Polyhexamethylene biguanide (PHMB) is an antimicrobial that has been actively used in the domestic and clinical application for the past two decades. The compound work effectively
Polyhexamethylene biguanide (PHMB) or polyhexanide is a cationic polymer composed of repeating biguanides group linked by hexamethylene chains (Figure1).91This polymer exhibits broad-spectrum antibacterial activity, and has been widely used as antiseptic in medicine, food industry and domestic application. Its applications include impregnation into wound dressing,93water treatment,94mouthwash and disinfection in contact lenses.95Though the compound has been used for the past 40 years, there is no sign of bacterial resistance towards this compound.PHMB exerts antimicrobial activities by interaction of biguanides groups with the cytoplasmic membrane, lipopolysaccharide and peptidoglycan component of the cells wall. This binding displaces divalent cations, Ca2+causing membrane destabilisation and cellular leakages.92Simultaneously, the hexamethylene segment can interact with neutral phospholipids, causing phase separation that disturbs random distribution of lipids.91Furthermore, recent finding in our laboratory demonstrates that PHMB can enter bacteria cells and cause chromosome condensation.96All together, these mechanisms support the longstanding potent PHMB antimicrobial activities.
Additionally PHMB is known to enter mammalian cells and kill intracellular bacteria. PHMB is known to effectively kill intracellular Staphylococcus aureus at low concentrations that was associated with skin infections in human and mastitis in animal and Leishmania species that cause cutaneous leishmaniasis. PHMB showed effective antimicrobial activites to kill the pathogens in the skin cell and bovine mammary epithelial cells and macrophages cells respectively. PHMB kills intracellular MRSA via direct interaction with pathogens inside keratinocytes and host cell entry is dynamin dependentThe mechanism of killing intracellular S. aurues Additionally, can kill intracellular Leishmania inside macrophages. PHMB’s leishmanicidal mechanisms of action appear to involve disruption of parasite cell membranes, cell entry and condensation/disruption of parasite chromosomes. PHMB is known By using Fluorescein-tagged PHMB (PHMB-FITC), PHMB uptake and interaction with the intracellular pathogen inside the host cells. The mechanism for the PHMB uptake and intracellular activities is dynamin dependent.Additionally PHMB also showed effective anti-biofilms activities and these has been demonstrated in multiple pathogens. Additionally, PHMB itself has potent leishmanicidal activity at submicromolar concentrations. PHMB’s leishmanicidal mechanisms of action appear to involve disruption of parasite cell membranes, cell entry and condensation/disruption of parasite chromosomes.
The positive charge of the biguanide group allows PHMB to form complexation with biomolecules. PHMB can binds to nucleic acids forming nanoparticles in vitro [28]. PHMB can form nanoparticles and improve delivery of CpG oligonucleotide (ODN), an immunomodulatory, into macrophages [29,30]. PHMB spontaneously binds CpG ODN and forms stable nanopolyplexes by interacting with CpG ODN at appropriate dose ratios. PHMB also protects and prompts cellular uptake of CpG ODN. Interestingly, the nanopolyplexes effectively counteract the toxicity associated with PHMB by interacting with negatively charged CpG ODN through charge complexation/shielding. Therefore, PHMB/CpG ODN can provide combined host- and pathogen-directed leishmanicidal effects against CL and may provide multiple benefits for CL therapy. Additionally PHMB can form nanoparticles with small molecules antibiotics. PHMB was formulated with nadifloxacin, a topical antibiotic. The nanoparticles killed intracellular MRSA, and relative to free polymer or drug used separately or together the nanoparticles displayed reduced toxicity and improved host cell recovery. Additionally PHMB has been formulated as Together, these findings show that PHMB can effective as be used individually and can be formulated with other biomolecules for improvement of activities and reduced cellular toxicity.
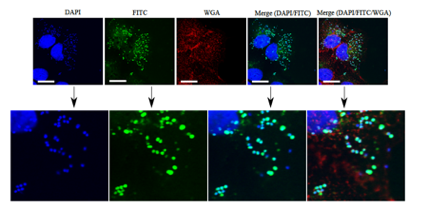
Figure 11. Intracellular localization and bactericidal activities of PHMB against intracellular methicillin-resistant S. aureus (MRSA). Colocalization of fluorescence-tagged PHMB (PHMB-FITC) with intracellular S. aureusstrain EMRSA-15 in keratinocytes. Keratinocytes were infected with EMRSA-15 followed by treatment with PHMB-FITC (green). Keratinocytes were labelled with DAPI (blue) for keratinocytes and EMRSA-15 nuclei staining and WGA (red) for keratinocyte membrane stain. Upper panels are images of infected cells and merged images. Lower panels are enlarged images that clearly show colocalization between PHMB-FITC (green) and EMRSA-15 (blue). White scale bar is 25 μm. Image is reprinted from [22].
References
- WHO High Levels of Antibiotic Resistance Found Worldwide, New Data Shows. WHO, News Release. Available online: https://www.who.int/mediacentre/news/releases/2018/antibiotic-resistance-found/en/(accessed on 29 January 2018).
- O’Neill, J. Antimicrobial Resistance: Tackling a crisis for the health and wealth of nations. Rev. Antimicrob. Resist. 2014, 1, 1–16.
- Boucher, H.W.; Talbot, G.H.; Bradley, J.S.; Edwards, J.E.; Gilbert, D.; Rice, L.B.; Scheld, M.; Spellberg, B.; Bartlett, J. Bad bugs, no drugs: No ESKAPE! An update from the Infectious Diseases Society of America. Clin. Infect. Dis. 2009, 48, 1–12.
- Bjarnsholt, T. The role of bacterial biofilms in chronic infections. APMIS 2013, 121, 1–58.
- Natarajan, S.V.; Dhanasekar, U. Trends in antibiotic resistance pattern against ESKAPE pathogens from tertiary care teaching institute – South India. Infect. Dis. Heal. 2016, 21, 142–143.
- Mohamed, J.A.; Huang, D.B. Biofilm formation by enterococci. J. Med. Microbiol. 2007, 56, 1581–1588.
- Gao, W.; Howden, B.P.; Stinear, T.P. Evolution of virulence in Enterococcus faecium, a hospital-adapted opportunistic pathogen. Curr. Opin. Microbiol. 2018, 41, 76–82.
- Al-Talib, H.; Yean, C.; Al-Jashamy, K.; Hasan, H. Methicillin-resistant Staphylococcus aureus nosocomial infection trends in Hospital Universiti Sains Malaysia during 2002–2007. Ann. Saudi Med. 2010, 30, 358–363.
- Harch, S.A.J.; MacMorran, E.; Tong, S.Y.C.; Holt, D.C.; Wilson, J.; Athan, E.; Hewagama, S. High burden of complicated skin and soft tissue infections in the Indigenous population of Central Australia due to dominant Panton Valentine leucocidin clones ST93-MRSA and CC121-MSSA. BMC Infect. Dis. 2017, 17, 405.
- Enright, M.C.; Robinson, D.A.; Randle, G.; Feil, E.J.; Grundmann, H.; Spratt, B.G. The evolutionary history of methicillin-resistant Staphylococcus aureus (MRSA). Proc. Natl. Acad. Sci. USA 2002, 99, 7687–7692.
- Macmorran, E.; Harch, S.; Athan, E.; Lane, S.; Tong, S.; Crawford, L.; Krishnaswamy, S.; Hewagama, S. The rise of methicillin resistant Staphylococcus aureus: Now the dominant cause of skin and soft tissue infection in Central Australia. Epidemiol. Infect. 2017, 145, 2817–2826.
- Malani, P.N. National burden of invasive methicillin-resistant Staphylococcus aureus infection. Jama 2014, 311, 1438–1439.
- Sisirak, M.; Zvizdic, A.; Hukic, M. Methicillin-resistant Staphylococcus aureus (MRSA) as a cause of nosocomial wound infections. Bosn J. Basic Med. Sci. 2010, 10, 32–37.
- Xie, R.; Zhang, X.D.; Zhao, Q.; Peng, B.; Zheng, J. Analysis of global prevalence of antibiotic resistance in Acinetobacter baumannii infections disclosed a faster increase in OECD countries. Emerg. Microbes Infect.2018, 7.
- Olaitan, A.O.; Morand, S.; Rolain, J.M. Emergence of colistin-resistant bacteria in humans without colistin usage: A new worry and cause for vigilance. Int. J. Antimicrob. Agents 2016, 47, 1–3.
- Rossi, F.; Girardello, R.; Cury, A.P.; Di Gioia, T.S.R.; de Almeida, J.N.; Duarte, A.J.D.S. Emergence of colistin resistance in the largest university hospital complex of São Paulo, Brazil, over five years. Brazilian J. Infect. Dis. 2017, 21, 98–101.
- Sun, L.; Klein, E.Y.; Laxminarayan, R. Seasonality and temporal correlation between community antibiotic use and resistance in the United States. Clin. Infect. Dis. 2012, 55, 687–694.
- Sundqvist, M. Reversibility of antibiotic resistance. Ups. J. Med. Sci. 2014, 119, 142–148.
- Biotech Connection. Trends and Opportunities in Antibiotic Development for Multidrug-Resistant Bacterial Infections. 2018. Available online: https://biotechconnectionbay.org/market-reports/antibioticdevelopment/(accessed on 1 March 2019).
- Kamaruzzaman, N.; Pina, M.; Chivu, A.; Good, L. Polyhexamethylene biguanide and nadifloxacin self-assembled nanoparticles: Antimicrobial effects against intracellular methicillin-resistant Staphylococcus aureus. Polymers 2018, 10, 521.
- Weiner, L.M.; Webb, A.K.; Limbago, B.; Dudeck, M.A.; Patel, J.; Kallen, A.J.; Edwards, J.R.; Sievert, D.M. Antimicrobial-Resistant Pathogens Associated With Healthcare-Associated Infections: Summary of Data Reported to the National Healthcare Safety Network at the Centers for Disease Control and Prevention, 2011-2014. Infect. Control. Hosp. Epidemiol. 2016, 37, 1288–1301.