Colorectal cancer (CRC) is the third leading cause of death in men and the fourth in women worldwide and is characterized by deranged cellular energetics. Thymoquinone, an active component from Nigella sativa, has been extensively studied against cancer, however, its role in affecting deregulated cancer metabolism is largely unknown. Further, the phosphoinositide 3-kinase (PI3K) pathway is one of the most activated pathways in cancer and its activation is central to most deregulated metabolic pathways for supporting the anabolic needs of growing cancer cells.
1. Introduction
Tumor cells are supported by metabolic rewiring in their growth, proliferation, survival, maintenance and reprogram the pathways of nutrient acquisition to meet the bioenergetics
[1]. This metabolic reprogramming is known as one of the prominent hallmarks of almost all cancers
[2]. The hypothesis that cancer cells uptake glucose and produce a significant amount of lactate in the presence of normoxia due to weak mitochondrial function led to the broadly held delusion that cancer cells follow glycolysis as their major source of ATP
[3]. Many tumor cells reside in nutrient and oxygen-deficient environments, which makes a heterogeneous build-up that led cancer cells to adopt several methods to sustain mitochondrial function for survival
[4]. Metabolism is improved in differentiated non-dividing cells to supply ATP through OXPHOS. Cells that proliferate at faster rates whether tumor cells or normal embryonic, immune and regenerating cells need ATP as well as anabolic constructive material for biomass improvement and nutrient refilling
[5][6]. A glycolytic fermentative constitution similar to tumor cells is displayed by highly proliferative normal cells as well; a major division in cancer cells is the loss of the regulatory response kink
[7]. This exclusive glucose-dependent phenotype together with enhanced lactate tumors serving as potential biomarkers of poor patient survival has motivated us along with other scientists
[8][9][10][11] to disengage glycolysis in cancers as a presumed therapeutic move.
In human cancers, the most commonly activated pathway is said to be the phosphoinositide 3-kinase PI3K-AKT pathway
[12]. This pathway renovated cellular metabolism in cancer cells by increasing the action of nutrient transporters and metabolic enzymes by oncogenic activation following the anabolic demands of abnormally growing cells. It was reported that the PI3K pathway controls the reprogramming of hexokinases (HKs), the enzymes which play a potent role in the conversion of glucose into glucose-6-phosphate (a major rate-limiting stage of glycolysis) and other metabolic pathways which lead to the up-regulation of HK II expression
[13][14].
In glucose metabolism, the catalysis loyalty is started by Hexokinases (HKs). Glucose transporters (GLUTs) transport glucose via the plasma membrane and are phosphorylated by Hexokinases to produce glucose-6-phosphate
[15]. In humans, four isoforms of Hexokinases were categorized which are HK: I, II, III and IV. Being structurally similar, the four isoforms vary in expression pattern, cellular localization and properties in regulation. The dominantly expressed among these is HK II which is found to be up-regulated in most of the tissues
[16]. Hexokinase II was found to be over-expressed in most cancers leading to enhanced glycolytic rate, which is a phenotype for cancer cells
[17]. Many studies have suggested HK II as a therapeutic target for treating cancer
[18][19]. Therefore, it is important to evaluate compounds/drugs that could be effective in repressing HK II and hence could inhibit tumor metabolism.
Thymoquinone (TQ), also known as black seed or black cumin, is a key bioactive phytochemical constituent of Nigella sativa and it plays a major role in providing health benefits via anti-oxidant, anti-microbial, anti-diabetic, anti-inflammatory effects, metabolic syndrome disorders and has a long history of medicinal use in traditional medicinal practices
[20][21][22][23]. Many studies have established TQ as a potential anti-cancer agent by inhibiting proliferation, migration, invasion and angiogenesis in a variety of cancer cells
[24]. Thymoquinone targets cancer signaling molecules and pathways through different axes in various cancers. In prostate cancer thymoquinone targets via the down-regulation of the androgen receptor (AR) and the proliferation regulator E2F-1
[25]; the (STAT3) pathway in human multiple myeloma cells
[26]; JAK2 and c-Src in gastric cancer cells
[27]; Bax up-regulation, Bcl-2 inhibition and activation of caspases in colorectal cancer
[28]; inhibiting p38 MAPK in oral cancer
[29]; activating p73 and UHRF1-dependent mitochondrial and cell cycle checkpoints in acute lymphoblastic leukemia
[30][31], and the inhibition of the Notch signaling pathway in hepatic carcinoma
[32]. On the other hand, the effect of thymoquinone on cancer metabolism, an emerging hallmark of cancer, is unclear.
2. Thymoquinone Induces Cell Death in CRC Cells
Their first aim was to study the effect of thymoquinone on the growth inhibition against two human colorectal cancer cells lines, HCT116 and SW480. It was observed that treating the cells with increasing concentrations of thymoquinone (0–100 µM) led to a dose and/or time-dependent reduction in cell viability (
Figure 1A). The corresponding IC
50 values for HCT116 and SW480 at 24 h (post-treatment) were 21.71 µM and 20.53 µM, and those at 48 h (post-treatment) were 10.26 µM and 10.50 µM, respectively, (
Figure 1A,B). The lowest:highest percent death induction in HCT116 and SW480 at 24 h (post-treatment) was 20.35 ± 4.01; 74.95 ± 5.39 and 17.71 ± 3.87; 76.67 ± 4.16 while at 48 h (post-treatment) it was 44.87 ± 4.38; 88.30 ± 6.26 and 48.04 ± 4.47; 87.13 ± 6.18, respectively. Researchers also evaluated cell death over a time period of 1–4 days by employing a trypan blue dye exclusion assay; the results were consistent with MTT results showing more cell death/inhibition in cell doublings per day with the increase in drug concentration (
Figure 1B). Researchers did not evaluate TQ’s biocompatibility on normal intestinal cells because the same was previously reported to be well tolerated by normal intestinal cells for concentrations of up to 60 µM
[33].
Figure 1. Thymoquinone induces cell death and hampers cell proliferation in CRC cells. HCT116 and SW480 CRC cells were treated with thymoquinone as indicated. (A) Thymoquinone induced cell death in a dose and time-dependent manner, statistical significance was calculated by two-way ANOVA, considering both time and concentration-dependent observations. (B) Thymoquinone treatment-induced cell death/inhibited cell doublings per day over a time period of 1–4 days. (C) Pre-treatment of thymoquinone to HCT116 (21.71 µM) and SW480 (20.53 µM) led to a significant reduction in cell proliferation over time (0–4 days), statistical significance was calculated by employing two way ANOVA following appropriate post hoc test (Bonferroni test). The results are expressed mean ± SEM (n = 3 or 4). ** p < 0.01, *** p < 0.001.
Since uncontrolled cell proliferation is a well-established cancer hallmark
[2], researchers next attempted to evaluate whether sub-lethal dose/s of TQ have any modulatory effect on the proliferation index of CRC cells. It was observed that pre-treatment of TQ in both HCT116 (21.71 µM) as well as SW480 (20.53 µM) cells for 24 h led to a significant reduction in proliferation rate over time (0–4 days) (
Figure 1C), highlighting its potential anti-proliferative role against CRC.
Deregulated cellular metabolism is a characteristic feature of most, if not all, cancerous cells and an emerging cancer hallmark
[34][35], which expedites the cell-autonomous proliferation and survival of these cells
[36]. The Warburg effect manifests an aberrant metabolic state in which cancer cells ferment glucose at higher rates to sustain the higher energy needs as well as biomass production, and hence ensure increased proliferation
[5][37]. To this end, researchers speculated on studying whether cell death induced by TQ in CRC cells (HCT116 and SW480) could be attributed to the inhibition of the glycolytic phenotype in these cells. Researchers found that sub-lethal doses of TQ in both HCT116 (21.71 µM) and SW480 (20.53 µM) after 24 h of treatment significantly reduced the glucose fermentation potential of these cells (
Figure 2A). Additionally, such reduction in glucose fermentation capacity also led to a significant reduction in overall ATP production as well as maintaining the redox state (NADPH production) of these cells (
Figure 2B,C). These results demonstrate that TQ-induced cell death and/or proliferation inhibition in CRC could be attributed to modulation of the glucose metabolic reprogramming, otherwise necessary to ensure increased biomass production and maintaining redox balance for sustaining proliferative signaling.
Figure 2. Thymoquinone reduces glycolytic metabolism in CRC. HCT116 and SW480 CRC cells were treated with thymoquinone as indicated. Thymoquinone treatment led to a significant reduction in—(A) glucose fermentation rate/s (glucose production and/or lactate production), (B) ATP production, and (C) redox state (NADPH) in these cells. Statistical significance was calculated by employing unpaired t-test, data expressed as mean ± SEM (n = 3). * p < 0.05 and ** p < 0.01.
4. Thymoquinone Inhibits Hexokinase 2 via Modulating the PI3K-AKT Pathway
To underpin the role behind the reduction in the glucose fermentation rate in TQ-treated CRC cells, researchers evaluated the expression status of HK2, the first rate-limiting enzyme of glucose metabolism and an enzyme highly overexpressed in most cancers including CRC
[38][39][40][41]. It was observed that TQ treatment substantially inhibited HK2 expression in HCT116 and SW480 cells (
Figure 3A). Further, to know the mechanism behind the HK2 reduction, researchers evaluated the PI3K-AKT levels because previously it was reported that PI3K-AKT induces glycolytic phenotype via HK2 in pediatric osteosarcomas
[13]. Expectedly, the reduction in HK2 levels upon TQ treatment coincided with significant inhibition in PI3K-AKT activation (i.e., reduction in p-PI3K-p-AKT
T308/S473) (
Figure 3B), suggesting that TQ inhibits HK2 via modulating the PI3K-AKT pathway. To confirm whether such reduction in HK2 was PI3K-AKT-dependent, researchers used the selective pharmacologic inhibitor (LY294002) of PI3K and researchers observed a significant reduction in HK2 levels concomitant to PI3K-AKT inhibition (
Figure 3C). These results demonstrate that TQ inhibits glycolytic metabolism in CRC cells by inhibiting HK2 via the PI3K-AKT pathway.
Figure 3. Thymoquinone inhibits Hexokinase-2 via modulating the PI3K-AKT pathway. HCT116 and SW480 CRC cells were treated with thymoquinone or LY294002 as indicated. (A) Thymoquinone treatment led to inhibition in HK2 protein levels. Such inhibition in HK2 was concomitant to inhibition in—(B) the PI3K-AKT pathway. (C) Inhibiting the PI3K-AKT pathway using selective PI3K pharmacologic inhibitor LY294002 led to an inhibition of HK2 protein levels.
5. Inhibition of the PI3K-AKT/ HK2 Pathway Abrogates the Tumorigenic Potential of CRC Cells
To seek whether targeting the PI3K-AKT/HK2 pathway also abrogates the tumorigenic propensity of CRC cells, researchers used a selective pharmacologic inhibitor of PI3K (LY294002) to study colony formation and cell proliferation over time. It was found that PI3K inhibition significantly inhibited colony formation potential as well as proliferation over time (Figure 4A,B); further confirming that the carcinogenic propensity of CRC cells (HCT116 and SW480) is majorly regulated via the PI3K-AKT axis. More importantly, genetic (siRNA) and low-dose pharmacologic (10 µM, 3-Bromopyruvate) ablation of HK2 led to a significant reduction in cell proliferation as well as colony formation propensity in these cells (Figure 4C,D). Additionally, pharmacologic silencing of PI3K also led to a significant rescue in glycolytic reprogramming (Figure 4E–G), similarly, genetic ablation (siRNA-mediated) of HK2 also showed a significant reduction in glucose fermentation, ATP and NADPH production rates (Figure 4H–J). These results demonstrate that PI3K-AKT/HK2 activity directly regulates the tumorigenic propensity of CRC cells, which can be effectively targeted by TQ.
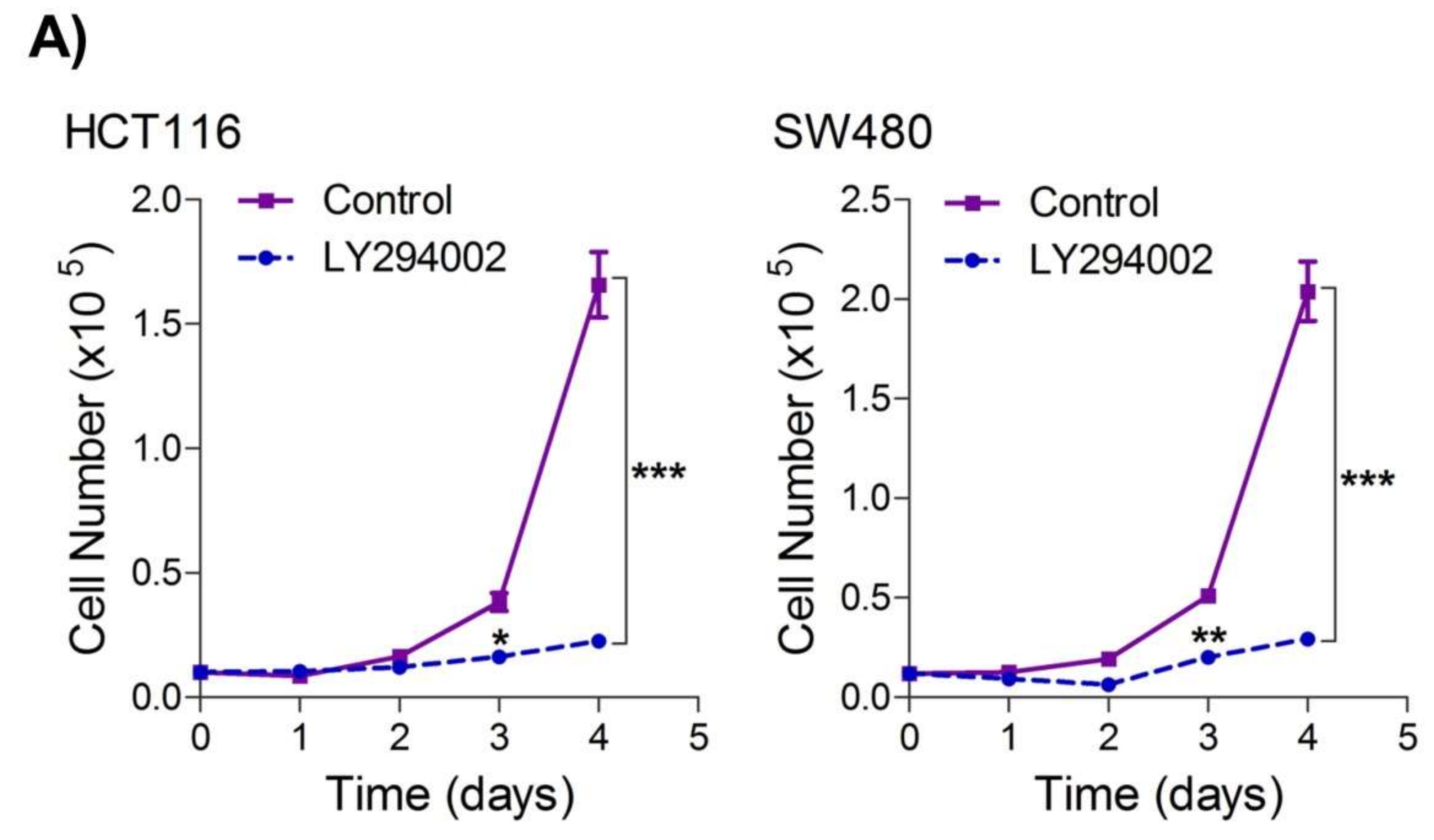
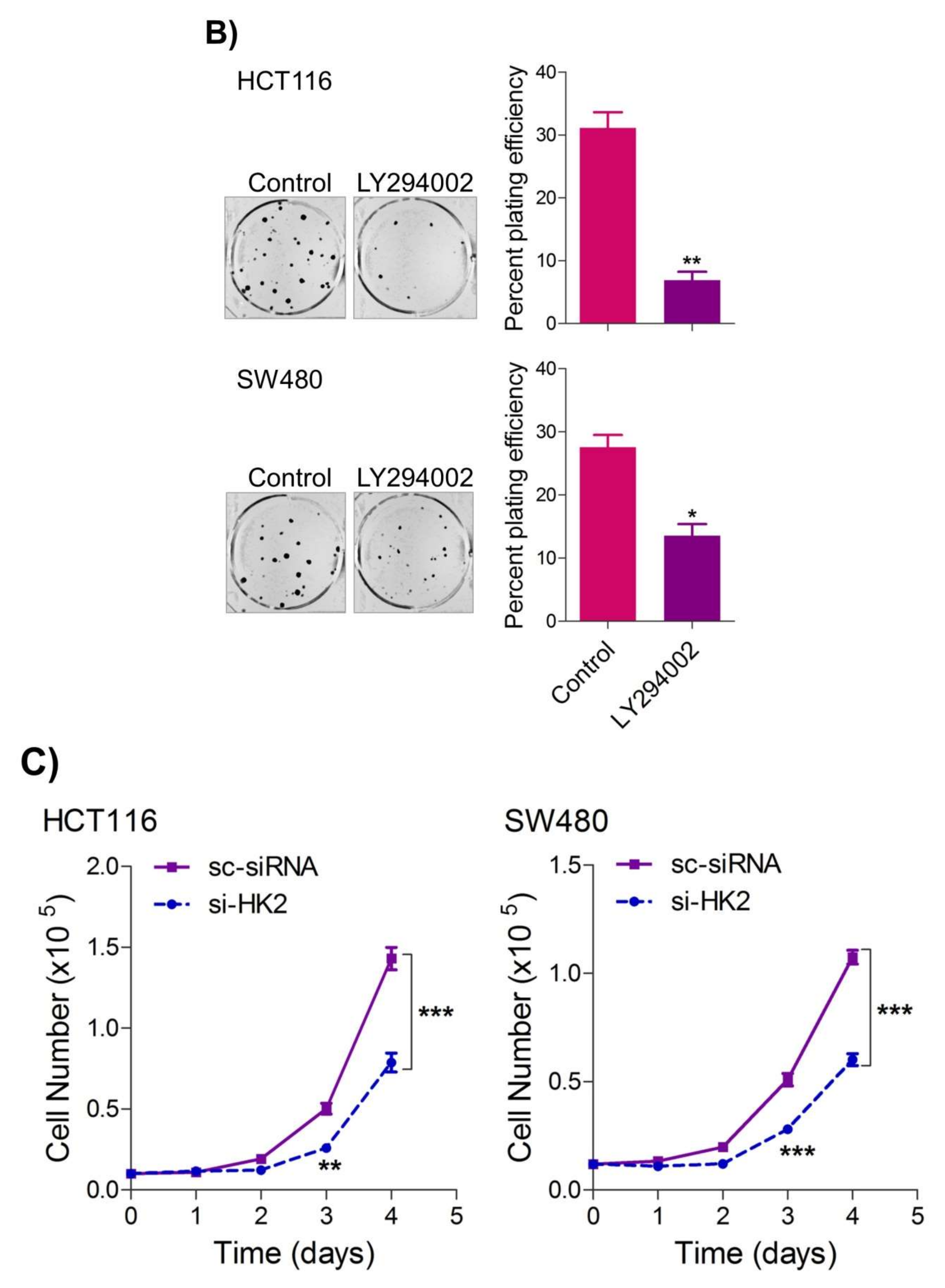
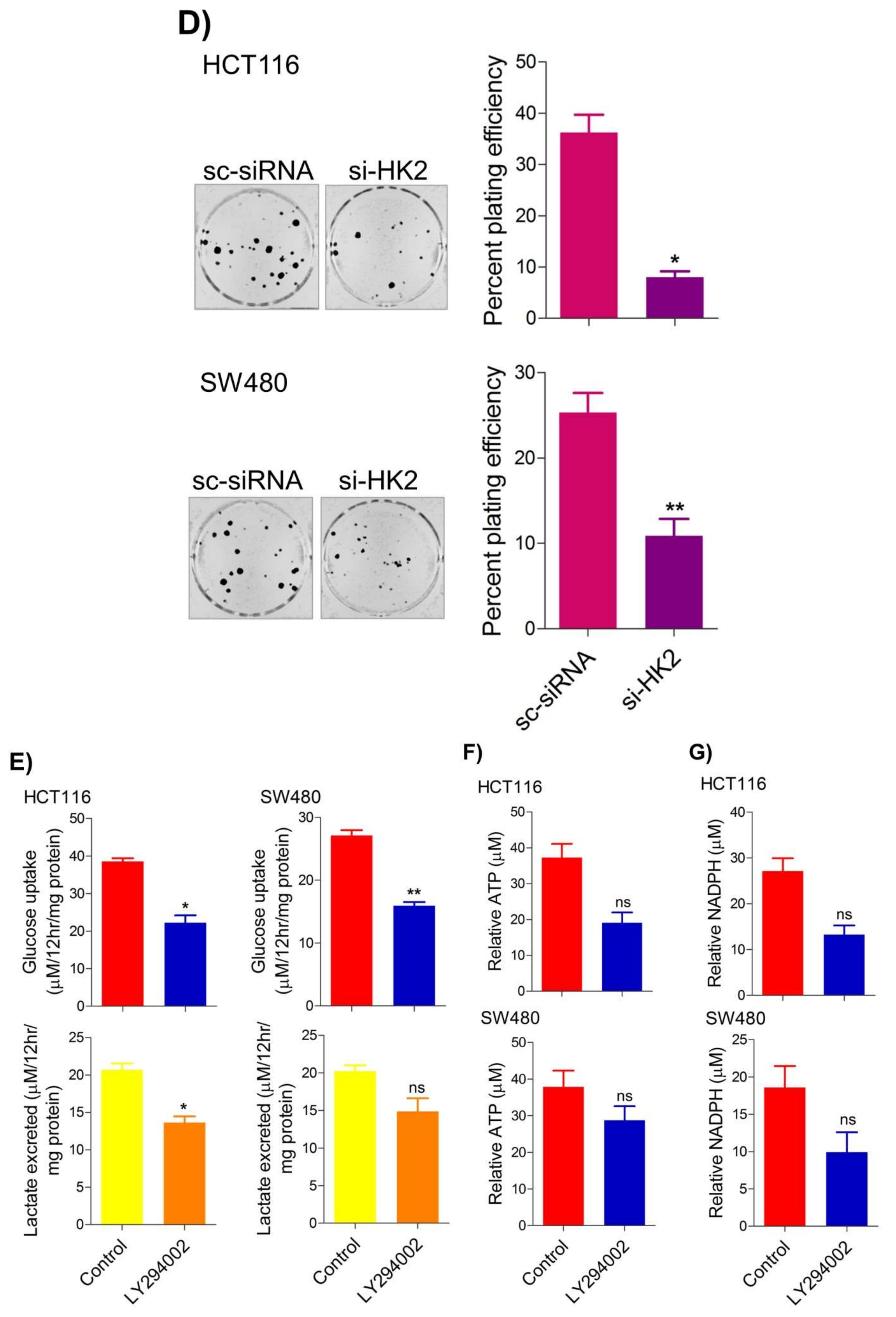
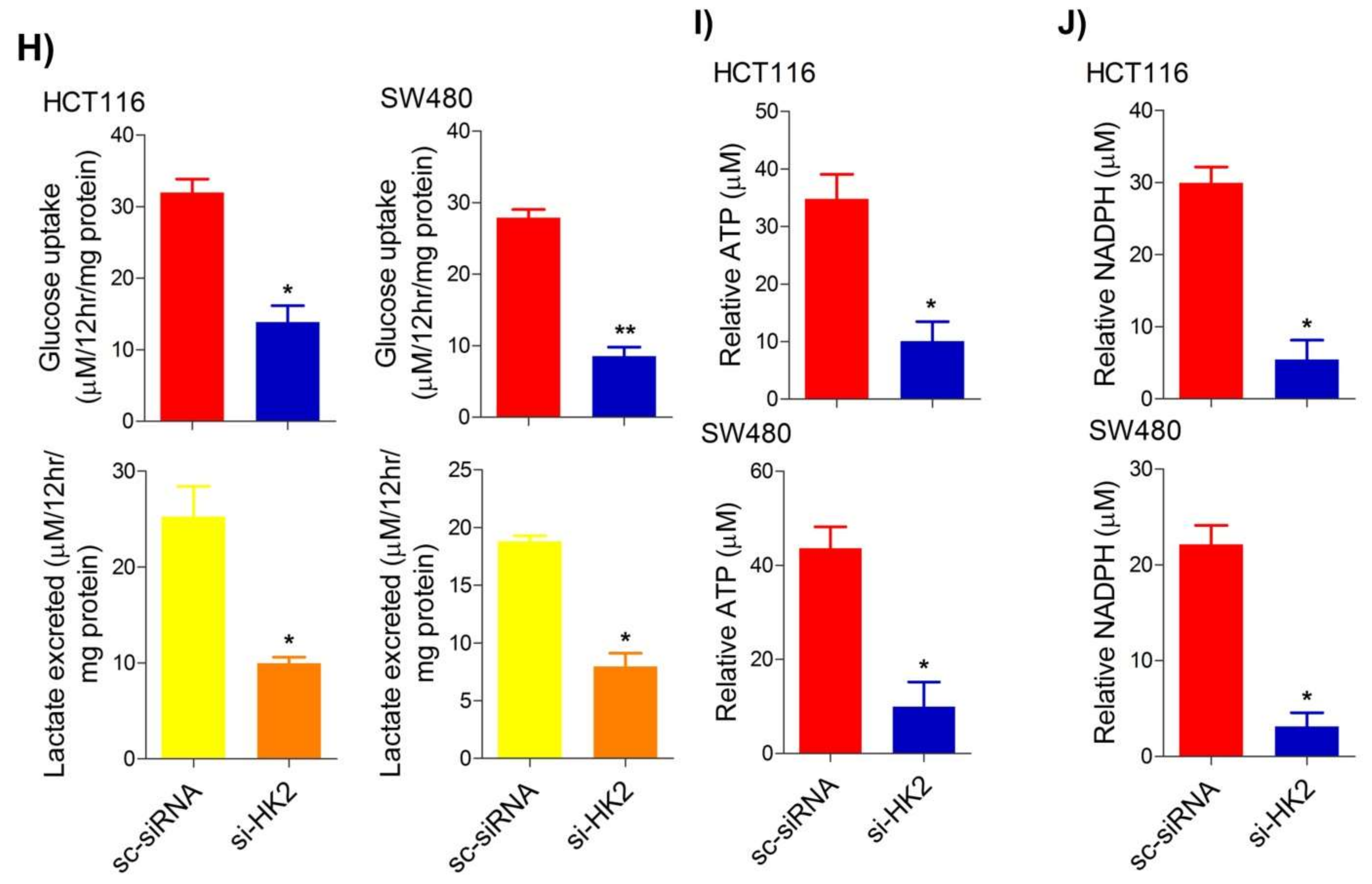
Figure 4. Inhibition of PI3K-AKT/ HK2 pathway impedes tumorigenic potential of CRC cells. HCT116 and SW480 CRC cells were treated as indicated. Inhibition of PI3K with its pharmacologic inhibitor LY294002 led to a significant inhibition in—(A) cell proliferation, and (B) clonogenic propensity of these cells. Genetic (siRNA) or pharmacologic (3-Bromopyruvate) inhibition of HK2 led to a significant reduction in—(C) proliferation, and (D) Clonogenic potential of these cells. Statistical analyses were carried out employing two-tailed paired t-test (proliferation assays) and two-way ANOVA-(Clonogenic assays) followed by appropriate post hoc tests (Bonferroni). Pharmacologic (LY294002) inhibition of PI3K led to an inhibition in (E) glucose fermentation rate/s (glucose uptake and lactate excretion), (F) ATP production and (G) redox state (NADPH). Genetic (siRNA) ablation of HK2 led to a significant abrogation in (H) glucose fermentation rate/s (glucose uptake and lactate excretion), (I) ATP production and (J) redox state (NADPH). Statistical significance was calculated by employing unpaired t-test, data expressed as mean ± SEM (n = 3 or 4). * p < 0.05, ** p < 0.01, *** p < 0.001.
Metastatic dissemination is one of the potent hallmarks of cancer
[2], and with glucose metabolic reprogramming having a strong role in metastatic dissemination
[9][42], their next interest was to seek whether and how thymoquinone impacts metastasis in CRC cells. To this end, researchers found that TQ treatment significantly inhibited the wound healing propensity and invasiveness of HCT116 and SW480 cells (
Figure 5A,B), indicating its anti-metastatic potential. At the molecular level, TQ was able to induce E-cadherin while inhibiting N-cadherin expression (
Figure 5C). Further, to confirm whether such inhibition in metastatic propensity was through glucose metabolic reprogramming, researchers employed genetic ablation of HK2 to inhibit the glucose uptake and hence glycolysis, which was found to rescue both wound healing as well as invasiveness in HCT116 and SW480 cells (
Figure 5D,E). At the molecular level, such inhibition in glycolysis led to the induction of E-cadherin with a concomitant inhibition in N-cadherin levels (
Figure 5F). Taken together, these data suggest that TQ inhibits metastatic predisposition of CRC cells, possibly via modulation of glucose metabolic reprogramming.
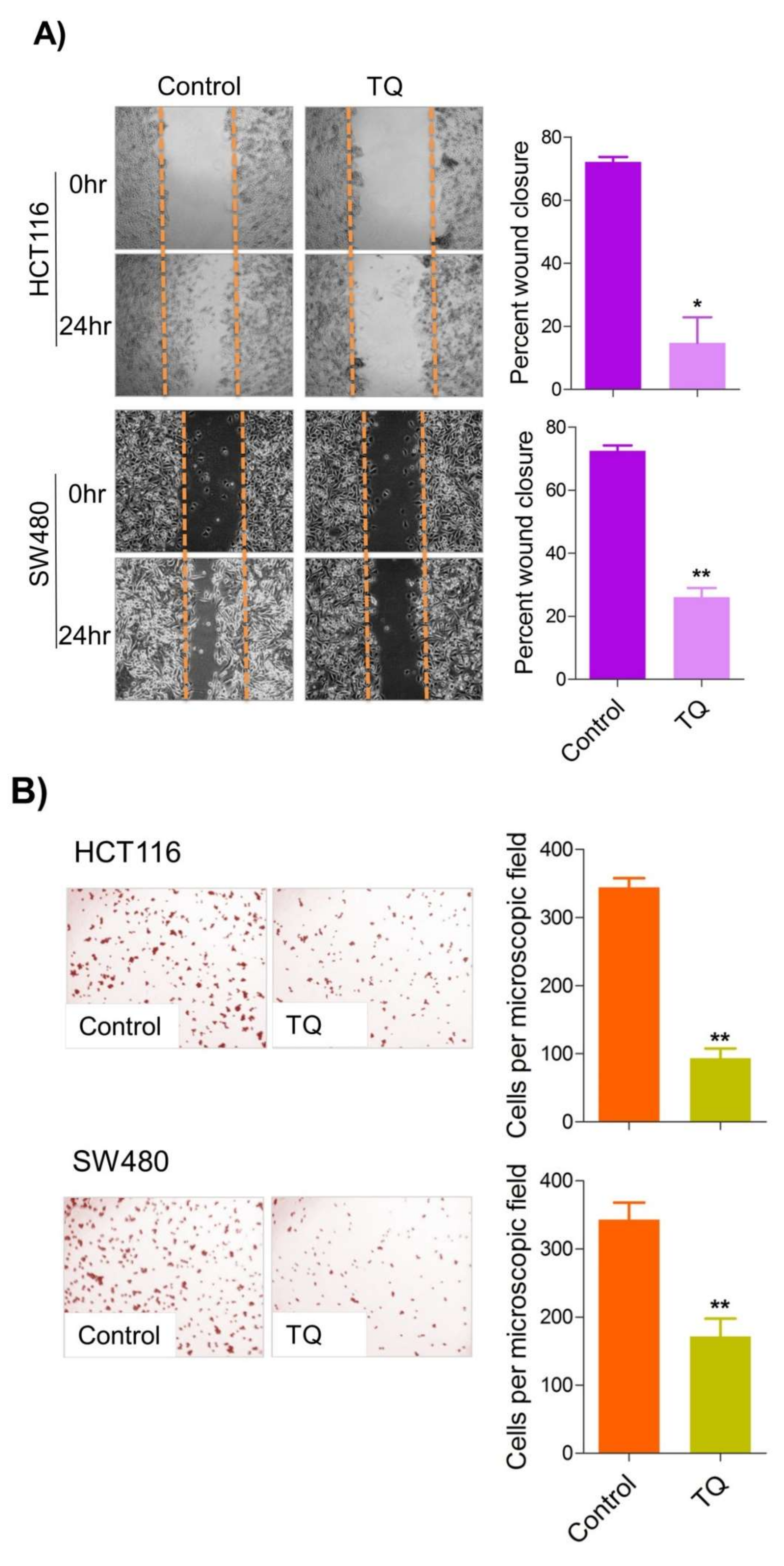
Figure 5. Thymoquinone inhibits cell migration and invasion via modulating glucose metabolic reprogramming. HCT116 and SW480 CRC cells were treated as indicated. Treating these cells with thymoquinone led to a significant inhibition in—(A) wound healing, and (B) invasion potential. (C) Thymoquinone also led to induction in E cadherin with a concomitant inhibition in N cadherin levels. Genetic (siRNA) ablation of HK2 led to a significant inhibition in—(D) wound healing, and (E) cell invasion. (F) HK2 ablation also led to induction in E cadherin and inhibition in N cadherin levels. Statistical analyses were carried out by employing two-tailed paired t-test, data expressed as mean ± SEM (n = 3). * p < 0.05, ** p < 0.01.