High-grade gliomas (HGG) are the most common primary malignant brain cancer in adults. Complete surgical removal of HGG is known to increase the overall survival and progression-free survival. Fluorescence-guided surgery with 5-aminolevulinic acid (5-ALA) increases gross total resection considerably compared to white light surgery (65% vs. 36%). Recently, an off-label fluorophore, sodium fluorescein (SF), has become popular in fluorescence-guided surgery due to numerous utility benefits compared to 5-ALA, including lower cost, non-toxicity, easy administration during surgery and a wide indication for other CNS tumors.
1. Introduction
High-grade gliomas (HGGs) arise from astrocytes or oligodendrocytes. They are classified as WHO grade III–IV
[1], with an aggressive growth pattern
[2][3], a poor prognosis and a median overall survival of approximately 12 to 15 months from diagnosis
[4][5]. The current treatment consists of the maximum safe resection followed by chemotherapy and radiotherapy
[6]. It is well-documented that the extent of resection (EOR) correlates positively with improved overall survival (OS) and progression-free survival (PFS)
[7][8]. Surgery is commonly aimed at gross total resection (GTR) with removal of all contrast-enhancing tumor. However, a recent study by Glenn et al.
[9] showed that supramaximal resection (SMR), i.e., resection beyond the boundaries of contrast enhancement, further improved PFS and OS for the 32 glioma patients included in the study compared to GTR and subtotal resection (STR), respectively, with PFS = 15 months vs. 7 months vs. 6 months (
p < 0.003) and OS = 24 months vs. 11 months vs. 9 months (
p < 0.004). At the same time, the authors found no statistically significant differences in the postoperative rate of complications between the groups
[9]. These findings support the notion that glioma surgery should aim for resection to the functional limit of the peritumoral region. Although an interesting prospect, this concept is associated with technical challenges as access to functional intraoperative mapping techniques is limited in some centers. Furthermore, it can be difficult to distinguish healthy brain tissue from tumor-infiltrated tissue when surgery is performed under white light, which is the most common technique in many centers (
Figure 1A). These issues result in suboptimal EOR with historical GTR rates of approximately 36–52%
[10][11] despite the use of supportive technologies such as neuronavigation systems and intraoperative MRI.
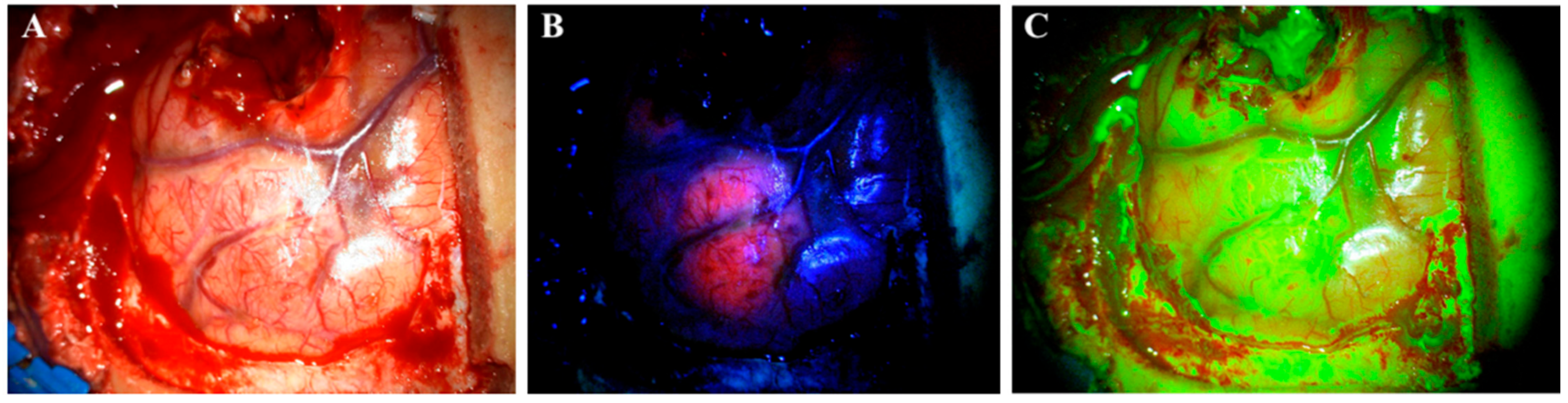
Recently, fluorescence-guided surgery (FGS) has been becoming increasingly popular among neurosurgeons as a tool to distinguish between pathological and nonpathological tissue intraoperatively
[12][13]. The only approved drug for fluorescence-guided glioma surgery is 5-aminolevulinic acid (5-ALA), although sodium fluorescein (SF) has been used increasingly (off-label) by many neurosurgeons due to several benefits. The use of 5-ALA is supported by multiple studies demonstrating superior evidence level compared to SF
[14].
2. Agents for FGS (5-ALA and SF)
Currently, 5-ALA is the only approved drug for FGS. It is administrated orally three hours before surgery in doses of 20 mg/kg
[10][11]. However, a recent study indicated that the maximum fluorescence occurs 7–8 h after administration and even later in marginal tumor tissue (8–9 h)
[15]. One study even reported fluorescence of 5-ALA 28 h after administration
[16]. In addition to glioma surgery, 5-ALA also has other medical applications, such as photodynamic therapy of actinic keratosis, superficial basal cell carcinomas
[17][18] and urothelial carcinomas
[19]; 5-ALA is a natural precursor molecule to the hemeprotein of hemoglobin synthesis. The compound undergoes a number of intracellular transformations in the cytosol and is transferred to the mitochondria to complete the synthesis. Protoporphyrin IX (PPIX) is the last compound in the synthesis pathway before the enzyme ferrochelatase adds the Fe
2+ ion and transforms it into a heme. By similar mechanisms, exogenous 5-ALA is taken up by cells and then transformed into PPIX. Due to the downregulated level of ferrochelatase in glioma cells, PPIX accumulates in them instead of in normal cells
[20]. PPIX has fluorescent properties and absorbs light in the blue spectrum (375–440 nm) to emit a red–violet light (640–710 nm) upon fluorophore relaxation. This enables neurosurgeons to visualize glioma tissue with accumulated PPIX under a surgical microscope using a red light filter
[21] (
Figure 1B).
Contrary to 5-ALA, SF is an unspecific fluorescent marker of a disrupted blood–brain barrier (BBB). The mechanism of action for SF is different from 5-ALA as it is not accumulated intracellularly in tumor cells, but rather is distributed and accumulated in the extracellular space throughout brain areas where the BBB is disrupted, e.g., by tumor infiltration
[22]. Thereby, SF is not selectively used for HGGs, but rather accumulates in all tumors with contrast enhancement on CT or MR
[13]. When first introduced, SF was administrated intravenously in high doses (20 mg/kg) and visualized under a surgical microscope illuminating white light. Recently, the surgical procedure changed and SF is now administrated at the beginning of an operation in low doses of 3–5 mg/kg
[13]. SF has the light absorption maximum at 494 nm and emits green fluorescence at 540–690 nm. It is visualized under a surgical microscope with a yellow (560 nm) filter (
Figure 1C). SF can be visualized up to 4 h after administration
[23].
3. White Light Surgery Compared to 5-ALA-Guided Surgery in HGGs
Many studies have found increased GTR, OS and PFS with 5-ALA-guided HGG resection compared to conventional white light surgery. Specifically, a recent meta-analysis found GTR rates of 79.1% with 5-ALA use compared to 52.8% without 5-ALA
[10]. The OS was increased by approximately three months (95% CI, 2.43–3.68 months;
p < 0.001), PFS—by approximately one month (95% CI, 0.61–1.45 months;
p < 0.001) when using 5-ALA compared to conventional surgery.
In 2006, a pivotal randomized controlled trial (RCT) was performed to evaluate the efficacy of 5-ALA for HGG surgery
[11]. The study found a significant difference in GTR rates between the groups, with GTR = 65% in the 5-ALA group and 36% in the white light group (
p < 0.0001).
4. SF-Guided Surgery Compared to White Light Surgery in HGGs
In recent years, SF has become the preferred agent for fluorescence-guided surgery for HGG in some centers, although the evidence is less compared to 5-ALA.
Several studies report an increased GTR rate in SF-guided tumor resection compared to conventional white light surgery (73.4–85.7% vs. 30.1–62.5%, respectively)
[24][25][26][27][28][29]. However, it is important to note that the level of evidence in the available literature is class III or below as all the studies have important caveats that must be considered, such as lack of randomization and small sample size
[30].
5. Direct Comparison of 5-ALA and SF
Until recently, all evidence of SF-guided surgery was based on uncontrolled studies or studies comparing SF to white light surgery alone
[24][25][26][27][28][29]. As 5-ALA guidance is a standard practice technology for HGG resection in many centers, studies that directly compare SF with 5-ALA are necessary to investigate the superiority or potential equivalence of either compound based on GTR or survival endpoints. In addition, such studies would also clarify the potential practical and health economic benefits of one agent over the other.
In 2019, some of these aspects were investigated in a retrospective cohort study by Hansen et al.
[31]. The authors found no significant difference in GTR or CRET between 5-ALA and SF (64% vs. 62% (OR, 0.90,
p = 0.76) and 29.5% vs. 36.2% (OR, 1.34,
p = 0.86), respectively).
Although the evidence of 5-ALA is superior in HGG surgery, it is well-known that it does not have an essential part in the removal of brain metastases. In 2019, Marhold et al., administrated 5-ALA to 157 different brain metastases and found that only 66% of the tumors exhibited visible fluorescence
[32]. Hence, 5-ALA is not reliable to use in brain metastases and therefore is not routinely used.
Currently, the strongest evidence for FGS in brain metastases is based on the use of SF. Five clinical studies have investigated SF for this indication with the GTR rate as the primary endpoint
[33][34][35][36][37]. Only two studies compared SF directly to white light surgery
[36][37], while the others did not include a control population. Studies with control group found a significant higher GTR in the SF group compared to the white light group (94% and 62-84%, respectively)
7. Conclusions
It is found that there is generally solid evidence for the use of FGS for multiple indications in neuro-oncology. Although 5-ALA remains the gold standard for FGS in HGG surgery, recent studies have indicated that SF may be a plausible alternative for HGG surgery. Additional comparative studies with 5-ALA and SF are needed to determine if SF is a feasible alternative based on GTR. Furthermore, SF is useful for a multitude of contrast-enhancing CNS tumors, including brain metastases. SF has several benefits over 5-ALA, including a broader spectrum of indications, lower cost and more practical utility features.