In recent years, much interest has been generated by the idea that nitrosative stress plays a role in the aetiology of human diseases, such as atherosclerosis, inflammation, cancer, and neurological diseases. The chemical changes mediated by reactive nitrogen species (RNS) are detrimental to cell function, because they can cause nitration, which can alter the structures of cellular proteins, DNA, and lipids, and hence, impair their normal function. One of the most potent biological nitrosative agents is peroxynitrite (ONOO−), which is produced when nitric oxide (•NO) and superoxide (•O2−) are combined at extremely rapid rates. Considering the plethora of oxidations by peroxynitrite, this makes peroxynitrite the most prevalent nitrating species responsible for protein, DNA, and lipids nitration in vivo. There is biochemical evidence to suggest that the interactions of the radicals NO and superoxide result in the formation of a redox system, which includes the reactions of nitrosation and nitration, and is a component of the complex cellular signalling network. However, the chemistry involved in the nitration process with peroxynitrite derivatives is poorly understood, particularly for biological molecules, such as DNA, proteins, and lipids.
1. Protein Nitration
Peroxynitrite derivatives can modify proteins, promoting changes in protein function through oxidation/nitration mechanisms, with biological relevance, by producing new functions, protein aggregation, turnover, signalling, and immunological processes
[1]. Peroxynitrite is involved in cell signalling processes, and some peroxynitrite-modified proteins have been found to be immunogenic, and implicated in the aetiology of several diseases. Among the molecular after-effects of peroxynitrite, the tyrosine-nitrated proteins are of prime relevance
[2]. The nitration of tyrosine residues has been observed in a variety of processes, including those associated with oxidative stress, such as inflammatory, neurodegenerative, and cardiovascular diseases
[3]. The oxidative inflammatory environment dictates the production and pro-oxidative reactivity of NO metabolites, as well as the pro- and antioxidant activity of
•NO itself. Proteins and polyunsaturated fatty acids (PUFAs) can be oxidised, nitrosated, or nitrated, depending on the redox state. The nitration of protein tyrosine residues has been identified as one of the primary characteristics of peroxynitrite and peroxynitrite-derived species’ interactions with biomolecular targets. The chemical reactions involved in protein oxidation and nitration are outlined below, in
Figure 1 and
Figure 2.
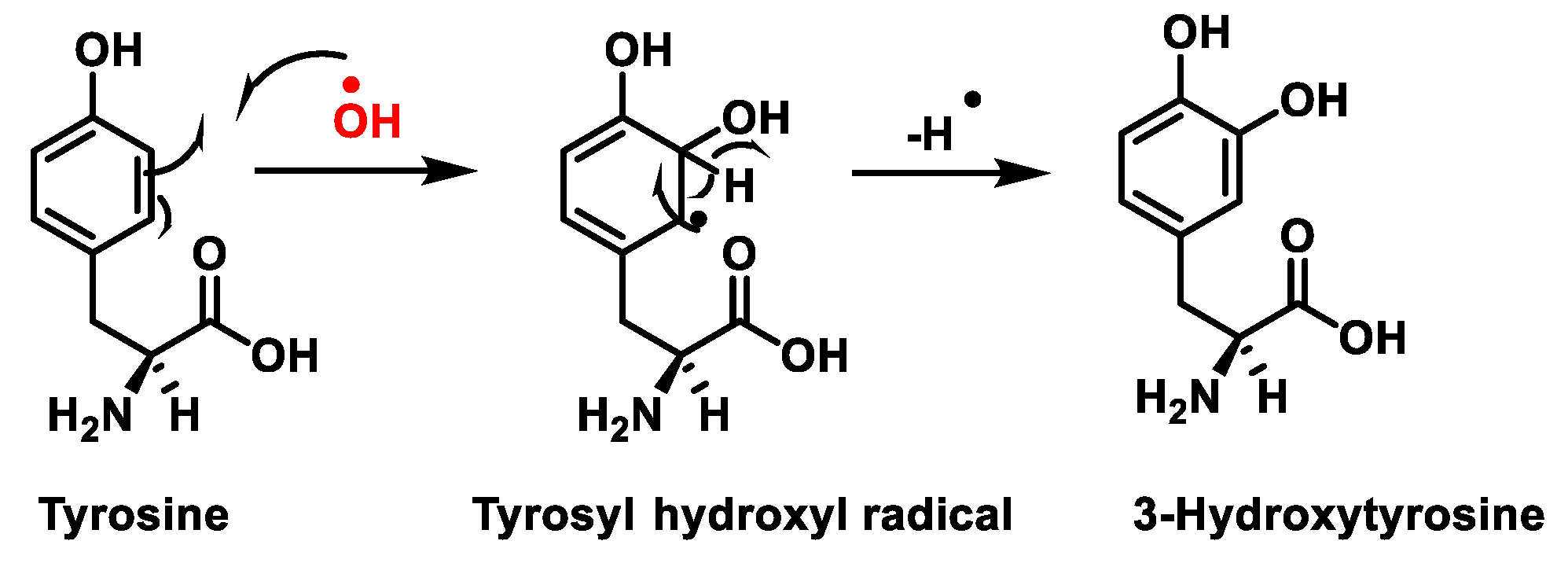
Figure 1. Tyrosine oxidation with hydroxyl radical •OH to hydroxytyrosine.
Figure 2. Tyrosine nitration with •NO2 and nitrosation with •NO to nitrotyrosine.
Protein nitration in tyrosine residue is a covalent protein modification from the addition of a nitro •NO2 group adjacent to the hydroxyl group on the aromatic ring. A stable product 3-nitrotyrosine is formed via the addition of •NO2 to the ortho position of tyrosine, Figure 2. The proximity of catalytic metal centres appears to be of critical relevance in the process of Tyr-nitration. This allows for more selectivity and, in certain cases, greater specificity in the nitration of the Tyr residue.
The nitration of tyrosine residues has been observed in a variety of processes, including those associated with oxidative stress, such as inflammatory, neurodegenerative, and cardiovascular diseases
[3]. A key role in cellular defence against oxidative stress is played by Mn superoxide dismutase MnSOD or SOD2. Several residues of MnSOD (including Tyr34, Tyr9 and Tyr11) are susceptible to nitration, and the loss of MnSOD activity on Tyr34 nitration implies its inactivation
[4]. Some amino acids can react directly with peroxynitrite: cysteine, methionine, and tryptophan, and others do not react directly with peroxynitrite (e.g., tyrosine, phenylalanine, and histidine), but can be modified through secondary species, such as hydroxyl, carbonate, and nitrogen dioxide radicals. In contrast to tyrosine, tryptophan has many sites to be nitrated.
2. Lipid Nitration
Lipid peroxynitrite-mediated oxidation and nitration, including cell membranes and lipoproteins, lead to the formation of new signalling modulators, modifying the role of key lipid-metabolising enzymes
[5]. The chemical reactions on lipid oxidation and nitration are outlined below,
Figure 3. It is believed that NO
2-FAs are formed by the non-enzymatic interaction of unsaturated fatty acids with NO-derived species, such as nitrogen dioxide (NO
2), nitrite (
•NO
2), or peroxynitrite (ONOO
−); nevertheless, the exact process by which fatty acids are nitrated in vivo is unclear as yet
[6]. However, nitrated unsaturated fatty acids have chemically electrophilic characteristics that allow them to facilitate reversible nitroalkylation reactions (Michael reaction), with deprotonated thiolate anions such as cysteine thiol groups in proteins, or peptides such as glutathione. Furthermore, the formation of NO
2-FAs in a lipophilic environment, such as the bilayer of cellular membranes, provides a suitable signal transduction pathway. Nitrated lipids also serve as signalling molecules, since modest quantities of these molecules can act as effective mediators for signal-transduction cascades when present in high concentrations.
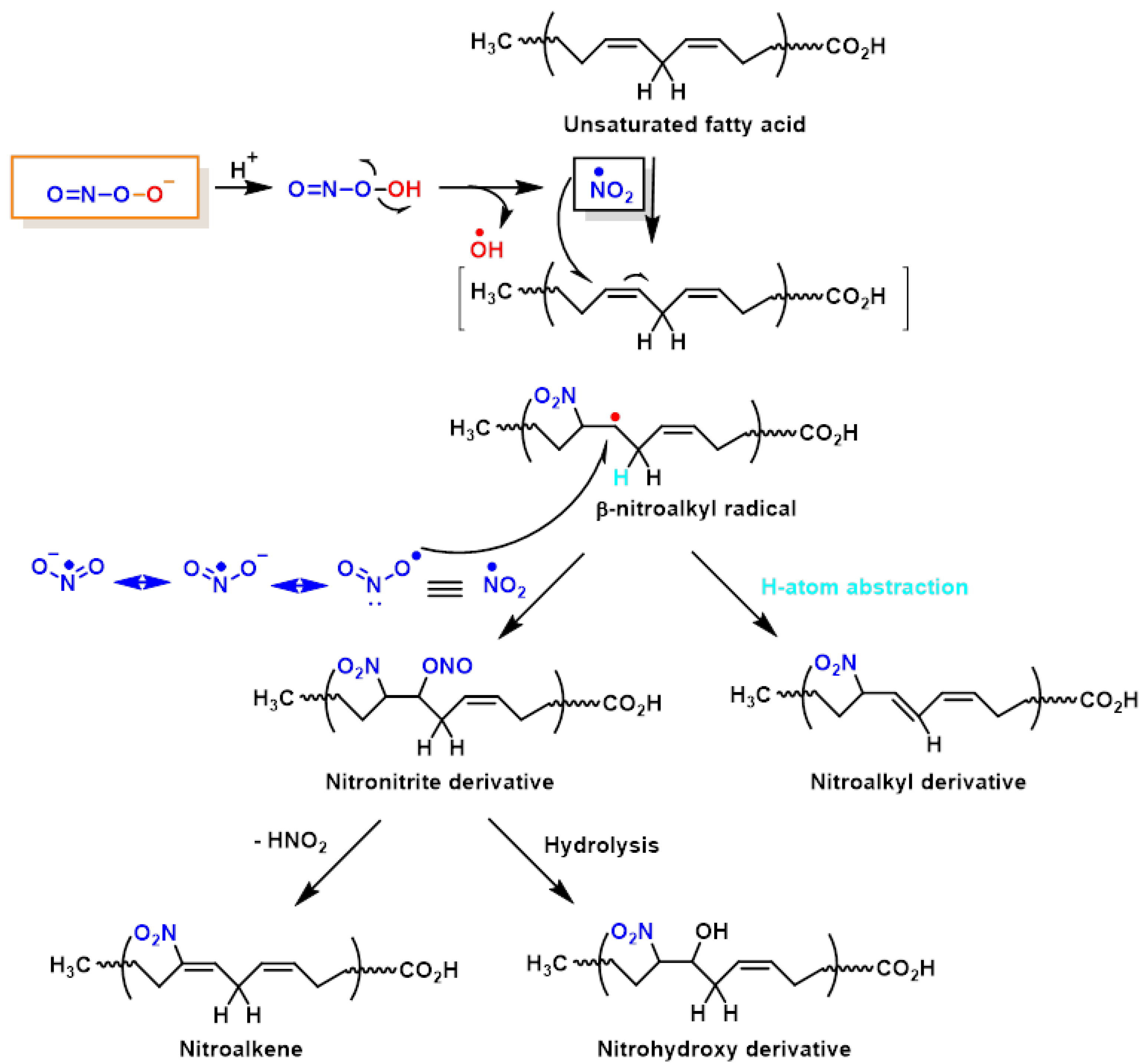
Figure 3. Lipid oxidation and nitration to nitrohydroxyderivatives.
3. DNA Nitration
Peroxynitrite can cause DNA strand breaks and even react with DNA, causing damage to both the sugar and the bases in the nucleus. Of the four DNA bases, guanine has the lowest oxidation potential (E° = 0.81 V) and is the most reactive, giving 8-oxoguanine and 8-nitroguanine, oxazolone, and its precursor, imidazolone
[7]. The chemical reactions on guanine oxidation and nitration are outlined below,
Figure 4.
Figure 4. Guanine reactions with peroxynitrite to 8-oxoguanine and 8-nitroguanine.
4. Role of •NO as a Potential Treatment against COVID-19
COVID-19 is both a respiratory and a vascular disease, especially for patients with severe symptoms
[8]. The virus SARS-CoV-2, with a size of 80–100 nm, infects and replicates in endothelial cells EC before infecting the underlying tissue, as the EC is the major source of
•NO synthesis in mammals.
•NO is an antimicrobial and anti-inflammatory molecule that plays an important role in vascular and pulmonary function against viral infections
[9]. In the case of the SARS-CoV-1 virus, it caused the severe acute respiratory outbreak in 2003,
•NO inhibited viral replication through cytotoxic reactions via intermediates such as peroxynitrite
[10].
•NO derivatives are antimicrobial, with key roles in pulmonary vascular function in the context of viral infections and pulmonary diseases. At this point,
•NO emerges as a potential treatment against COVID-19
[11].
Reactive oxygen species, such as superoxide anion
•O
2−, serve as the host defence and are generated during viral infection. Excess ROS activate M1 macrophages, recruit neutrophils, and increase peroxynitrite production
[12], which act as a potent response to the invading virus, but induce collateral damage, such as endothelial dysfunction, vessel permeabilisation, and lipid membrane peroxidation. Factors that contribute to regulating the inflammatory and immune response include the nitric oxide/reactive oxygen species
•NO/ROS ratio, M1 macrophage activation, and induced red blood cell RBC damage
[13]. Inducible NOS is commonly elevated during infection by viruses, as in SARS-CoV-1 infection
[14].
•NO generated from endothelial eNOS decreases with age, and patients with chronic vascular inflammation, such as diabetes mellitus type 2, metabolic syndrome, chronic obstructive pulmonary disease, obesity, hypertension, autoimmune disorders, and haemoglobinopathies, may produce less eNOS. Simultaneously, this enzyme, under oxidative stress, reduces its expression and
•NO production
[15].
The
•NO-deprived vasculature suffers from persistent inflammation, reduced oxygen supply, and the elimination of toxic by-products generated during infection, due to reduced blood flow to and from the hypoxic tissue. Both NO and its generated RNS can have substantial impacts on mitochondrial activity. Nitric oxide interacts strongly with all heme moieties, reducing the activity of iron-containing enzymes, such as cytochrome c oxidase (complex IV). The electron transport chain is inhibited by nanomolar
•NO, which competes with O
2 for electrons. Supplementation with
•NO-generating compounds prevents cytokine storming, and restores functional capillary density, which is crucial for oxygen supply to organs that are sensitive to oxygen deprivation, such as the kidneys
[16]. Older people with vascular stressors may have low levels of vascular
•NO, increasing their vulnerability. The supplementation of arginine for specific patient populations may be a treatment that reduces viral load in the lungs and prevents the cascade of negative events associated with infection
[17], but above all, restoring
•NO bioavailability may be a genuine preventive or early treatment option for COVID-19
[18].