Detection of intermediates during the catalytic process by infrared techniques has been widely implemented for many important reactions. For the reduction of CO2 into hydrocarbons on metal surfaces, CO molecule is one of the most important transient species to be followed due to its involvement in several products’ pathways, and its distinct vibrational features.
1. Introduction
Successful reduction of CO
2 gas into hydrocarbons “reverse fuel” will contribute to solve two overlapping global problems related to the global warming caused by the excess of CO
2 in the atmosphere, and the high demand of hydrocarbons to be utilized in the expanding urbanization
[1][2]. However, attaining an efficient route to do this reduction process is still in demand, since many parameters are not well under control. Knowing these parameters will happen by understanding key intermediates for such reduction process, especially under operational conditions such as the presence of external bias
[3][4][5][6][7].
One of these important intermediates during the CO
2 reduction process is the CO (carbon monoxide). CO is an important surface intermediate for many catalytic reactions including methanol oxidation, and CO
2 reduction process
[8]. Depending on the nature of the catalyst and the working conditions with respect to electrolyte, pH, temperature, and other diverse ions, several reactions of CO
2 reduction can take place. Each of the following reactions has required redox energy (versus SHE at pH = 7) (Some literature uses NHE instead; however, the relation between them is (
ESHE =
ENHE+0.059
pH)), and certain amount of moles for electrons and protons are needed. The lowest kinetic parameter needed to reduce CO
2 is by using two moles of electrons as the following equation
[3][9][10].
Mixing the resultant CO and H
2 gases on metal surfaces under different conditions can produce varieties of hydrocarbons such as CH
3CH
2OH, CH
3OH, and CH
4 [11].
In addition, CO is one of the most transient species (COOH*, CO*, CHO*, and CH
2O*) that show strong vibrational bands in the infrared (IR) region, allowing for better detection and analysis, see
Figure 1 [6][12]. Moreover, due to the adsorption of CO
2 and its transient species like CO on material surfaces, the concentration of such molecules is extremely low, and special spectroscopic techniques are needed to access such low concentrations with high accuracy. IR surface sensitive techniques can, however, be more sensitive than other techniques such as gas chromatography
[2].
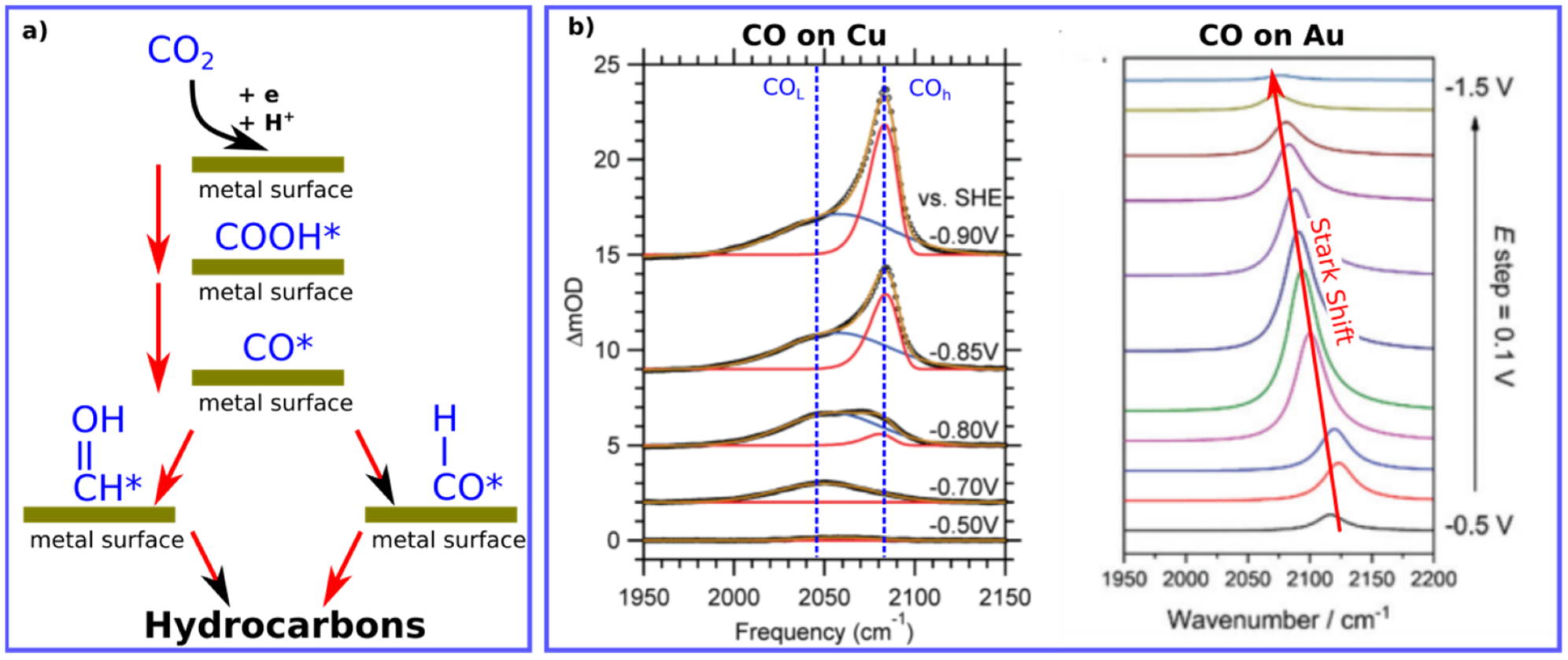
Figure 1. (
a) Illustration for the important of CO intermediate during the reduction process of CO
2 into hydrocarbons. (
b) Typical positions of adsorbed CO molecules on Cu (ATR-SEIRAS) and Au (VSFG) surfaces found by IR surface-sensitive techniques. Data are adapted with permissions from references
[7][13].
Various spectroscopic techniques at different spectral range can generally be utilized to investigate the dynamics of reactants, CO herein, at surfaces and interfaces such X-ray spectroscopic techniques
[14][15]. However, utilizing the IR is more applicable for many groups due to its relative simplicity and accessibility in comparison to other methods based on X-rays. Moreover, IR energy is considered as a non-destructive source of energy that doesn’t generally cause any sample degradation or sample ionization; however, sample heating is expected. The IR source has to be utilized in a specific manner to be surface sensitive such as in ATR-SEIRAS (Attenuated total reflectance−surface enhanced infrared absorption spectroscopy), and (Vibrational sum frequency generation) VSFG.
Figure 1 shows typical IR signatures for CO molecules adsorbed on metal surfaces during electrochemical processes by both techniques. The position of CO can vary depending on the utilized metal, applied voltage and the IR technique as well. For instance, CO on Cu measured by ATR-SEIRAS shows two vibrational bands, while change of on CO vibrational position on Au is shown using VSFG, see
Figure 1.
Herein, general overview of the IR spectroscopy along with main IR sensitive techniques utilized (SEIRAS and VSFG) for detecting CO on metal surfaces along with literature values will be discussed, aiming for better understanding of the implications for utilizing such methods for following reaction dynamics of CO2 reduction on metal surfaces.
2. IR Spectral Signatures for CO on Metal Surfaces
Table 1 shows a list of IR signatures for CO detected on various metal surfaces using common IR techniques (SEIRAS, VSFG). Clearly, copper and gold are one of the most studied metal surfaces compared to other metals
[12]. Generally Ag, Au, and Cu have moderate binding energies towards CO species, allowing them to produce hydrocarbons more than other metals that strongly stick CO molecules such Ir
[16], see
Figure 2. Among various metals, copper is known by efficient conversion of CO
2 into hydrocarbons due to its intermediate binding energies towards various intermediate species (COOH, CO, CHO, and CH
2O)
[12][16][17]. On the other hand, copper is known by its weak stability towards corrosion and weak selectivity for specific products. However, gold is a robust metal that provide stable and steady surface property, making it attractive to study primary steps for the reduction of CO
2 molecules. Still, gold is not an efficient surface to produce hydrocarbons, due to the higher energy required to stick species such as CHO and CH
2O, see
Figure 2. Clearly from
Table 1, more studies using SEIRAS more than VSFG are found, reflecting the ease of utilizing SEIRAS technique over VSFG as mentioned before.
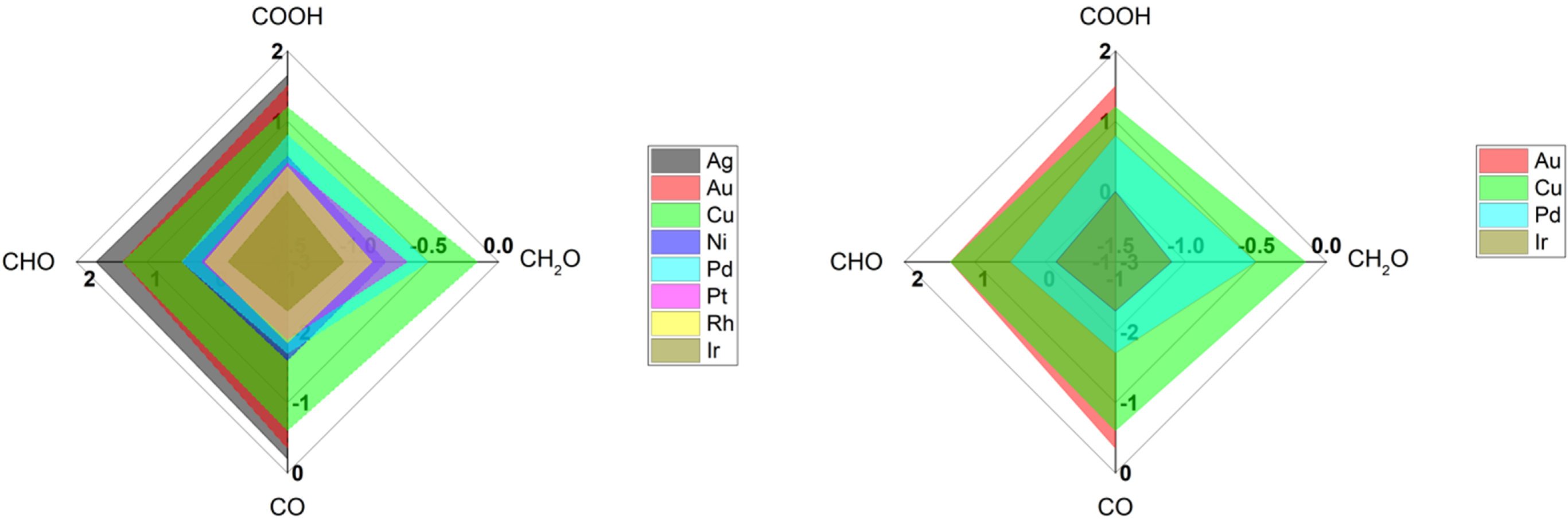
Figure 2. Radar plot for the binding energies for various key intermediate species for reduction of CO
2 into hydrocarbons on various metal surfaces. The extracted values are found in Nørskov’s paper
[16].
Interestingly, the average frequency positions of CO on Cu and Au surfaces are ca. 2075 cm
−1. and 2100 cm
−1, respectively (see
Table 1). The higher frequency difference for CO on Cu, compared to CO vibration in gas phase, reflects the higher the bond force present between CO and Cu surface in comparison to CO on Au surfaces, matching with the binding energies reported for CO on Cu and Au surfaces
[16], see
Figure 2. Such a small difference in vibrational frequencies can correlate with the binding energies for CO on Cu vs Au, highlighting the ability of CO on Cu to interact with water (or protons) to form hydrocarbons but not on Au surfaces
[6][16].
Interestingly, most of the found peak-positions of CO on metal surfaces indicate towards the preference of atop position for CO molecules on metals. The bridge position of CO is a matter of debate and it has been attributed to some contaminations in recent studies
[18].
Apparently, there are no significant differences between extracted values for CO on metals using SEIRAS or VSFG. On one hand, this is an advantage for the SEIRAS technique as it is easier to operate and utilize. However, VSFG has lots of potential to extract more information like capturing short-lived intermediates, orientation and phase shifts during for the adsorbed CO molecules.
Table 1. Summarized data for the detection of adsorbed CO on metal surfaces using infrared techniques under applied external fields.
Metal |
IR Technique |
Peak Position (cm−1) |
Notes |
References |
Cu |
VSFG |
not available |
assigned to fast CO conversion into products |
[6] |
Cu |
SEIRAS |
2075–2065 |
peak positions depend on applied voltage |
[19] |
Cu |
SEIRAS |
2083–2052 |
peak positions depend on applied voltage |
[17] |
Cu |
SEIRAS |
2075–2025 |
peak shifts with time after CO insertion at specific potential |
[20] |
Cu |
SEIRAS |
2085 (2050) |
high frequency CO and (low frequency CO) |
[13] |
Cu |
SEIRAS |
2090–2060 |
depends on copper surface properties |
[21] |
Cu |
SEIRAS |
2100–2050 (1900–1800) |
atop CO shifts with voltage (bridge CO shifts with voltage) |
[22] |
Ag |
SEIRAS |
1900 |
in ionic liquid |
[23] |
Pt |
VSFG |
2060–2090 |
peak positions depend on applied voltage |
[24] |
Au |
VSFG |
2100–2125 |
peak positions depend on applied voltage |
[7] |
Au |
VSFG |
2110–2075 |
peak positions depend on applied voltage |
[4] |
Au |
VSFG |
2130–2080 |
peak positions depend on applied voltage |
[6] |
Au |
SEIRAS |
2100 |
atop CO don’t shift with applied potential |
[25] |
Au |
SEIRAS |
2100–2075 |
peak positions depend on applied voltage |
[19] |
Au |
SEIRAS |
not available (2100–2075) |
under CO2 atmosphere due to adsorption instability of CO on Au but only under (CO atmosphere) |
[18] |
Au |
ATR-SEIRAS |
2100–2120 |
peak positions depend on the applied voltage |
[18] |
3. Conclusions
The Electrocatalytic reduction of CO2 into hydrocarbons on metal surfaces is limited by several factors and to disassemble such process, IR surface sensitive techniques are excellent tools to achieve such approaches. Following the IR signature of CO during the catalysis process is very important due to the involvement of CO transient species in many reaction paths of CO2 reduction process, and the ability to capture the vibrational modes of CO on metal surfaces. As shown from this brief summary about the common IR techniques for detecting CO on metal surfaces, VSFG and SEIRAS are the common IR techniques to follow the electrochemical conversion of CO2 into hydrocarbons. The extracted data from IR measurements can be correlated with other theoretical approaches to understand such as the binding energies for various species. Both techniques have different geometrical configurations and can reveal different information for the catalytic process of CO2. From the current results present in the literature, there is no significant information that one technique can provide over the other one, despite the fact that VSFG can theoretically provide more information than SEIRAS, such as the contribution of surface response, the distinction between surface and double region dynamics, and the effect of dipole alignments on surfaces. Thus, clearly, due to its ease of utilization and low cost, SEIRAS is more common than VSFG to follow the electrochemical process of CO2 on metal surfaces. However, VSFG should be utilized under various conditions to explore more information that could not be shown by SEIRAS.