to E2, 8-PN and naringenin were reported to promote osteogenic differentiation in vitro
[60]. In order to gain further insight into the importance of the prenyl group in 8-PN for its antiosteoporotic effects, 8-PN and naringenin were compared in in vitro conditions
[61]. 8-PN was found to have a stronger ability than naringenin to improve osteoblast differentiation and osteogenic function in cultured rat calvarial osteoblasts. Concomitantly, 8-PN was more effective in inhibiting osteoclastogenesis, inducing osteoclast apoptosis and reducing the resorptive activity of osteoclasts in rabbit bone marrow cells, thus confirming the importance of the prenyl group in the naringenin structure for the bone protective mechanism. In another in vitro study, 8-PN closely mimicked E2 by enhancing osteoblast activity in the MC3T3-E1 osteoblast cell line and inhibiting osteoclastic differentiation from the multinuclear macrophage RAW264.7
[62]. The effects of 8-PN could be largely abolished by the selective ERα antagonist MPP but not with the selective ERβ antagonist PHTPP. The magnitude of 8-PN effects was smaller than that of a 1000-fold lower concentration of E2, but larger compared with equimolar concentrations of genistein and daidzein.
8. Effects on Tumor Cells In Vitro
A variety of in vitro studies have probed the impact of 8-PN and related hop flavonoids on cancer cell invasion, proliferation and apoptosis. In the majority of these studies, 8-PN along with its precursors and other naringenin derivates displayed growth inhibitory and apoptotic effects in various cancer cell lines. For example, Delmulle et al. (2006) exposed the human prostate cancer cell lines PC-3 and DU145 to 8-PN, 6-PN, XN, IX and desmethyl-XN for 2 days. They found inhibited growth of the cells, with the following order of compound potency (IC
50 [µM] for DU145 and PC-3, respectively): XN (12.3, 13.2) > 6-PN (29.1, 18.4) > 8-PN (43.1, 33.5) > IX (47.4, 45.2) > desmethyl-XN (53.8, 49.9)
[63]. In these cell lines, 8-PN, IX and 6-PN were shown to induce a caspase-independent form of cell death, suggested to be autophagy
[64]. 8-PN and 6-PN also dose-dependently (6.25–100 µM) reduced proliferation of PC-3 and UO.31 renal carcinoma cells
[65]. In the human leukemic T lymphocyte cell line Jurkat, the antiproliferative and proapoptotic effects of 8-PN were associated with inhibition of voltage-gated Kv1.3 potassium channels
[66].
9. Other Beneficial and Adverse Effects of 8-PN
9.1. Cytotoxicity to Somatic Primary Cells
Regarding human somatic primary cells, no decrease in cell viability was detected in human umbilical vein endothelial cells (HUVEC) or human aortic smooth muscle cells (HASMC) at any 8-PN concentration tested (highest 20 µM). In fact, the converse was true of HUVEC, in which 20 µM 8-PN caused a significant increase in viable cell number. Meanwhile, 10 and 20 µM concentrations of XN and IX led to reduced viability of HUVEC and/or HASMC
[67]. In keeping with these findings, apoptosis was dose-dependently inhibited by 8-PN but promoted by XN and IX in both cell lines. On the other hand, cell proliferation displayed opposite effects only in HUVEC (increased by 8-PN, decreased by XN and IX).
9.2. Effects on Gonadal Cells
Similarly to E2, 8-PN significantly stimulated capacitation and the acrosome reaction in incapacitated epididymal mouse spermatozoa, compared with untreated controls. Unexpectedly, however, 8-PN proved to be some 1000 times more potent than E2, being active already at low nM concentrations. The SERM compound hydroxytamoxifen failed to interfere with these actions. In capacitated sperm, E2 had no discernible effect whereas 8-PN was again able to induce the acrosome reaction. Both E2 and 8-PN enhanced sperm fertilizing ability in vitro
[68]. Another phytoestrogen, genistein, exhibited the same effect pattern and potency, and low concentrations of genistein and 8-PN were more effective in combination than individually to accelerate capacitation
[69]. Moreover, sensitivity to genistein was even higher for human than mouse sperm (sensitivities to 8-PN were not tested).
9.3. Effects on Aromatase in Other Cell Types
Aromatase is a major enzyme in the biosynthesis of steroids, catalyzing a critical step for estrogen production from circulating androgens. It is expressed not only in the gonads but also in adipose tissue, vasculature, bone, brain, placenta, fetal liver, and estrogen-dependent cancers
[70]. In choriocarcinoma-derived JAR cells, which express high levels of aromatase, 8-PN proved to be a highly potent inhibitor of its activity. The IC
50 was as low as 65 nM, while for XN and IX it was 20 and 140 µM, respectively. However, no effect was recorded on aromatase gene expression (
CYP19)
[71].
9.4. Effects on Other Enzymes of Clinical Relevance
AKR1B1 or human aldose reductase mediates the first step in the reduction of glucose to sorbitol in the polyol pathway, which under hyperglycemic conditions is co-responsible for the diabetic complications (i.e., retinopathy, neuropathy, nephropathy, cataract)
[72]. Consequently, aldose reductase inhibitors are at the focus of research aiming at the prevention of these complications. The AKR1B1 homologue AKR1B10, in turn, is a NADPH-dependent oxidoreductase that converts carbonyl group containing compounds to their corresponding alcohols. It is overexpressed in several cancers and precancerous lesions, thus possibly playing a crucial role in the development of cancer
[73].
9.5. Effects on Blood Vessels
8-PN inhibited growth factor-induced angiogenesis of bovine endothelial cells in vitro, with an IC
50 between 3 and 10 µM. In the chicken early chorioallantois membrane, it reduced vessel diameter and tended to shorten vessel length. In both assay types, 8-PN was roughly equipotent to genistein
[74].
10. Conclusions
8-PN is a potent phytoestrogen with a multitude of target organs and tissues. It also influences a wide variety of cellular signaling pathways, both ER-dependent and ER-independent. While these effects have been repeatedly and convincingly established in vitro, the in vivo data are much more meagre. Furthermore, in most cases, the effects have required such high concentrations that they cannot be reached physiologically from dietary exposure to 8-PN and its precursors. The inter-individual differences in intestinal and hepatic generation of 8-PN from IX are yet noteworthy if hop-derived dietary supplements are used.
The most urgent information needs currently include the health consequences of chronic exposures to high doses of 8-PN (heavy beer drinkers with high IX conversion capability) and—closely related to this—its effects in men. Since hops have mainly found therapeutic use in the treatment of menopausal health problems in women, the great preponderance of human studies on 8-PN have understandably confined to female subjects. However, given that 8-PN has endocrine disrupting potency in males, beer is more commonly consumed by men than women, and that sperm has proven an exceptionally sensitive target to 8-PN, men should no longer be neglected in 8-PN studies.