Guided tissue regeneration (GTR) and guided bone regeneration (GBR) became common procedures in the corrective phase of periodontal treatment. In order to obtain good quality tissue neo-formation, most techniques require the use of a membrane that will act as a barrier, having as a main purpose the blocking of cell invasion from the gingival epithelium and connective tissue into the newly formed bone structure. Different techniques and materials have been developed, aiming to obtain the perfect barrier membrane. The membranes can be divided according to the biodegradability of the base material into absorbable membranes and non-absorbable membranes. The use of absorbable membranes is extremely widespread due to their advantages, but in clinical situations of significant tissue loss, the use of non-absorbable membranes is often still preferred.
1. Introduction
Periodontitis is an infectious disease, often of multifactorial etiology, in which the perio-pathogenic bacterial biofilm plays an important role. The disease is characterized by an inflammatory reaction of the host to the bacterial aggression, reactions on which both local and systemic risk factors can be grafted. In the evolution of periodontitis, through the aggressive action of bacterial factors in the context of hyper-inflammatory status, there is a gradual destruction of the supporting periodontal tissues: periodontal ligaments, cementum and alveolar bone. To address this issue, periodontal therapy has evolved over time through regenerative therapy surgical methods that include guided tissue regeneration (GTR) and bone regeneration (GBR) techniques. Such guided techniques involve isolating the bone defect with the help of barrier membranes, allowing the regeneration of lost and damaged tissues
[1]. The use of a barrier membrane, at the interface with the gingival/epithelial connective tissue and with periodontal ligaments and alveolar bone to promote the regeneration of periodontal tissues is called GTR, and the restoration of alveolar bone sites is called GBR.
The association of barrier membranes and biomaterials of infrabony periodontal lesions investigated in various clinical studies generated significantly better results in terms of attachment gain and reduction of probing depths than the open flap debridement alone
[2][3][4].
In guided tissue regeneration, there is a number of four essential biological principles under the PASS acronym: (a) Primary closure of the surgical wound to allow uninterrupted healing; (b) Angiogenesis for adequate blood supply (supply of nutrients, as well as cell types that facilitate healing); (c) maintaining the Space for bone neo-formation, while blocking the proliferation of soft tissues and (d) wound Stability to allow blood clots to form
[5].
The idea that the repopulation of cells on the root surface after periodontal surgery determines the nature of the attachment that will form is generally accepted. After the periodontal debridement, with or without open flap procedure, the root surface will be repopulated by the fastest cells, which were epithelial cells
[6]. Barrier membranes placed over areas of tissue defect have as their main purpose the blocking of cell invasion from the gingival epithelium and connective tissue
[7]. Barrier membranes require full in situ functionality for 4–6 weeks for periodontal tissue regeneration and 16–24 weeks for bone growth
[8].
There are a number of mandatory requirements for a barrier membrane to be used successfully in guided regenerative therapies; these requirements include (a) good mechanical isolation, occlusion and blocking capabilities; (b) to be biologically active; (c) to be biocompatible; (d) to exhibit tolerance to exposure and (e) to be biodegradable
[9]. Moreover, barrier membranes should have adequate porosity to prevent the excessive penetration of oral keratinocytes into the bone defect on one side but also to allow neovascularization and bone formation in the connective tissue part of the membrane
[10]. It should also be easy to manipulate the membrane, to stabilize it at the site without damaging it
[11].
To date, there is no commercially available membrane that meets all these parameters at optimum capacity, but recent developments in membrane production technology are trying, through various innovative forms, to meet as wide a range of characteristics as necessary to achieve predictable surgical results.
To meet some of these requirements, various techniques and materials have been developed to generate tissue neo-formation. They can be divided according to the biodegradability of the base material into absorbable membranes and non-absorbable membranes
[12] (
Figure 1). The use of absorbable membranes is extremely widespread due to their advantages, but in clinical situations of significant tissue loss, the use of non-absorbable membranes is often still preferred
[13].
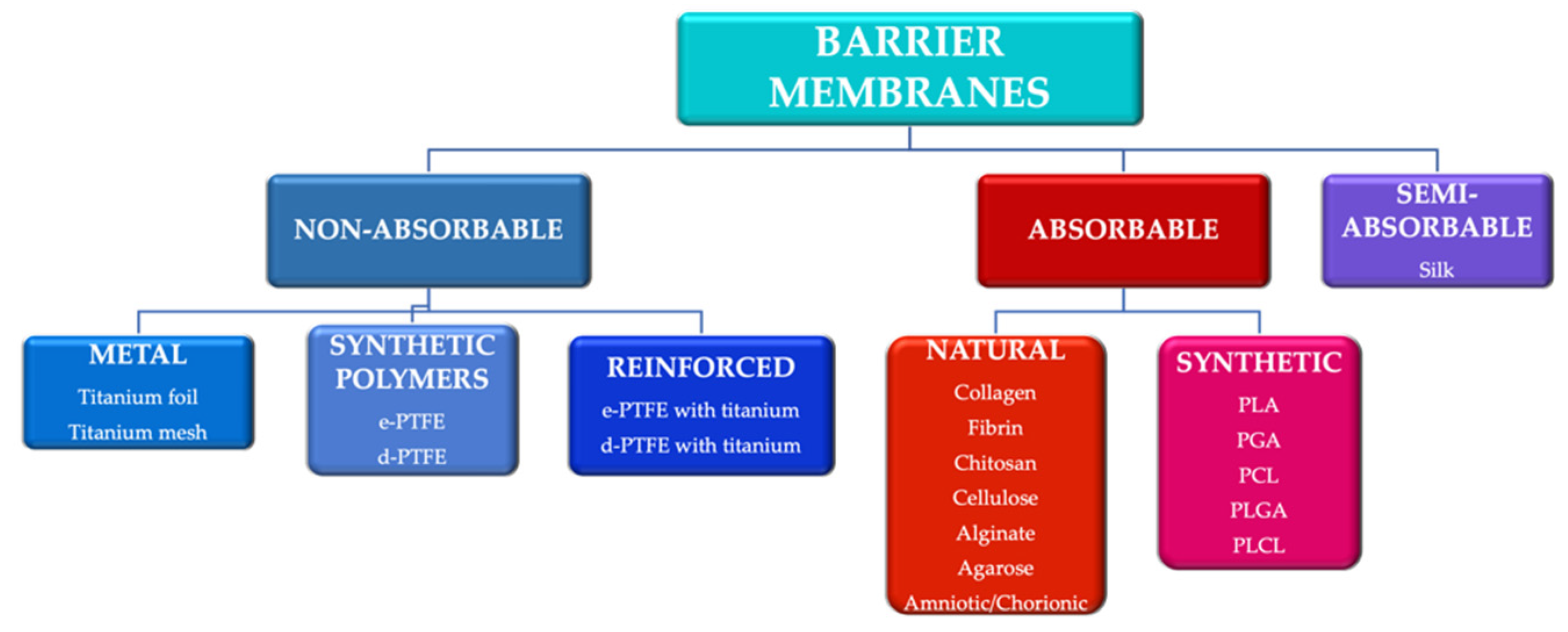
Figure 1. Main categories of barrier membranes used in guided tissue regeneration. e-PTFE: expanded polytetrafluoroethylene; d-PTFE: high density polytetrafluoroethylene; PLA: polylactic acid; PGA: polyglactic acid; PCL: polycaprolactone; PLGA: poly-lactic-co-glycolic acid PLCL: poly-lactic-co-caprolactone acid.
Another classification of barrier membranes includes the first generation of membranes (non-absorbable), second generation (absorbable) and third generation (membranes as a product of tissue engineering)
[14].
2. Trends in the Development of Barrier Membranes
2.1. Amniotic and Chorionic Membranes
Amniotic and chorionic membranes are biological membranes, which means that they are bio-absorbable and compatible with tissues. The human placenta is essential in the development and survival of the fetus, ensuring physical and biological protection
[15]. The amniotic membrane consists of a thick basal membrane and an avascular stromal matrix; this is the innermost layer of the placenta. Chorion forms the outer end of the sac and is made up of several types of collagen and bioactive components of cell adhesion
[16]. Amniotic and chorionic membranes have been used in transplant surgery, proving healing, anti-inflammatory and antibacterial properties
[17].
Membrane harvesting is usually done from healthy pregnant patients; caesarean section is preferred because vaginal birth placentas may be contaminated
[15]. After harvesting, the collected placenta is placed in a sterile transport medium; to obtain the amniotic membrane, the amnion is separated from the underlying chorion along their natural plane of cleavage. Subsequently, the amniotic membrane is abundantly irrigated with a saline solution containing streptomycin, penicillin, neomycin and amphotericin prior to storage
[17]. Methods of preserving the amniotic membrane include cryopreservation, lyophilization or air drying. Cryopreservation results in an improved retention of proteins and growth factors compared to lyophilization
[18], but cryopreservation can affect the viability of cells in the amniotic membrane
[19]. After lyophilization or air drying, it is necessary to sterilize the membrane with the help of gamma radiation
[20] or with peracetic acid
[21].
The amniotic membrane contains type IV, V and VI collagen as well as proteins (fibronectin, laminin, proteoglycans, glycosaminoglycans)
[22]. The amniotic membrane contains laminin 5, a protein that stimulates the cell adhesion of gingival epithelial cells, collagen types I, II, IV, V and VI, platelet-derived growth factor, fibroblast growth factor and TGF-β
[23]. Perlecan found in the amniotic membrane plays an important role in binding growth factors and interacts with various cell adhesion molecules
[24].
Histologically, the chorionic membrane consists of three layers: reticulate, basal membrane and trophoblastic. Collagen types I, III, IV, V, VI and VII, as well as proteoglycans are found in the crosslinked layer; the basal membrane contains type IV collagen, fibronectin and laminin
[25]. Inhibitors of matrix metalloproteinases have been identified in the chorion, factors that can inhibit inflammatory status and stop collagen degradation
[26].
Given these aspects, amniotic and chorion structures offer potential for use as barrier membranes in GTR and GBR. Such membranes have antibacterial and antifungal properties, minimize wound inflammation and provide a protein-rich matrix that allows cells to migrate more easily
[27]. Moreover, the harvesting technique is relatively simple; by hydration with the blood, the membrane becomes malleable
[16]. Major limitations include the risk of cross-contamination, as well as their fragility
[25].
Amniotic and chorionic membranes have been shown to be effective in bone neo-formation in periodontal defects, on canine
[28] and human
[29] subjects. Venkatesan et al. used an amniotic membrane in combination with an alloplastic biphasic bone substitute (60% hydroxyapatite, 40% tricalcium phosphate) to treat infrabony defects; this membrane generated similar results to porcine-derived collagen membrane in terms of postoperative healing and the amount of newly formed bone
[29]. Holtzclaw et al.
[30] used an amnio-chorionic membrane to treat infrabony defects, observing significant improvements of clinical parameters (probing depth and loss of periodontal clinical attachment) at 12 months
[30]. Another study compared the effects of lyophilized amniotic membrane and collagen membrane on newly formed bone density; the bone density of defects treated with amniotic membrane was higher than the sites where no barrier membrane was used and equivalent to the density obtained with collagen membrane (
p < 0.05) at 3 weeks
[31].
The amniotic membrane has also been used successfully in periodontal muco-gingival surgery; the association of the amniotic membrane with repositioned flaps has generated favorable results in covering the gingival recessions and in increasing the thickness of the attached gingiva
[32][33].
An amniotic membrane with different potential areas of use was patented (US6326019B1)
[34], but its usage was directed mainly in skin and mucosal grafting. Actishield™ (Wright Medical, Memphis, TN, USA) was also developed, but with main indication for orthopedic surgery. Up to date, there is no approved commercial amniotic/chorionic membrane for periodontal tissue regeneration. Nevertheless, in all types of membranes, Quality by Design principles and regulations have been introduced in order to obtain benefits of an integrated and risk-free approach to the industrialization process in membranes manufacturing
[35].
2.2. Barrier Membranes from PRF
Platelet concentrates have been a research topic for more than 20 years; platelet-rich plasma, discovered in the late 1990s, was the result of centrifugation of blood harvested on the spot from the patient, offering good advantages in oral and maxillofacial surgery
[36]. This procedure, on the other hand, had disadvantages related to the use of anticoagulants that could interfere with the healing process. Subsequently, a new product without anticoagulant was developed, platelet-rich fibrin (PRF). PRF has been shown to significantly increase the potential for tissue regeneration, favoring the slow and gradual release of growth factors trapped in its fibrin matrix
[37].
PRP has been used primarily for soft tissue regeneration rather than osteogenesis and requires more blood than PRF. PRP is centrifuged at a higher rate, causing all heavy white blood cells and stem cells to sink to the base of the tube, not being collected in the sample
[38]. Further research has found that a higher platelet concentration with the inclusion of white blood cells and stem cells in the sample would be even more therapeutic. The inclusion of white blood cells helped prevent postoperative infection, and the presence of stem cells generated an obvious capacity for regeneration. PRF is centrifuged at a slower rate, which causes a higher concentration of white blood cells, stem cells and platelets to remain in the middle plasma layer. Thus, PRF has a platelet concentration almost double that of blood. Platelets are involved in the release of growth and clotting factors. If the release of the growth factor occurs rapidly in the case of PRP, it is slow for PRF, with an average duration of 7–10 days postoperatively
[39].
PRF has also proven effective in treating gingival recessions. Anilkumar et al.
[40] compared PRF and connective tissue grafting with lateral repositioned flap to cover Miller class II recessions. Recession coverage was complete for 91% of patients treated with PRF, compared with 66% for those treated with a connective graft
[40]. Jankovic et al.
[41], in a treatment of Miller class I or II recessions, compared the results of the use of PRF membranes and connective tissue graft, combined with coronally repositioned flaps and connective tissue graft. The parameters studied were the size of the recession, attachment gain, the height of the keratinized gingiva, the pocket depth, the quality and speed of wound healing and the patient’s discomfort; clinical parameters were evaluated at baseline and after 12 months. The results were similar between the use of a PRF membrane and a connective graft. The authors noted that although the amount of acquired keratinized tissue is higher with the connective graft, PRF provided better healing and less discomfort to the patient
[41]. Thus, PRF may be an interesting, effective and relatively low-cost option, but further studies are needed on larger study groups in order to establish accurate protocols.
2.3. 3D Printed Membranes
Three-dimensional printing aims to generate an individualized 3D object, according to a design developed by software (computer aided design—CAD), by depositing the chosen material layer by layer. Three-dimensional technology already has applications in various fields, such as the production of anatomical models and surgical guides or regenerative medicine. There are multiple methods of 3D printing, including fusion deposition modeling (FDM), stereolithography (SLA) or selective laser sintering (SLS), but FDM remains the technique of choice for bioprinting
[42]. The biomaterials used include hydrogels in combination with living cells and/or growth factors, natural and synthetic bioplastics, proteins, polymer biomolecules and ceramics
[43].
Natural biomaterials used in 3D printing techniques include collagen, agarose, alginate, chitosan, silk, gelatin, cellulose, hyaluronic acid and fibrin
[44], and synthetic biomaterials include polylactic acid (PLA), polyglycolic acid (PGA), poly-lactic-co-glycolic acid (PLGA) and polycaprolactone (PCL)
[45][46]. Decellularized matrix components containing both conserved cellular elements and specific signaling factors of high importance in regenerative processes can also be used, because the latter can guide the cells of the resident tissue or provide the host cells with the necessary instructions for tissue regeneration
[47].
Tayebi et al. tested a 3D-printed membrane of sodium elastin/gelatin/hyaluronate in vitro; the membrane has shown good mechanical properties as well as a stimulation of fibroblast proliferation
[10]. Bai et al.
[48] developed an individualized membrane with 3D-printed titanium mesh; various types of titanium mesh designed with different diameters, and thicknesses were tested based on their mechanical strength by a three-point bending test and FEA. According to the authors, the mechanical properties of the titanium mesh increased when the thickness decreased (0.5 mm to 0.3 mm); by increasing mesh diameter (3 mm to 5 mm), the mechanical properties of the mesh decreased
[48].
A time dimension was added to 3D technology, with the appearance of being 4D; 3D printed construction thus changes, resulting in a transition in shape, structure and function
[49]. This technology is based on intelligent biomaterials that have the ability to undergo changes as a result of exposure to various stimuli (temperature, pH, humidity, electric or magnetic fields, light, sound or a combination thereof)
[50]. Thus, 4D bioprinting offers new directions for the development of tissue reconstruction techniques.
3. Allergological Considerations
The most common allergic reactions to various implants, reported in the literature, were with nickel, cobalt and even titanium, although the latter has a high biocompatibility
[51]. The titanium test was patch-type and was conducted later and not before the implant. Authors who tried a lymphoblastic transformation test (LLT) are cited, but did not have success. However, these reactions are very rare
[52].
The implantation of biomembranes can give two types of reactions: type I reactions (non IgE dependent) and IV (delayed type). A type I immune-allergic reaction is manifested by immediate angioedema and type IV by late reactions, which occur after a few days, even weeks, from the implant
[53].
Regarding biological biomembranes (platelet concentrate, amniotic and chorionic concentrate) the high risk is rejection, as well as type IV reactions, such as contact dermatitis or mucositis, due to the additives with which they are impregnated, and which have been previously described
[54].
Prophylactic testing would not be indicated, as it has no real clinical predictive benefit. Especially in the case of titanium, the time of penetration into the tissues is long. If the patient develops an immediate allergic reaction, which occurs in a few minutes, it would require a prior sensitization of the patient so that he already has cellular memory, with a specific IgE dosage, such as the situation of another implant, and not necessarily in the dental field or for the patient to work in an industry that uses titanium or other metals invoked in biomembranes. Type IV reactions would occur within weeks or even 2 months after membrane insertion.
The only test that would be eloquent is the patch test (with reading at 30 min, 1 h, 24 h, 72 h and 7 days), but only if after the placement of these membranes the suspicion of an allergic reaction arises. Additionally, if the reaction is not severe, such as anaphylactic shock, difficult to control generalized urticaria, or a Stevens Johnson-type reaction, it would be worthwhile to treat the reaction and keep the membrane in place, even if the test is positive.
What is the profile of a risk patient? This is best illustrated by the patient who has had an implant, such as knee, hip, etc., the patient who works in industries that handle these materials and may associate contact dermatitis and, practically, a patient who could have been professionally sensitized prior to a dental implant.
Would immunosuppressive treatment be justified or not, in the case of these delayed type allergic reaction implants? This might depend on the severity of the periodontal disease, the severity of the rejection reaction and the patient’s choice (since these implants are not cheap and the immunosuppressive therapy might not be subsidized by the state in this situation).