B plays an essential role in several symbioses between plants or animals and bacteria, including the following:
All these data presented above show that, differing from inorganic B species, B organic species mainly appear to be essential for the symbiosis among organisms from different kingdoms.
3. Is AI-2B an Essential Quorum Sensing in the Symbiosis between Microbiota and Hosts?
QS is described as a bacterial communication activity that determines competence, bioluminescence, sporulation, antibiotic production, virulence factor secretion, and biofilm evolution in bacteria [83]. A recent study proved that the breadth of QS includes interkingdom communication, as well as being mediated by many newly determined extracellular signaling molecules called autoinducers (AIs) [84].
To date, two types of AI-2 structures have been reported: (2S,4S)-2-methyl-2,3,3,4-tetrahydroxytetrahydrofuranborate (S-THMF-borate, AI-2B) in and (2R,4S)-2-methyl-2,3,3,4-tetrahydroxytetrahydrofuran (R-THMF, AI-2) in Salmonella typhimurium [62,85]. AI-2 has been suggested to promote communication between species of bacteria in the mammalian gut [86]. AI-2 also plays an essential role in probiotic functionality and intestinal colonization [87], and is also correlated with gut dysbiosis [88,89]. The AI-2 concentration is correlated with gut microbial disorders, so AI-2 can be considered a new biomarker for dysbiosis [90].
AI-2B has a recognized role in bacterial communication (intra- and inter-species). Recent findings show that cross-communication between kingdoms (i.e., bacteria and eukaryotic cells) takes place via the bacterium AI-2B quorum detection system [91]. The limit of detection for AI-2B can range from 35 nM to 0.4 μM. A larger concentration of AI-2B could not cause luminescence; subsequently, BA hinders AI-2B signaling [85,92]. Nevertheless, at an AI-2B level of 70 μM, luminescence restriction occurred. As shown, AI-2B may modify the constitution of the microbe population from the gut, improve dysbiosis caused by antibiotics and develop a healthy microbiota [93].
There is evidence that B is essential for the development of certain types of bacteria, such as heterocysts of cyanobacteria and actinomycetes of the genus Frankia [94]. Additionally, many other bacteria are tolerant to large amounts of B, although its essentiality has not yet been demonstrated for them. For example, 15 strains of bacteria (Algoriphagus, Arthrobacter, Bacillus, Gracilibacillus, Lysinibacillus, and Rhodococcus taxa) were isolated and then shown to have tolerance to high concentrations of B [95,96].
In symbioses across kingdoms, bacteria use the ability of B to attach to glycoproteins, thus blocking the bacterium from infecting the host of the symbiosis. In addition, the discovery of bacteria containing one B-signaling molecule (an AI-2 self-inducer, identified as furanosyl borate diester) revealed a surprising role for B in detecting the bacterial quorum. AI-2B is a new signaling molecule that serves as a universal bacterial signal for communication between species and between kingdoms [97].
IM is distributed across the whole GI tract in a heterogeneous way. Collectively, in humans, it is an ecosystem typically weighing 1.5 kg, being formed of more than 1500 bacteria and more than 1000 other species (for instance, viruses, fungi, parasites, phages, and archaebacteria) [98,99]. The most important bacterial phyla of a healthy IM are Firmicutes and Bacteroidetes, followed by Fusobacteria, Actinobacteria, and Proteobacteria. Since the most important species are Faecalibacterium, Bacteroides, and Bifidobacterium, IM achieves several functions, such as maintaining metabolic homeostasis, nutrient absorption, defense against infections, and the development of mucosa and systemic immunity. AI-2B could influence commensal bacterial behaviors to maintain the balance between Bacteroidetes and Firmicutes species. AI-2B is generated by multiple bacterial phyla found in the GI tract, such as Bacteroides spp., Eubacterium rectale, Ruminococcus spp., and Lactobacillus spp. [48,100].
These remarks support the hypothesis that AI-2B is an essential signaling molecule that might regulate bacteria, community dynamics, and behavior in the microbiota, and could also modulate the composition of the microbiota under dysbiosis conditions. AI-2B production by one phylum may affect the expression of genes of other species and can promote communication between species, allowing bacteria to change their behavior, namely their virulence, luminescence, and biofilm formation, between different species [65,101,102]. This feature makes AI-2B a great candidate for modulating interactions between cells in mammalian intestines, where thousands of bacterial phyla coexist and communicate [103]. An example of the protective role of commensal microbes against pathogenic bacteria is as follows: AI-2B produced from Ruminococcus obeum can confuse Vibrio cholerae, resulting in the premature repression of AI-2B QS-mediated virulence and decreased colonization in the intestine [104].
4. Are NOB Species Essential for Healthy Human/Animal Microbiome Symbiosis?
Nutritional essentiality was clearly established as a concept approximately 100 years ago. This resulted from the observation that certain pathologies may be stopped by including a specific food in the diet. Physiological essentiality and nutritional essentiality are two different concepts. Physiological essentiality means an indispensable material for life, while nutritional essentiality means an indispensable material in the diet [105]. A substance is usually considered to be nutritionally essential if a substance deficiency from the diet results in biological dysfunction. The higher intake of that substance prevents biological dysfunction or makes it reversible. Below, we attempt to prove the nutritional essentiality of NOB species based on standard criteria of nutritional essentiality [105]:
(i) “substance is necessary in the diet for growth, health and survival”. B species, such as carbohydrate and phenolic acids esters, are needed for healthy symbiosis between human/animal hosts and the microbiota. The literature holds evidence that certain bacteria need B (AI-2B), a B carbohydrate, for communication, and our preliminary data show that the colonic mucus gel layer of rats also contains B (Figure 1). Our recent discovery of the presence of B within the colonic mucus will need to be confirmed by other laboratories. This notwithstanding, glycoproteins containing B are used in all symbiosis processes between kingdoms in which B is involved and in controlling the interaction between the two entities taking part in the symbiosis process [52].
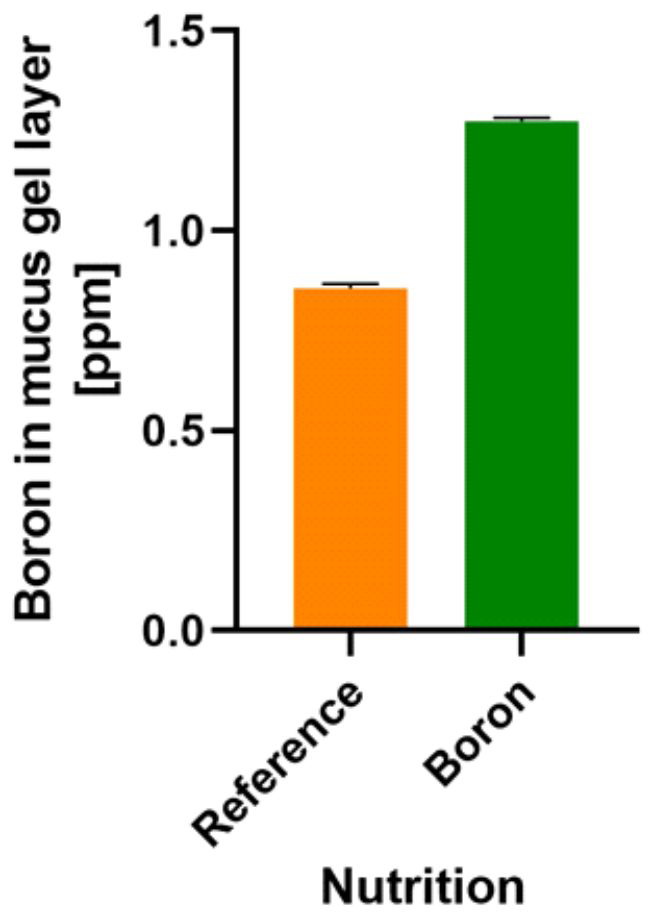
Figure 1. Boron detection in a rat’s colonic mucus gel layer. Reference: normal diet [106]. Boron: CaFB (NOB) supplemented diet. Boron detection was performed by UHPLC/MS [107] (Part S1). CaFB: calcium fructoborate; NOB: naturally organic boron; UHPLC/MS: ultra-high-performance liquid chromatography/mass spectrometry.
(ii) “Its absence from the diet or inadequate intake results in characteristic signs of a deficit disease and, ultimately, death”. The absence of B species from the diet could be a determinant, in our hypothesis, of the permeability of the intestine (“leaky gut syndrome”) and dysbiosis. Dysbiosis and permeability of the intestine cause serious pathologies that lead to death, including inflammation, cancer, heart and brain diseases, arthritis, and bone diseases [108,109]. In most diseases, the composition of the microbiota is altered, causing pathophysiological diseases in vital human organs. The complex interactions between the IM and the host immune system influence the body’s functions, resulting in the formation of an “axis” between the IM and various organs [110]. The “host-microbe” metabolic axis provides systemic multidirectional communication between the cellular pathways of the host and different microbial phyla in the microbiota. Within these axes, various microbes sequentially regulate metabolic reactions, producing bile acids, choline, and SCFAs, which are vital to human/animal health. These metabolites contribute to the metabolic phenotype of the host and the risk of developing the disease [111]. In our view, B deficiency from the symbiosis process may lead to dysbiosis and gut permeability by decreasing the concentration of AI-2B. At the same time, decreasing the concentration of B in the colonic mucus induces the formation of bacterial metabolites and proinflammatory cytokines, which could help the pathophysiological mechanisms of osteoarthritis (OA) [112–114]. This mechanism could explain why in areas with a high content of B, people have a low OA index [115]. In our opinion, in the future, B analysis from the feces and colonic mucus could become an important marker indicating the lack of B from nutrition and a predictive factor for several diseases resulting from unhealthy symbiosis. Subsequently, the commensal bacteria need B as an essential component of their diet for survival, thus proving B’s role in modulating microbiome physiology [116]. Furthermore, the male microbiome is different from the female one [117]. This means that males need a different amount of dietary B than females. Recent studies have shown that there are differences in the level of B in the hair of men and women, with women having almost two times less B in their hair [118]. Thus, women are more susceptible to diseases that are caused by B deficiency in their diet. Subsequently monitoring the AI-2-borate marker in feces will open new scientific horizons for monitoring the prophylaxis of diseases due to a B deficiency in food (i.e., OA, osteoporosis, ovarian cancer, polycystic ovary syndrome, obesity, inflammatory bowel disease, diabetes, fatty liver disease, allergic diseases, and cardiovascular diseases) [109,119,120]. In the future, studies aiming to find the causal relationship among sex, the microbiota, and disease will be crucial. The alteration of the IM from normobiosis to dysbiosis may also be different in women and men. Moreover, different diet-induced changes to the gut microbiota by gender suggest that women and men could differentially benefit from the consumption of a specific B diet, depending on their gender and disease.
(iii) “Growth failure and/or characteristic signs of deficiency are prevented only by the nutrient or a specific precursor of it, not by other substances”. NOB species are prebiotic candidates that may help with bacterial communication via AI-2B [34] and strengthen the mucus gel lining for the human colon (Figure 1). Consequently, the effects of B species deficiency in the microbiota could be: (a) dysbiosis, an alteration in the symbiosis between the human/animal host and the microbiota; in our opinion, this is due to the lack of B, which results in the deficiency of the AI-2B signaling molecule [18,34]; (b) increased intestinal permeability (known as “leaky gut syndrome”) and translocation of the IM from the gut lumen to the systemic circulation due to the lack of B in the structure of the mucin gel. From mucin separation techniques, the interaction of B with glycosylation sites within mucins and O-glycosylated linear glycoproteins is well known [121]. B deficiency thus determines the interaction of the bacterial biofilm directly with the membranes of the host cell and, therefore, the infection of the host. Since 2008, B-stabilized glycoproteins have been claimed to be important for signaling during symbiosis in plants [122]. The description of MoA for B-stabilized glycoproteins has created the probability that identical membrane constituents in animal cells may have a similar role due to B [122]. In all symbiosis processes in which B is essential, this cannot be replaced by any other supporting symbiosis [6].
(iv) “Below some critical level of intake of the nutrient, growth response and/or severity of signs of deficiency are proportional to the amount consumed”. There is a direct correlation between the amount of B species ingested, the amount of B in feces [75,123,124] and the level of B in the gel layer of colonic mucus following NOB-supplemented diet in rats (Figure 1). It is known that in OA, diet modification by increasing the B intake results in diminishing the OA’s severity, correlated with the amount of ingested B [115,125].
(v) “The substance (NOB compounds) is not synthesized in the body and is therefore required to be obtained from the diet for some critical function throughout life”. Humans and other animal cells do not have a metabolic pathway to synthesize organic B compounds, so these must be obtained from plants. Although the World Health Organization (WHO) has still not accepted B as an essential element for humans with a key role in the human metabolism, this may change as our insights are confirmed by other laboratories. Furthermore, confirmation would vindicate Newnham’s 28-year-old recommendation to use B for preventing and managing arthritis and osteoporosis [115].
5. Are NOB Species Novel Prebiotic Candidates?
When discussing B’s essentiality, we must consider not the element itself but the molecular species that incorporate it [18]. A few such essential B species have been detected in bacteria (AI-2B), in fungi (SBEs), and in higher plants (SBEs). Recently, a CBDC chemical form was discovered in green coffee beans [47].
Essentiality should be associated with one specific kind of speciation for the same element. In our view, NOB complexes (e.g., the B-RG-II complex, SBEs, organic polyhydroxy acid borate esters, bis-sucrose borate complexes, amino acid borate esters and, recently, CBDC) may be prebiotic candidates in human/animal nutrition. This is different from BA/borates, which cannot be prebiotic compounds, as these inorganic compounds are digestible and toxic to microorganisms [34]. In general, in the acidic gastric medium, soluble and insoluble organic B species degrade into B monoesters and diesters [18,34]. As natural B monoesters and diesters (polyalcohols, organic acids sugars, and CBDC) have a pKa of between 2.5 and 5.0, many of them do not degrade and therefore remain mainly in the organic form of B. At the same time, because BA can be associated with cis-diol organic compounds (fructose, ribose, glucose, and phenolic acids), in the superior part of the digestive tract, the NOB species is probably reconstituted even at pH 4.5 postprandially [18].
Our 30-year research has attempted to fill this scientific gap by studying the many chemical and biochemical reactions in which B is involved [3,4,18,34,35,41,77,126–134]. Recently, we analyzed some of our old animal studies and found that at the beginning of the B diet, the increase in the B concentration in feces could be explained by NOB species’ indigestibility; then, NOB is released into the colon, where the microbiota needs it [135–138]. We evaluated in vivo the ability of a protein concentrate additive enriched in NOB (as FB) to diminish the toxic effect of a corn-based diet contaminated with Fusarium toxins in piglets. FB has been hypothesized to have stimulated the activity of intestinal microflora, knowing that mycotoxins can disrupt the IM by altering the relative abundance of the commensal bacteria [139,140]. The natural explanation hypothesis for these results was that the human/animal body did not need B, but the IM needed B to create a healthy symbiotic relationship with the host. Subsequently, the MoA of NOB species is as follows: supplying NOB species to bacteria that require B to communicate (AI-2B), and the fortification of the colonic mucus gel layer with organic B esters (this is similar to plant symbiosis, to protect the host from bacterial infection). Consequently, NOB compounds are both a source of B (essential for symbiosis) and a source of carbon for the specific nutrition of microbiota.
Given the above-mentioned MoA, both the microbiota and the gel layer of the colonic mucus become targets for NOB species. Therefore, in the future, NOB species may become promising novel prebiotic candidates. For instance, in the acidic gastric environment, NOB species (such as FB) have a pKa of approximately 4.0, so they do not dissociate in the postprandial upper gastric system (Figure 2; Tables S1 and S2) [34,141–146]. In addition, indirect evidence of B’s presence in the gel layer of colonic mucus is the probable result of B “sequestration” in the colon during B nutrition in animals [75].
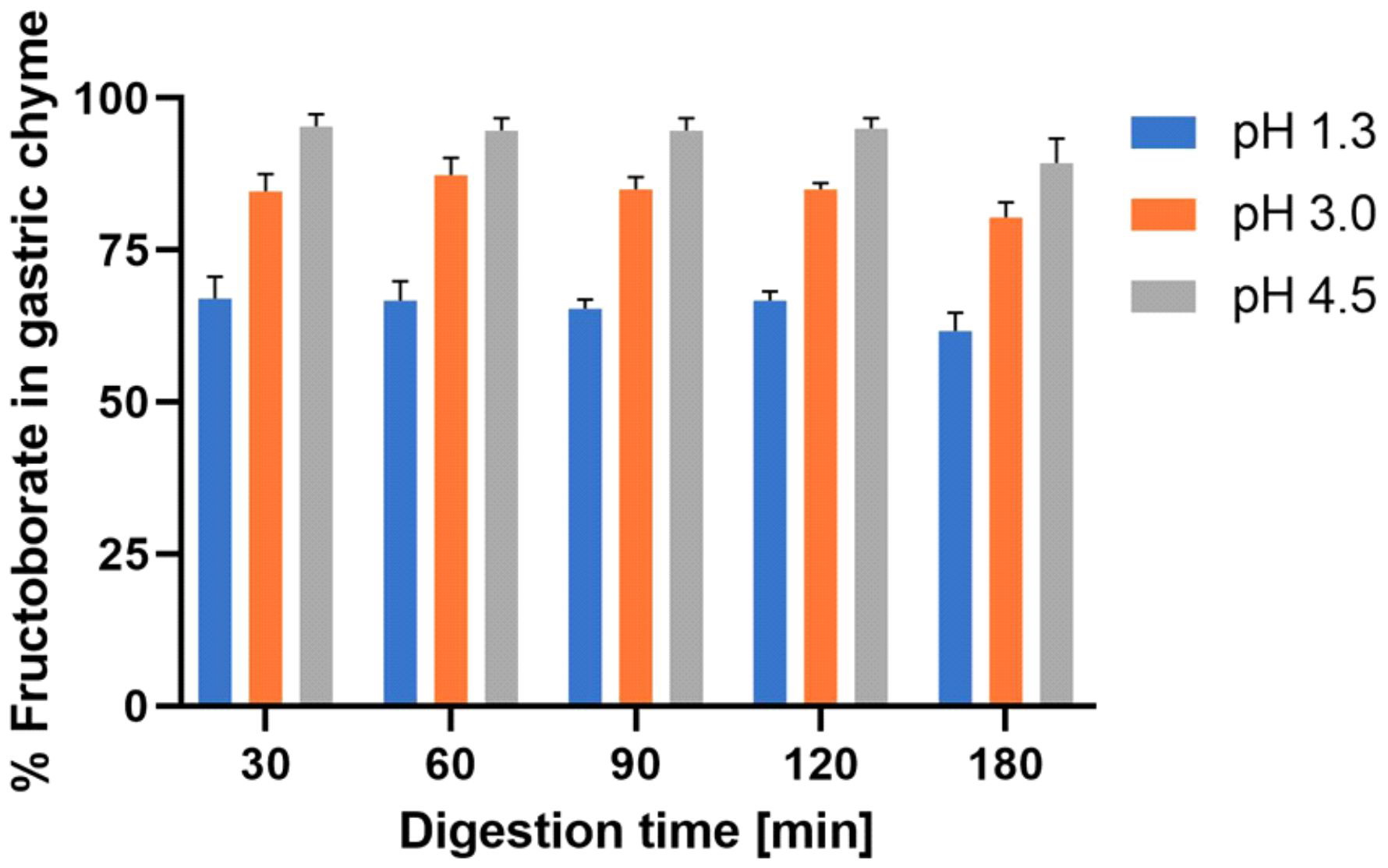
Figure 2. In vitro simulation of gastric digestion of NOB species (Part S2 [142–146]; Tables S1 and S2). NOB: Naturally organic boron.
6. Perspectives
New discoveries about metabolism and molecular nutrition have increased the level of our understanding in the relationship between the colon and human health. As this understanding has improved, the colon has come to be recognized as the optimum location to improve the bioavailability of food due to its distinct features, including its nearly neutral pH, its low enzymatic activity, and its long transit time [147]. Ideally, nutraceuticals should be preserved in the aggressive medium of the superior GI tract and then released into the colon to achieve a successful distribution in the colon and to obtain their full bioavailability [148].
Establishing the essentiality of NOB species for a healthy relationship between microbiota and their human/animal hosts will mark a new approach to the use of these species as prebiotic candidates in human and animal nutrition. The literature explains that the health of humans/animal organisms depends on the health of the microbiota [116,149], supporting the view that the role of NOB species is crucial for the health of the human/animal organism [34]. This role has resulted from many scientific experiments shown that NOB species increase the buffering capacity of saliva [77,81], have a positive impact on the intestinal and oral microbiome [76], protect important probiotic bacteria, namely Bifidobacterium spp. and Lactobacillus spp. [150–152], improve SCFAs production [74], are essential for improving the integrity and impermeability of the intestinal barrier [76], and improve the immunity and anti-inflammatory and antioxidant actions of the microbiota [153]. The microbiota influences the health of the body through the “axes” already formulated and studied in the literature: the “gut-brain” axis, “gut-immunity” axis, “gut-bone” axis, “gut-cartilage” axis, “gut-heart” axis, “gut-lung” axis, and “gut-thyroid” axis [111]. This may explain the beneficial role of B in preventing certain diseases, such as OA [115], osteoporosis [154], rheumatoid arthritis [155], cardiovascular inflammation [18,131,156], depression [157], obesity [158], diabetes [159], viral and bacterial infections [133,160], and thyroid diseases [161].
7. Conclusions
New insights into the essentiality of B for humans and animals suggest that a new hypothesis might be required to understand the role of B in health: “Naturally occurring B species are essential for human/animal host healthy microbiome symbiosis”. This, in turn, will drive new insights into the essentiality of NOB species for healthy symbiosis between the human/animal host and the microbiota, which in turn will guide the use of natural B-based nutraceuticals to target the colon as “colonic foods”. The MoA of NOB species may be primarily related to B molecule signaling (AI-2B), but may also fortify the colonic mucus with B from the specific prebiotic diet.
Subsequently, the key aspects of NOB species’ use in nutrition are:
(i) NOB species are novel and potential prebiotic candidates.
(ii) NOB species are needed for the symbiosis between bacteria and human/animal hosts.
(iii) NOB species can be considered effective novel prebiotics because more than 95% of them reach the colon and do not dissociate in the form of inorganic B (Figure 2; Tables S1 and S2).
(iv) BA, borax and all inorganic B salts are not prebiotics because they are digestible, leading to B dissociation and have shown cytotoxic and genotoxic activity for microbiota [162–164]. The BA is highly available in the bloodstream, while NOB species are indigestible and therefore reach the colon.
(v) NOB species are likely to function as carriers for (a) carbon to support microbiome growth and (b) the essential B element needed for healthy symbiosis.
From a practical point of view, the essentiality of NOB species will open up new opportunities for supplementing B in human/animal nutrition to stay healthy and live long [18,34,82,165]. The dietary intake of NOB molecules could have an important role in the life extension of humans [18]. This is because NOB species intakes have been shown to moderate or alleviate several pathological conditions associated with aging, including cancer, cognitive decline, sarcopenia, and bone health [34]. These findings indicate that a diet rich in B will promote a healthy life expectancy [14,18,34].
New knowledge about the essentiality of NOB species for a healthy symbiosis between human/animal hosts and microbiota will lead to the use of natural B-based nutraceuticals to target the human/animal microbiome (gut, oral, vaginal, skin, and scalp microbiomes). Of these, the gut microbiome is the most important for human health. Subsequently, NOB species have become novel prebiotic candidates and target the colon as novel colonic foods. Moreover, NOB species target colon nutrition, resulting in a healthy gut microbiome, as well as a healthy microbiome in the mouth, vagina, skin, and scalp.
In conclusion, NOB species are novel prebiotic candidates (“a prebiotic is a substrate that is selectively utilized by host microorganisms conferring a health benefit”) [21]; they are indigestible, and they do not dissociate postprandially at pH 4.5 in the upper gastric system (Figure 2). An increasing number of studies show that nutrition may influence gut microbiota and that human health is crucially dependent on the healthy microbiota [166]. Prospective nutrition will be personalized depending on microbiota type for every human being [167]. Thus, NOB species will become essential for personalized nutrition as further novel prebiotic microbiota-accessible candidates. In the future, the colon’s ability to absorb B in the mucus gel layer may be a new target for the B neutron capture therapy (BNCT) of colon cancers by using 10B-enriched prebiotic NOB compounds [138,168,169]. Moreover, supplements containing naturally occurring B should be taken preventively at any time because these supplements ensure the capture and extinction of increased radiation from the living environment. Therefore, these supplements are effective as chemical protection against radiation in conditions of natural radioactivity in soil and water and/or nuclear disasters (nuclear accidents or attacks with nuclear bombs) [170–172]. Recently, the IM and its associated metabolites have been proven to play a major role in high-dose radiation protection [173]. Researchers have noticed that only a group of mice resistant to radiation had two main important classes of bacteria in their gut: Lachnospiraceae and Enterococcaceae. These bacteria need B for the biosynthesis of AI-2B, and the MoA that we proposed for NOB complexes could explain why these complexes ensure absorption of neutrons and gamma-radiation when they reach the colon [138,168,169]. Additionally, in areas where the natural radiation of the soil is increased, the concentration of B in the blood of animals and humans is very low because B in the body is consumed by natural radiation from the environment and transformed into lithium and helium, but only the 10B isotope conducts the reaction [174–176]. In these areas, people have very advanced OA (over 70%) [177–180].
Supplementary Materials
The following supporting information can be downloaded at: https://www.mdpi.com/article/10.3390/ijms23169147/s1.
Author Contributions
Conceptualization, A.B., I.R.S. and T.A.B.; methodology, M.V.C., C.B., A.R., L.E.B. and G.R.; writing—original draft preparation, I.R.S., G.D.M. and J.N.; writing—review and editing, A.B., I.R.S., G.D.M. and S.A.B.; supervision, A.B., I.R.S., G.D.M. and J.N.; funding acquisition, A.B. and I.R.S. All authors have read and agreed to the published version of the manuscript.
Funding
This work was supported by a grant of the Romanian Ministry of Education and Research, CNCS-UEFISCDI, project number PN-III-P1-1.1-PD-2019-0214, within PNCDI III.
Institutional Review Board Statement
The experimental protocol was applied according to the European Council Directive No. 86/609 (24 November 1986), the European Convention for the Protection of Vertebrate Animals used for Experimental and Other Scientific Purposes (2 December 2005), and the Romanian Parliament Law No. 43 (11 April 2014) on the protection of animals used for scientific purposes. The study was approved by the Ethics Committee of the University of Medicine and Pharmacy of Craiova (Approval No. 51/20.04.2018).
Informed Consent Statement
Not applicable.
Acknowledgments
We thank Jennifer Murphy, MS, RD, Director of Innovation and Clinical Development from PLT Health Solutions, Inc., for her kind assistance with the final version of the manuscript.
Conflicts of Interest
The authors declare no conflict of interest.
References
- Ricardo, A.; Carrigan, M.A.; Olcott, A.N.; Benner, S.A. Borate minerals stabilize ribose. Science 2004, 303, 196.
- Scorei, R.; Cimpoiaşu, V.M. Boron enhances the thermostability of carbohydrates. Life Evol. Biosph. 2006, 36, 1–11. https://doi.org/10.1007/s11084-005-0562-1.
- Scorei, R. Is boron a prebiotic element? A mini-review of the essentiality of boron for the appearance of life on Earth. Life Evol. Biosph. 2012, 42, 3–17. https://doi.org/10.1007/s11084-012-9269-2.
- Kim, H.J.; Furukawa, Y.; Kakegawa, T.; Bita, A.; Scorei, R.; Benner, S.A. Evaporite borate-containing mineral ensembles make phosphate available and regiospecifically phosphorylate ribonucleosides: Borate as a multifaceted problem solver in prebiotic chemistry. Chemie Int. Ed. Engl. 2016, 55, 15816–15820. https://doi.org/10.1002/anie.201608001.
- Zumreoglu-Karan, B.; Kose, D.A. Boric acid: A simple molecule of physiologic, therapeutic and prebiotic significance. Pure Appl. Chem. 2015, 87, 155–162. https://doi.org/10.1515/pac-2014-0909.
- Bolaños, L.; Lukaszewski, K.; Bonilla, I.; Blevins, D. Why boron? Plant Physiol. Biochem. 2004, 42, 907–912. https://doi.org/10.1016/j.plaphy.2004.11.002.
- Pereira, G.L.; Siqueira, J.A.; Batista-Silva, W.; Cardoso, F.B.; Nunes-Nesi, A.; Araújo, W.L. Boron: More than an essential element for land plants? Plant Sci. 2021, 11, 610307. https://doi.org/10.3389/fpls.2020.610307.
- Bolanos, L.; Esteban, E.; De Lorenzo, C.; Fernandez-Pascual, M.; De Felipe, M.R.; Garate, A.; Bonilla, I. Essentiality of boron for symbiotic dinitrogen fixation in pea (Pisum sativum) Rhizobium Plant Physiol. 1994, 104, 85–90. https://doi.org/10.1104/pp.104.1.85.
- Raja, C.E.; Omine, K. Characterization of boron resistant and accumulating bacteria Lysinibacillus fusiformis M1, Bacillus cereus M2, Bacillus cereus M3, Bacillus pumilus M4 isolated from former mining site, Hokkaido, Japan. Environ. Sci. Health A Tox. Hazard. Subst. Environ. Eng. 2012, 47, 1341–1349. https://doi.org/10.1080/10934529.2012.672299.
- Estevez-Fregoso, E.; Farfán-García, E.D.; García-Coronel, I.H.; Martínez-Herrera, E.; Alatorre, A.; Scorei, R.I.; Soriano-Ursúa, M.A. Effects of boron-containing compounds in the fungal kingdom. Trace Elem. Med. Biol. 2021, 65, 126714. https://doi.org/10.1016/j.jtemb.2021.126714.
- Hunt, C.D. The biochemical effects of physiologic amounts of dietary boron in animal nutrition models. Health Perspect. 1994, 102, 35–43. https://doi.org/10.1289/ehp.94102s735.
- Nielsen, F.H.; Eckhert, C.D. Boron. Nutr. 2020, 11, 461–462. https://doi.org/10.1093/advances/nmz110.
- Nielsen, F.H. The justification for providing dietary guidance for the nutritional intake of boron. Trace Elem. Res. 1998, 66, 319–330. https://doi.org/10.1007/BF02783145.
- Nielsen, F.H. Is boron nutritionally relevant? Rev. 2008, 66, 183–191. https://doi.org/10.1111/j.1753-4887.2008.00023.x.
- Nielsen, F.H. Update on human health effects of boron. Trace Elem. Med. Biol. 2014, 28, 383–387. https://doi.org/10.1016/j.jtemb.2014.06.023.
- Rowe, R.I.; Bouzan, C.; Nabili, S.; Eckhert, C.D. The response of trout and zebrafish embryos to low and high boron concentrations is U-shaped. Trace Elem. Res. 1998, 66, 261–270. https://doi.org/10.1007/BF02783142.
- Rowe, R.I.; Eckhert, C.D. Boron is required for zebrafish embryogenesis. Exp. Biol. 1999, 202, 1649–1654. https://doi.org/10.1242/jeb.202.12.1649.
- Donoiu, I.; Militaru, C.; Obleagă, O.; Hunter, J.M.; Neamţu, J.; Biţă, A.; Scorei, I.R.; Rogoveanu, O.C. Effects of boron-containing compounds on cardiovascular disease risk factors—a review. Trace Elem. Med. Biol. 2018, 50, 47–56. https://doi.org/10.1016/j.jtemb.2018.06.003.
- Benner, S.A. Paradoxes in the origin of life. Life Evol. Biosph. 2014, 44, 339–343. https://doi.org/10.1007/s11084-014-9379-0.
- Gibson, G.R.; Hutkins, R.; Sanders, M.E.; Prescott, S.L.; Reimer, R.A.; Salminen, S.J.; Scott, K.; Stanton, C.; Swanson, K.S.; Cani, P.D.; et al. Expert consensus document: The International Scientific Association for Probiotics and Prebiotics (ISAPP) consensus statement on the definition and scope of prebiotics. Rev. Gastroenterol. Hepatol. 2017, 14, 491–502. https://doi.org/10.1038/nrgastro.2017.75.
- Swanson, K.S.; Gibson, G.R.; Hutkins, R.; Reimer, R.A.; Reid, G.; Verbeke, K.; Scott, K.P.; Holscher, H.D.; Azad, M.B.; Delzenne, N.M.; et al. The International Scientific Association for Probiotics and Prebiotics (ISAPP) consensus statement on the definition and scope of synbiotics. Rev. Gastroenterol. Hepatol. 2020, 17, 687–701. https://doi.org/10.1038/s41575-020-0344-2.
- Shi, H.; Wang, Q.; Zheng, M.; Hao, S.; Lum, J.S.; Chen, X.; Huang, X.F.; Yu, Y.; Zheng, K. Supplement of microbiota-accessible carbohydrates prevents neuroinflammation and cognitive decline by improving the gut microbiota–brain axis in diet-induced obese mice. Neuroinflammation 2020, 17, 77. https://doi.org/10.1186/s12974-020-01760-1.
- Corrêa, T.A.F.; Rogero, M.M.; Hassimotto, N.M.A.; Lajolo, F.M. The two-way polyphenols–microbiota interactions and their effects on obesity and related metabolic diseases. Nutr. 2019, 6, 188. https://doi.org/10.3389/fnut.2019.00188.
- Mithul Aravind, S.; Wichienchot, S.; Tsao, R.; Ramakrishnan, S.; Chakkaravarthi, S. Role of dietary polyphenols on gut microbiota, their metabolites and health benefits. Food Res. Int. 2021, 142, 110189. https://doi.org/10.1016/j.foodres.2021.110189.
- Costantini, L.; Molinari, R.; Farinon, B.; Merendino, N. Impact of omega-3 fatty acids on the gut microbiota. J. Mol. Sci. 2017, 18, 2645. https://doi.org/10.3390/ijms18122645.
- Zhu, X.; Bi, Z.; Yang, C.; Guo, Y.; Yuan, J.; Li, L.; Guo, Y. Effects of different doses of omega-3 polyunsaturated fatty acids on gut microbiota and immunity. Food Nutr. Res. 2021, 65, 6263. https://doi.org/10.29219/fnr.v65.6263.
- Aslam, H.; Marx, W.; Rocks, T.; Loughman, A.; Chandrasekaran, V.; Ruusunen, A.; Dawson, S.L.; West, M.; Mullarkey, E.; Pasco, J.A.; et al. The effects of dairy and dairy derivatives on the gut microbiota: A systematic literature review. Gut Microbes 2020, 12, 1799533. https://doi.org/10.1080/19490976.2020.1799533.
- Cunningham, M.; Azcarate-Peril, M.A.; Barnard, A.; Benoit, V.; Grimaldi, R.; Guyonnet, D.; Holscher, H.D.; Hunter, K.; Manurung, S.; Obis, D.; et al. Shaping the future of probiotics and prebiotics. Trends Microbiol. 2021, 29, 667–685. https://doi.org/10.1016/j.tim.2021.01.003.
- Wu, H.J.; Wu, E. The role of gut microbiota in immune homeostasis and autoimmunity. Gut Microbes 2012, 3, 4–14. https://doi.org/10.4161/gmic.19320.
- Markowiak, P.; Śliżewska, K. Effects of probiotics, prebiotics, and synbiotics on human health. Nutrients 2017, 9, 1021. https://doi.org/10.3390/nu9091021.
- Peng, M.; Tabashsum, Z.; Anderson, M.; Truong, A.; Houser, A.K.; Padilla, J.; Akmel, A.; Bhatti, J.; Rahaman, S.O.; Biswas, D. Effectiveness of probiotics, prebiotics, and prebiotic-like components in common functional foods. Rev. Food Sci. Food Saf. 2020, 19, 1908–1933. https://doi.org/10.1111/1541-4337.12565.
- Daïen, C.I.; Pinget, G.V.; Tan, J.K.; Macia, L. Detrimental impact of microbiota-accessible carbohydrate-deprived diet on gut and immune homeostasis: An overview. Immunol. 2017, 8, 548. https://doi.org/10.3389/fimmu.2017.00548.
- Gentile, C.L.; Weir, T.L. The gut microbiota at the intersection of diet and human health. Science 2018, 362, 776–780. https://doi.org/10.1126/science.aau5812.
- Hunter, J.M.; Nemzer, B.V.; Rangavajla, N.; Biţă, A.; Rogoveanu, O.C.; Neamţu, J.; Scorei, I.R.; Bejenaru, L.E.; Rău, G.; Bejenaru, C.; et al. The fructoborates: Part of a family of naturally occurring sugar–borate complexes—biochemistry, physiology, and impact on human health: A review. Trace Elem. Res. 2019, 188, 11–25. https://doi.org/10.1007/s12011-018-1550-4.
- Mogoşanu, G.D.; Biţă, A.; Bejenaru, L.E.; Bejenaru, C.; Croitoru, O.; Rău, G.; Rogoveanu, O.C.; Florescu, D.N.; Neamţu, J.; Scorei, I.D.; et al. Calcium fructoborate for bone and cardiovascular health. Trace Elem. Res. 2016, 172, 277–281. https://doi.org/10.1007/s12011-015-0590-2.
- Xia, X.; Chang, J.S.; Hunter, J.M.; Nemzer, B.V. Identification and quantification of fructoborate ester complex using liquid chromatography coupled with Q exactive orbitrap mass spectrometry. Food Res. 2017, 6, 85–92. https://doi.org/10.5539/jfr.v6n3p85.
- Brown, P.H.; Hu, H. Phloem mobility of boron is species dependent: Evidence for phloem mobility in sorbitol-rich species. Bot. 1996, 77, 497–506. https://doi.org/10.1006/anbo.1996.0060.
- Kobayashi, M.; Matoh, T.; Azuma, J. Two chains of rhamnogalacturonan II are cross-linked by borate–diol ester bonds in higher plant cell walls. Plant Physiol. 1996, 110, 1017–1020. https://doi.org/10.1104/pp.110.3.1017.
- Brown, P.H.; Hu, H. Phloem boron mobility in diverse plant species. Acta 1998, 111, 331–335. https://doi.org/10.1111/j.1438-8677.1998.tb00717.x.
- Miljkovic, D.; Scorei, R.I.; Cimpoiaşu, V.M.; Scorei, I.D. Calcium fructoborate: Plant-based dietary boron for human nutrition. Diet. Suppl. 2009, 6, 211–226. https://doi.org/10.1080/19390210903070772.
- Scorei, I.D.; Scorei, R.I. Calcium fructoborate helps control inflammation associated with diminished bone health. Trace Elem. Res. 2013, 155, 315–321. https://doi.org/10.1007/s12011-013-9800-y.
- Dinca, L.; Scorei, R. Boron in human nutrition and its regulations use. Nutr. Ther. 2013, 2, 22–29. https://doi.org/10.6000/1929-5634.2013.02.01.3.
- O’Neill, M.A.; Ishii, T.; Albersheim, P.; Darvill, A.G. Rhamnogalacturonan II: Structure and function of a borate cross-linked cell wall pectic polysaccharide. Rev. Plant Biol. 2004, 55, 109–139. https://doi.org/10.1146/annurev.arplant.55.031903.141750.
- Dembitsky, V.M.; Smoum, R.; Al-Quntar, A.A.; Ali, H.A.; Pergament, I.; Srebnik, M. Natural occurrence of boron-containing compounds in plants, algae and microorganisms. Plant Sci. 2002, 163, 931–942. https://doi.org/10.1016/S0168-9452(02)00174-7.
- Miguez-Pacheco, V.; Hench, L.L.; Boccaccini, A.R. Bioactive glasses beyond bone and teeth: Emerging applications in contact with soft tissues. Acta Biomater. 2015, 13, 1–15. https://doi.org/10.1016/j.actbio.2014.11.004.
- Dembitsky, V.M.; Gloriozova, T.A. Naturally occurring boron containing compounds and their biological activities. Nat. Prod. Resour. 2017, 3, 147–154. Available online: https://www.jacsdirectory.com/journal-of-natural-products-and-resources/articleview.php?id=40 ( April 20, 2022).
- Scorei, R.; Bita, A.; Dinca, L.; Mogosanu, D.; Rangavajla, N. Diester chlorogenoborate complex: Methods for identification, synthesis, purification and uses thereof. United States Patent and Trademark Office (USPTO), Provisional Patent Application No. 63271159/10/24/2021. 2021. Available online: https://www.uspto.gov/patents/basics/types-patent-applications/provisional-application-patent (accessed on April 20, 2022).
- Thompson, J.A.; Oliveira, R.A.; Xavier, K.B. Chemical conversations in the gut microbiota. Gut Microbes 2016, 7, 163–170. https://doi.org/10.1080/19490976.2016.1145374.
- Leidner, D.E. Review and theory symbiosis: An introspective retrospective. Assoc. Inf. Syst. 2018, 19, 552–567. https://doi.org/10.17705/1jais.00501.
- Nelson, P.G.; May, G. Coevolution between mutualists and parasites in symbiotic communities may lead to the evolution of lower virulence. Nat. 2017, 190, 803–817. https://doi.org/10.1086/694334.
- González, J.E.; Marketon, M.M. Quorum sensing in nitrogen-fixing rhizobia. Mol. Biol. Rev. 2003, 67, 574–592. https://doi.org/10.1128/MMBR.67.4.574-592.2003.
- Abreu, I.; Cerda, M.E.; de Nanclares, M.P.; Baena, I.; Lloret, J.; Bonilla, I.; Bolaños, L.; Reguera, M. Boron deficiency affects rhizobia cell surface polysaccharides important for suppression of plant defense mechanisms during legume recognition and for development of nitrogen-fixing symbiosis. Plant Soil 2012, 361, 385–395. https://doi.org/10.1007/s11104-012-1229-0.
- Bolaños, L.; Redondo-Nieto, M.; Bonilla, I.; Wall, L.G. Boron requirement in the Discaria trinervis (Rhamnaceae) and Frankia symbiotic relationship. Its essentiality for Frankia BCU110501 growth and nitrogen fixation. Plant. 2002, 115, 563–570. https://doi.org/10.1034/j.1399-3054.2002.1150410.x.
- Fournier, J.; Timmers, A.C.J.; Sieberer, B.J.; Jauneau, A.; Chabaud, M.; Barker, D.G. Mechanism of infection thread elongation in root hairs of Medicago truncatula and dynamic interplay with associated rhizobial colonization. Plant Physiol. 2008, 148, 1985–1995. https://doi.org/10.1104/pp.108.125674.
- Sonmez, O.; Aydemir, S.; Kaya, C. Mitigation effects of mycorrhiza on boron toxicity in wheat (Triticum durum) plants. Z. J. Crop. Hortic. Sci. 2009, 37, 99–104. https://doi.org/10.1080/01140670909510254.
- Begum, N.; Qin, C.; Ahanger, M.A.; Raza, S.; Khan, M.I.; Ashraf, M.; Ahmed, N.; Zhang, L. Role of arbuscular mycorrhizal fungi in plant growth regulation: Implications in abiotic stress tolerance. Plant Sci. 2019, 10, 1068. https://doi.org/10.3389/fpls.2019.01068.
- Hua, T.; Zhang, R.; Sun, H.; Liu, C. Alleviation of boron toxicity in plants: Mechanisms and approaches. Rev. Environ. Sci. Technol. 2021, 51, 2975–3015. https://doi.org/10.1080/10643389.2020.1807451.
- Simón-Grao, S.; Nieves, M.; Martínez-Nicolás, J.J.; Alfosea-Simón, M.; Cámara-Zapata, J.M.; Fernández-Zapata, J.C.; García-Sánchez, F. Arbuscular mycorrhizal symbiosis improves tolerance of Carrizo citrange to excess boron supply by reducing leaf B concentration and toxicity in the leaves and roots. Environ. Saf. 2019, 173, 322–330. https://doi.org/10.1016/j.ecoenv.2019.02.030.
- Quiroga, G.; Erice, G.; Aroca, R.; Ruiz-Lozano, J.M. Elucidating the possible involvement of maize aquaporins in the plant boron transport and homeostasis mediated by Rhizophagus irregularis under drought stress conditions. J. Mol. Sci. 2020, 21, 1748. https://doi.org/10.3390/ijms21051748.
- Bonilla, I.; Garcia-González, M.; Mateo, P. Boron requirement in cyanobacteria: Its possible role in the early evolution of photosynthetic organisms. Plant Physiol. 1990, 94, 1554–1560. https://doi.org/10.1104/pp.94.4.1554.
- Garcia-Gonzalez, M.; Mateo, P.; Bonilla, I. Boron requirement for envelope structure and function in Anabaena PCC 7119 heterocysts. Exp. Bot. 1991, 42, 925–929. https://doi.org/10.1093/jxb/42.7.925.
- Chen, X.; Schauder, S.; Potier, N.; Van Dorsselaer, A.; Pelczer, I.; Bassler, B.L.; Hughson, F.M. Structural identification of a bacterial quorum-sensing signal containing boron. Nature 2002, 415, 545–549. https://doi.org/10.1038/415545a.
- Bonilla, I.; Redondo-Nieto, M.; El-Hamdaoui, A.; Wall, L.G.; Bolaños, L. Essentiality of boron for symbiotic nitrogen fixation in legumes and actinorhizal plants. In Boron in Plant and Animal Nutrition; Goldbach, H.E., Brown, P.H., Rerkasem, B., Thellier, M., Wimmer, M.A., Bell, R.W., Eds.; Springer: Boston, MA, USA, 2002; pp. 261–267. https://doi.org/10.1007/978-1-4615-0607-2_24.
- Harris, W.R.; Amin, S.A.; Küpper, F.C.; Green, D.H.; Carrano, C.J. Borate binding to siderophores: Structure and stability. Am. Chem. Soc. 2007, 129, 12263–12271. https://doi.org/10.1021/ja073788v.
- Cuadra, G.A.; Frantellizzi, A.J.; Gaesser, K.M.; Tammariello, S.P.; Ahmed, A. Autoinducer-2 detection among commensal oral streptococci is dependent on pH and boric acid. Microbiol. 2016, 54, 492–502. https://doi.org/10.1007/s12275-016-5507-z.
- Kaper, J.B.; Sperandio, V. Bacterial cell-to-cell signaling in the gastrointestinal tract. Immun. 2005, 73, 3197–3209. https://doi.org/10.1128/IAI.73.6.3197-3209.2005.
- Pereira, C.S.; Thompson, J.A.; Xavier, K.B. AI-2-mediated signalling in bacteria. FEMS Microbiol. Rev. 2013, 37, 156–181. https://doi.org/10.1111/j.1574-6976.2012.00345.x.
- Jiang, M.; Dong, F.Y.; Pan, X.Y.; Zhang, Y.N.; Zhang, F. Boric acid was orally toxic to different instars of Blattella germanica (L.) (Blattodea: Blattellidae) and caused dysbiosis of the gut microbiota. Biochem. Physiol. 2021, 172, 104756. https://doi.org/10.1016/j.pestbp.2020.104756.
- Bourgeois, A.C.; Scott, M.E.; Sabally, K.; Koski, K.G. Low dietary boron reduces parasite (Nematoda) survival and alters cytokine profiles but the infection modifies liver minerals in mice. Nutr. 2007, 137, 2080–2086. https://doi.org/10.1093/jn/137.9.2080.
- Fort, D.J.; Rogers, R.L.; McLaughlin, D.W.; Sellers, C.M.; Schlekat, C.L. Impact of boron deficiency on Xenopus laevis: A summary of biological effects and potential biochemical roles. Trace Elem. Res. 2002, 90, 117–142. https://doi.org/10.1385/BTER:90:1-3:117.
- Evariste, L.; Flahaut, E.; Baratange, C.; Barret, M.; Mouchet, F.; Pinelli, E.; Galibert, A.M.; Soula, B.; Gauthier, L. Ecotoxicological assessment of commercial boron nitride nanotubes toward Xenopus laevis tadpoles and host-associated gut microbiota. Nanotoxicology 2021, 15, 35–51. https://doi.org/10.1080/17435390.2020.1839137.
- Elshahawi, S.I.; Trindade-Silva, A.E.; Hanora, A.; Han, A.W.; Flores, M.S.; Vizzoni, V.; Schrago, C.G.; Soares, C.A.; Concepcion, G.P.; Distel, D.L.; et al. Boronated tartrolon antibiotic produced by symbiotic cellulose-degrading bacteria in shipworm gills. Natl. Acad. Sci. USA 2013, 110, E295–E304. https://doi.org/10.1073/pnas.1213892110.
- Hernandez-Patlan, D.; Solis-Cruz, B.; Adhikari, B.; Pontin, K.P.; Latorre, J.D.; Baxter, M.F.A.; Hernandez-Velasco, X.; Merino-Guzman, R.; Méndez-Albores, A.; Kwon, Y.M.; et al. Evaluation of the antimicrobial and intestinal integrity properties of boric acid in broiler chickens infected with Salmonella enteritidis: Proof of concept. Vet. Sci. 2019, 123, 7–13. https://doi.org/10.1016/j.rvsc.2018.12.004.
- Sizmaz, O.; Koksal, B.H.; Yildiz, G. Rumen microbial fermentation, protozoan abundance and boron availability in yearling rams fed diets with different boron concentrations. Anim. Feed Sci. 2017, 26, 59–64. https://doi.org/10.22358/jafs/69038/2017.
- Miyamoto, S.; Sutoh, M.; Shiomoto, A.; Yamazaki, S.; Nishimura, K.; Yonezawa, C.; Matsue, H.; Hoshi, M. Determination of boron in animal materials by reactor neutron induced prompt gamma-ray analysis. Radioanal. Nucl. Chem. 2000, 244, 307–309. https://doi.org/10.1023/a:1006750617838.
- Sun, P.P.; Luo, Y.; Wu, X.T.; Ansari, A.R.; Wang, J.; Yang, K.L.; Xiao, K.; Peng, K.M. Effects of supplemental boron on intestinal proliferation and apoptosis in African ostrich chicks (Efectos del boro suplementario sobre la proliferación intestinal y apoptosis en polluelos de avestruz africana). J. Morphol. 2016, 34, 830–835. https://doi.org/10.4067/S0717-95022016000300002.
- Mitruţ, I.; Cojocaru, M.O.; Scorei, I.R.; Biţă, A.; Mogoşanu, G.D.; Popescu, M.; Olimid, D.A.; Manolea, H.O. Preclinical and histological study of boron-containing compounds hydrogels on experimental model of periodontal disease. J. Morphol. Embryol. 2021, 62, 219–226. https://doi.org/10.47162/RJME.62.1.21.
- Kuru, R.; Balan, G.; Yilmaz, S.; Taslı, P.N.; Akyuz, S.; Yarat, A.; Sahin, F. The level of two trace elements in carious, non-carious, primary, and permanent teeth. Oral Res. 2020, 54, 77–80. https://doi.org/10.26650/eor.20200072.
- Cho, H.M.; Macelline, S.P.; Wickramasuriya, S.S.; Shin, T.K.; Kim, E.; Son, H.C.; Heo, J.M. Moderate dietary boron supplementation improved growth performance, crude protein digestibility and diarrhea index in weaner pigs regardless to the sanitary condition. Biosci. 2022, 35, 434–443. https://doi.org/10.5713/ab.21.0110.
- Bargiel, P.; Szczuko, M.; Stachowska, L.; Prowans, P.; Czapla, N.; Markowska, M.; Petriczko, J.; Kledzik, J.; Jędrzejczyk-Kledzik, A.; Palma, J.; et al. Microbiome metabolites and thyroid dysfunction. Clin. Med. 2021, 10, 3609. https://doi.org/10.3390/jcm10163609.
- Kuru, R.; Yilmaz, S.; Balan, G.; Tuzuner, B.A.; Tasli, P.N.; Akyuz, S.; Yener Ozturk, F.; Altuntas, Y.; Yarat, A.; Sahin, F. Boron-rich diet may regulate blood lipid profile and prevent obesity: A nondrug and self-controlled clinical trial. Trace Elem. Med. Biol. 2019, 54, 191–198. https://doi.org/10.1016/j.jtemb.2019.04.021.
- Weber, K.S.; Ratjen, I.; Enderle, J.; Seidel, U.; Rimbach, G.; Lieb, W. Plasma boron concentrations in the general population: A cross-sectional analysis of cardio-metabolic and dietary correlates. J. Nutr. 2022, 61, 1363–1375. https://doi.org/10.1007/s00394-021-02730-w.
- Nealson, K.H.; Hastings, J.W. Quorum sensing on a global scale: Massive numbers of bioluminescent bacteria make milky seas. Environ. Microbiol. 2006, 72, 2295–2297. https://doi.org/10.1128/AEM.72.4.2295-2297.2006.
- Wu, L.; Luo, Y. Bacterial quorum-sensing systems and their role in intestinal bacteria–host crosstalk. Microbiol. 2021, 12, 611413. https://doi.org/10.3389/fmicb.2021.611413.
- Miller, S.T.; Xavier, K.B.; Campagna, S.R.; Taga, M.E.; Semmelhack, M.F.; Bassler, B.L.; Hughson, F.M. Salmonella typhimurium recognizes a chemically distinct form of the bacterial quorum-sensing signal AI-2. Cell 2004, 15, 677–687. https://doi.org/10.1016/j.molcel.2004.07.020.
- Ismail, A.S.; Valastyan, J.S.; Bassler, B.L. A host-produced autoinducer-2 mimic activates bacterial quorum sensing. Cell Host Microbe 2016, 19, 470–480. https://doi.org/10.1016/j.chom.2016.02.020.
- Christiaen, S.E.A.; O’Connell Motherway, M.; Bottacini, F.; Lanigan, N.; Casey, P.G.; Huys, G.; Nelis, H.J.; van Sinderen, D.; Coenye, T. Autoinducer-2 plays a crucial role in gut colonization and probiotic functionality of Bifidobacterium breve PLoS ONE 2014, 9, e98111. https://doi.org/10.1371/journal.pone.0098111.
- Bivar Xavier, K. Bacterial interspecies quorum sensing in the mammalian gut microbiota. R. Biol. 2018, 341, 297–299. https://doi.org/10.1016/j.crvi.2018.03.006.
- Zhao, T.S.; Xie, L.W.; Cai, S.; Xu, J.Y.; Zhou, H.; Tang, L.F.; Yang, C.; Fang, S.; Li, M.; Tian, Y. Dysbiosis of gut microbiota is associated with the progression of radiation-induced intestinal injury and is alleviated by oral compound probiotics in mouse model. Cell. Infect. Microbiol. 2021, 11, 717636. https://doi.org/10.3389/fcimb.2021.717636.
- Fu, C.Y.; Li, L.Q.; Yang, T.; She, X.; Ai, Q.; Wang, Z.L. Autoinducer-2 may be a new biomarker for monitoring neonatal necrotizing enterocolitis. Cell. Infect. Microbiol. 2020, 10, 140. https://doi.org/10.3389/fcimb.2020.00140.
- Loomis, W.D.; Durst, R.W. Chemistry and biology of boron. Biofactors 1992, 3, 229–239. Available online: https://pubmed.ncbi.nlm.nih.gov/1605832/ (accessed on April 20, 2022).
- Ascenso, O.S.; Marques, J.C.; Santos, A.R.; Xavier, K.B.; Ventura, M.R.; Maycock, C.D. An efficient synthesis of the precursor of AI-2, the signalling molecule for inter-species quorum sensing. Med. Chem. 2011, 19, 1236–1241. https://doi.org/10.1016/j.bmc.2010.12.036.
- Sun, Z.; Grimm, V.; Riedel, C.U. AI-2 to the rescue against antibiotic-induced intestinal dysbiosis? Trends Microbiol. 2015, 23, 327–328. https://doi.org/10.1016/j.tim.2015.04.002.
- Pesce, C.; Oshone, R.; Hurst, S.G., 4th; Kleiner, V.A.; Tisa, L.S. Stable transformation of the actinobacteria Frankia Appl. Environ. Microbiol. 2016, 85, e00957-19. https://doi.org/10.1128/AEM.00957-19.
- Ahmed, I.; Fujiwara, T. Mechanism of boron tolerance in soil bacteria. J. Microbiol. 2010, 56, 22–26. https://doi.org/10.1139/W09-106.
- Ahmed, I.; Yokota, A.; Fujiwara, T. A novel highly boron tolerant bacterium, Bacillus boroniphilus nov., isolated from soil, that requires boron for its growth. Extremophiles 2007, 11, 217–224. https://doi.org/10.1007/s00792-006-0027-0.
- Atkinson, S.; Williams, P. Quorum sensing and social networking in the microbial world. R. Soc. Interface 2009, 6, 959–978. https://doi.org/10.1098/rsif.2009.0203.
- Matijašić, M.; Meštrović, T.; Paljetak, H.Č.; Perić, M.; Barešić, A.; Verbanac, D. Gut microbiota beyond bacteria—mycobiome, virome, archaeome, and eukaryotic parasites in IBD. J. Mol. Sci. 2020, 21, 2668. https://doi.org/10.3390/ijms21082668.
- Vemuri, R.; Shankar, E.M.; Chieppa, M.; Eri, R.; Kavanagh, K. Beyond just bacteria: Functional biomes in the gut ecosystem including virome, mycobiome, archaeome and helminths. Microorganisms 2020, 8, 483. https://doi.org/10.3390/microorganisms8040483.
- Engevik, M.A.; Versalovic, J. Biochemical features of beneficial microbes: Foundations for therapeutic microbiology. Spectr. 2017, 5, BAD-0012-2016. https://doi.org/10.1128/microbiolspec.BAD-0012-2016.
- Federle, M.J.; Bassler, B.L. Interspecies communication in bacteria. Clin. Investig. 2003, 112, 1291–1299. https://doi.org/10.1172/JCI20195.
- Zhang, L.; Li, S.; Liu, X.; Wang, Z.; Jiang, M.; Wang, R.; Xie, L.; Liu, Q.; Xie, X.; Shang, D.; et al. Sensing of autoinducer-2 by functionally distinct receptors in prokaryotes. Commun. 2020, 11, 5371. https://doi.org/10.1038/s41467-020-19243-5.
- Thompson, J.A.; Oliveira, R.A.; Djukovic, A.; Ubeda, C.; Xavier, K.B. Manipulation of the quorum sensing signal AI-2 affects the antibiotic-treated gut microbiota. Cell Rep. 2015, 10, 1861–1871. https://doi.org/10.1016/j.celrep.2015.02.049.
- Hsiao, A.; Ahmed, A.M.; Subramanian, S.; Griffin, N.W.; Drewry, L.L.; Petri, W.A., Jr; Haque, R.; Ahmed, T.; Gordon, J.I. Members of the human gut microbiota involved in recovery from Vibrio cholerae Nature 2014, 515, 423–426. https://doi.org/10.1038/nature13738.
- Tondt, J.; Yancy, W.S.; Westman, E.C. Application of nutrient essentiality criteria to dietary carbohydrates. Res. Rev. 2020, 33, 260–270. https://doi.org/10.1017/S0954422420000050.
- Dupre, J.N.; Keenan, M.J.; Hegsted, M.; Brudevold, A.M. Effects of dietary boron in rats fed a vitamin D-deficient diet. Health Perspect. 1994, 102, 55–58. https://doi.org/10.1289/ehp.94102s755.
- Sah, R.N.; Brown, P.H. Techniques for boron determination and their application to the analysis of plant and soil samples. Plant Soil 1997, 193, 15–33. https://doi.org/10.1023/A:1004251606504.
- DeGruttola, A.K.; Low, D.; Mizoguchi, A.; Mizoguchi, E. Current understanding of dysbiosis in disease in human and animal models. Bowel Dis. 2016, 22, 1137–1150. https://doi.org/10.1097/MIB.0000000000000750.
- Durack, J.; Lynch, S.V. The gut microbiome: Relationships with disease and opportunities for therapy. Exp. Med. 2019, 216, 20–40. https://doi.org/10.1084/jem.20180448.
- Chilloux, J.; Neves, A.L.; Boulangé, C.L.; Dumas, M.E. The microbial–mammalian metabolic axis: A critical symbiotic relationship. Opin. Clin. Nutr. Metab. Care 2016, 19, 250–256. https://doi.org/10.1097/MCO.0000000000000284.
- Ahlawat, S.; Asha; Sharma, K.K. Gut–organ axis: A microbial outreach and networking. Appl. Microbiol. 2021, 72, 636–668. https://doi.org/10.1111/lam.13333.
- Favazzo, L.J.; Hendesi, H.; Villani, D.A.; Soniwala, S.; Dar, Q.A.; Schott, E.M.; Gill, S.R.; Zuscik, M.J. The gut microbiome–joint connection: Implications in osteoarthritis. Opin. Rheumatol. 2020, 32, 92–101. https://doi.org/10.1097/BOR.0000000000000681.
- Chisari, E.; Wouthuyzen-Bakker, M.; Friedrich, A.W.; Parvizi, J. The relation between the gut microbiome and osteoarthritis: A systematic review of literature. PLoS ONE 2021, 16, e0261353. https://doi.org/10.1371/journal.pone.0261353.
- Hao, X.; Shang, X.; Liu, J.; Chi, R.; Zhang, J.; Xu, T. The gut microbiota in osteoarthritis: Where do we stand and what can we do? Arthritis Res. Ther. 2021, 23, 42. https://doi.org/10.1186/s13075-021-02427-9.
- Newnham, R.E. Essentiality of boron for healthy bones and joints. Health Perspect. 1994, 102, 83–85. https://doi.org/10.1289/ehp.94102s783.
- Nandwana, V.; Nandwana, N.K.; Das, Y.; Saito, M.; Panda, T.; Das, S.; Almaguel, F.; Hosmane, N.S.; Das, B.C. The role of microbiome in brain development and neurodegenerative diseases. Molecules 2022, 27, 3402. https://doi.org/10.3390/molecules27113402.
- Kim, Y.S.; Unno, T.; Kim, B.Y.; Park, M.S. Sex differences in gut microbiota. World J. Mens Health 2020, 38, 48–60. https://doi.org/10.5534/wjmh.190009.
- Prejac, J.; Skalny, A.A.; Grabeklis, A.R.; Uzun, S.; Mimica, N.; Momčilović, B. Assessing the boron nutritional status by analyzing its cummulative frequency distribution in the hair and whole blood. Trace Elem. Med. Biol. 2018, 45, 50–56. https://doi.org/10.1016/j.jtemb.2017.09.018.
- Bull, M.J.; Plummer, N.T. Part 1: The human gut microbiome in health and disease. Med. (Encinitas) 2014, 13, 17–22. Available online: https://pubmed.ncbi.nlm.nih.gov/26770121/ (accessed on April 20, 2022).
- Vijay, A.; Valdes, A.M. Role of the gut microbiome in chronic diseases: A narrative review. J. Clin. Nutr. 2022, 76, 489–501. https://doi.org/10.1038/s41430-021-00991-6.
- Wang, X.; Xia, N.; Liu, L. Boronic acid-based approach for separation and immobilization of glycoproteins and its application in sensing. J. Mol. Sci. 2013, 14, 20890–20912. https://doi.org/10.3390/ijms141020890.
- Redondo-Nieto, M.; Reguera, M.; Bonilla, I.; Bolaños, L. Boron dependent membrane glycoproteins in symbiosome development and nodule organogenesis: A model for a common role of boron in organogenesis. Plant Signal. Behav. 2008, 3, 298–300. https://doi.org/10.4161/psb.3.5.5276.
- Popov, T.; Angelieva, R. The mechanism of action of boron on the organism of warm blooded animals (entry, distribution and elimination). Sanit. 1969, 34, 78–81. Available online: https://pubmed.ncbi.nlm.nih.gov/5348992/ (accessed on April 20, 2022).
- Baspinar, N.; Basoglu, A.; Semacan, A.; Gulersoy, E. Short term effects of dietary boron on mineral status in dairy cows. J. Environ. Agric. Res. 2017, 3, 65–70. Available online: https://ijoear.com/assets/articles_menuscripts/file/IJOEAR-NOV-2017-2.pdf (accessed on April 20, 2022).
- Newnham, R. Discovering the cure for arthritis. Health 2004, 17, 281–284. https://doi.org/10.1177/026010600401700403.
- Scorei, R.; Cimpoiasu, V.M.; Iordachescu, D. In vitro evaluation of the antioxidant activity of calcium fructoborate. Trace Elem. Res. 2005, 107, 127–134. https://doi.org/10.1385/BTER:107:2:127.
- Scorei, R.; Ciubar, R.; Iancu, C.; Mitran, V.; Cimpean, A.; Iordachescu, D. In vitro effects of calcium fructoborate on fMLP-stimulated human neutrophil granulocytes. Trace Elem. Res. 2007, 118, 27–37. https://doi.org/10.1007/s12011-007-0019-7.
- Scorei, R.I.; Ciofrangeanu, C.; Ion, R.; Cimpean, A.; Galateanu, B.; Mitran, V.; Iordachescu, D. In vitro effects of calcium fructoborate upon production of inflammatory mediators by LPS-stimulated RAW 264.7 macrophages. Trace Elem. Res. 2010, 135, 334–344. https://doi.org/10.1007/s12011-009-8488-5.
- Scorei, R.I.; Rotaru, P. Calcium fructoborate—potential anti-inflammatory agent. Trace Elem. Res. 2011, 143, 1223–1238. https://doi.org/10.1007/s12011-011-8972-6.
- Scorei, R.; Mitrut, P.; Petrisor, I.; Scorei, I. A double-blind, placebo-controlled pilot study to evaluate the effect of calcium fructoborate on systemic inflammation and dyslipidemia markers for middle-aged people with primary osteoarthritis. Trace Elem. Res. 2011, 144, 253–263. https://doi.org/10.1007/s12011-011-9083-0.
- Militaru, C.; Donoiu, I.; Craciun, A.; Scorei, I.D.; Bulearca, A.M.; Scorei, R.I. Oral resveratrol and calcium fructoborate supplementation in subjects with stable angina pectoris: Effects on lipid profiles, inflammation markers, and quality of life. Nutrition 2013, 29, 178–183. https://doi.org/10.1016/j.nut.2012.07.006.
- Biţă, A.; Scorei, I.R.; Mogoantă, L.; Bejenaru, C.; Mogoşanu, G.D.; Bejenaru, L.E. Natural and semisynthetic candidate molecules for COVID-19 prophylaxis and treatment. J. Morphol. Embryol. 2020, 61, 321–334. https://doi.org/10.47162/RJME.61.2.02.
- Scorei, I.R.; Biţă, A.; Mogoşanu, G.D. Letter to the Editor: Boron enhances the antiviral activity of the curcumin against SARS-CoV-2. J. Morphol. Embryol. 2020, 61, 967–970. https://doi.org/10.47162/RJME.61.3.39.
- Văruţ, R.M.; Melinte, P.R.; Pîrvu, A.S.; Gîngu, O.; Sima, G.; Oancea, C.N.; Teişanu, A.C.; Drăgoi, G.; Biţă, A.; Manolea, H.O.; et al. Calcium fructoborate coating of titanium–hydroxyapatite implants by chemisorption deposition improves implant osseointegration in the femur of New Zealand White rabbit experimental model. J. Morphol. Embryol. 2020, 61, 1235–1247. https://doi.org/10.47162/RJME.61.4.25.
- Criste, R.D.; Grossu, D.V.; Scorei, R.; Duca, R.C.; Ciurascu, C.; Mitrut, M. Investigations on the effect of the supplemental VETABOR, boron-enriched concentrate added to broiler diets, on breast meat fatty acids profile. In Proceedings of the XVIIth European Symposium on the Quality of Poultry Meat, Doorwerth, The Netherlands, 23–26 May 2005; pp. 165–169. Available online: https://www.cabi.org/Uploads/animal-science/worlds-poultry-science-association/WPSA-the-netherlands-2005/96.pdf ( April 20, 2022).
- Criste, R.D.; Grossu, D.V.; Scorei, R.; Duca, R.C.; Mitrut, M.; Ciurascu, C. New investigations on the effect of the dietary boron on broilers and layers; boron and food quality. Zootech. 2005, 8, 65–78. Available online: https://www.researchgate.net/publication/261027124_New_investigations_on_the_effect_of_the_dietary_boron_on_broilers_and_layers_boron_and_food_quality (accessed on April 20, 2022).
- Untea, A.; Panaite, T.; Criste, R.D. Effect of the dietary calcium fructoborate given to weaned piglets on calcium balance. Zootech. 2008, 11, 61–68. Available online: https://www.ibna.ro/arhiva/AZ%2011-3/AZ11-3%2006_Arabela.pdf (accessed on April 20, 2022).
- Scorei, I.R. Calcium fructoborate: Plant-based dietary boron as potential medicine for cancer therapy. Biosci. (Schol. Ed.) 2011, 3, 205–215. https://doi.org/10.2741/s145.
- Taranu, I.; Marin, D.E.; Manda, G.; Motiu, M.; Neagoe, I.; Tabuc, C.; Stancu, M.; Olteanu, M. Assessment of the potential of a boron–fructose additive in counteracting the toxic effect of Fusarium Br. J. Nutr. 2011, 106, 398–407. https://doi.org/10.1017/S0007114511000341.
- Liew, W.P.P.; Mohd-Redzwan, S. Mycotoxin: Its impact on gut health and microbiota. Cell. Infect. Microbiol. 2018, 8, 60. https://doi.org/10.3389/fcimb.2018.00060.
- Scorei, R.; Bita, A.; Dinca, L.; Mogosanu, D.; Rangavajla, N. Methods for extracting high content of diester cholorenoborate complex from green coffee beans and uses thereof. United States Patent and Trademark Office (USPTO), Provisional Patent Application No. 63326931/04/04/2022. 2022. Available online: https://www.uspto.gov/patents/basics/types-patent-applications/provisional-application-patent (accessed on April 20, 2022).
- Ferrua, M.J.; Singh, R.P. Human gastric simulator (Riddet model). In The Impact of Food Bioactives on Health: In Vitro and Ex Vivo Models; Verhoeckx, K., Cotter, P., López-Expósito, I., Kleiveland, C., Lea, T., Mackie, A., Requena, T., Swiatecka, D., Wichers, H., Eds.; Springer: Cham, Switzerland, 2015; pp. 61–71. https://doi.org/10.1007/978-3-319-16104-4_7.
- Wang, X.; Ye, A.; Dave, A.; Singh, H. In vitro digestion of soymilk using a human gastric simulator: Impact of structural changes on kinetics of release of proteins and lipids. Food Hydrocoll. 2021, 111, 106235. https://doi.org/10.1016/j.foodhyd.2020.106235.
- Kong, F.; Singh, R.P. A human gastric simulator (HGS) to study food digestion in human stomach. Food Sci. 2010, 75, E627–E635. https://doi.org/10.1111/j.1750-3841.2010.01856.x.
- Minekus, M.; Alminger, M.; Alvito, P.; Balance, S.; Bohn, T.; Bourlieu, C.; Carrière, F.; Boutrou, R.; Corredig, M.; Dupont, D.; et al. A standardised static in vitro digestion method suitable for food—an international consensus. Food Funct. 2014, 5, 1113–1124. https://doi.org/10.1039/c3fo60702j.
- Carrière, F.; Moreau, H.; Raphel, V.; Laugier, R.; Benicourt, C.; Junien, J.L.; Verger, R. Purification and biochemical characterization of dog gastric lipase. J. Biochem. 1991, 202, 75–83. https://doi.org/10.1111/j.1432-1033.1991.tb16346.x.
- Vinarov, Z.; Abrahamsson, B.; Artursson, P.; Batchelor, H.; Berben, P.; Bernkop-Schnürch, A.; Butler, J.; Ceulemans, J.; Davies, N.; Dupont, D.; et al. Current challenges and future perspectives in oral absorption research: An opinion of the UNGAP network. Drug Deliv. Rev. 2021, 171, 289–331. https://doi.org/10.1016/j.addr.2021.02.001.
- Hua, S. Advances in oral drug delivery for regional targeting in the gastrointestinal tract—influence of physiological, pathophysiological and pharmaceutical factors. Pharmacol. 2020, 11, 524. https://doi.org/10.3389/fphar.2020.00524.
- Trinh, P.; Zaneveld, J.R.; Safranek, S.; Rabinowitz, P.M. One health relationships between human, animal, and environmental microbiomes: A mini-review. Public Health 2018, 6, 235. https://doi.org/10.3389/fpubh.2018.00235.
- De Seta, F.; Schmidt, M.; Vu, B.; Essmann, M.; Larsen, B. Antifungal mechanisms supporting boric acid therapy of Candida J. Antimicrob. Chemother. 2009, 63, 325–336. https://doi.org/10.1093/jac/dkn486.
- Bradshaw, C.S.; Brotman, R.M. Making inroads into improving treatment of bacterial vaginosis—striving for long-term cure. BMC Infect. Dis. 2015, 15, 292. https://doi.org/10.1186/s12879-015-1027-4.
- De Seta, F.; Leonardi, L. Compositions containing boric acid and a mixture of Lactobacillus. World Intellectual Property Organization (WIPO), International Patent WO 2015/173693 A1. 2015. Available online: https://patentimages.storage.googleapis.com/ce/b6/32/3bb205af4bcf7d/WO2015173693A1.pdf (accessed on April 20, 2022).
- Routray, I.; Ali, S. Boron induces lymphocyte proliferation and modulates the priming effects of lipopolysaccharide on macrophages. PLoS ONE 2016, 11, e0150607. https://doi.org/10.1371/journal.pone.0150607.
- Rondanelli, M.; Faliva, M.A.; Peroni, G.; Infantino, V.; Gasparri, C.; Iannello, G.; Perna, S.; Riva, A.; Petrangolini, G.; Tartara, A. Pivotal role of boron supplementation on bone health: A narrative review. Trace Elem. Med. Biol. 2020, 62, 126577. https://doi.org/10.1016/j.jtemb.2020.126577.
- Hussain, S.A.; Abood, S.J.; Gorial, F.I. The adjuvant use of calcium fructoborate and borax with etanercept in patients with rheumatoid arthritis: Pilot study. Intercult. Ethnopharmacol. 2016, 6, 58–64. https://doi.org/10.5455/jice.20161204021549.
- Rogoveanu, O.C.; Mogoşanu, G.D.; Bejenaru, C.; Bejenaru, L.E.; Croitoru, O.; Neamţu, J.; Pietrzkowski, Z.; Reyes-Izquierdo, T.; Biţă, A.; Scorei, I.D.; et al. Effects of calcium fructoborate on levels of C-reactive protein, total cholesterol, low-density lipoprotein, triglycerides, IL-1β, IL-6, and MCP-1: A double-blind, placebo-controlled clinical study. Trace Elem. Res. 2015, 163, 124–131. https://doi.org/10.1007/s12011-014-0155-9.
- Becker, R.A.; Bykov, Y.V. Boron preparations in psychiatry and neurology: Their rise, fall and renewed of interest. Acta Biomed. Scient. 2018, 3, 85–100. https://doi.org/10.29413/ABS.2018-3.4.13.
- Doğan, A.; Demirci, S.; Apdik, H.; Bayrak, O.F.; Gulluoglu, S.; Tuysuz, E.C.; Gusev, O.; Rizvanov, A.A.; Nikerel, E.; Şahin, F. A new hope for obesity management: Boron inhibits adipogenesis in progenitor cells through the Wnt/β-catenin pathway. Metabolism 2017, 69, 130–142. https://doi.org/10.1016/j.metabol.2017.01.021.
- Coban, F.K.; Liman, R.; Cigerci, I.H.; Ince, S.; Hazman, O.; Bozkurt, M.F. The antioxidant effect of boron on oxidative stress and DNA damage in diabetic rats. Fresenius Environ. Bull. 2015, 24, 4059–4066. Available online: https://www.researchgate.net/publication/286186112_The_antioxidant_effect_of_boron_on_oxidative_stress_and_DNA_damage_in_diabetic_rats (accessed on April 20, 2022).
- Hernandez, V.; Crépin, T.; Palencia, A.; Cusack, S.; Akama, T.; Baker, S.J.; Bu, W.; Feng, L.; Freund, Y.R.; Liu, L.; et al. Discovery of a novel class of boron-based antibacterials with activity against Gram-negative bacteria. Agents Chemother. 2013, 57, 1394–1403. https://doi.org/10.1128/AAC.02058-12.
- Nielsen, F.H.; Meacham, S.L. Growing evidence for human health benefits of boron. Evid. Based Complementary Altern. Med. 2011, 16, 169–180. https://doi.org/10.1177/2156587211407638.
- MacGillivray, P.C.; Fraser, M.S. Boric acid poisoning in infancy arising from the treatment of napkin rash. Dis. Child. 1953, 28, 484–489. https://doi.org/10.1136/adc.28.142.484.
- Zan, R.; Hubbezoglu, I.; Ozdemir, A.K.; Tunc, T.; Sumer, Z.; Alici, O. Antibacterial effect of different concentration of boric acid against Enterococcus faecalis biofilms in root canal. Marmara Dent. J. 2013, 1, 76–80. Available online: https://dergipark.org.tr/en/pub/marudj/issue/17589/184279 (accessed on April 20, 2022).
- Sayin, Z.; Ucan, U.S.; Sakmanoglu, A. Antibacterial and antibiofilm effects of boron on different bacteria. Trace Elem. Res. 2016, 173, 241–246. https://doi.org/10.1007/s12011-016-0637-z.
- Kremer, D.; Pieters, T.T.; Verhaar, M.C.; Berger, S.P.; Bakker, S.J.L.; van Zuilen, A.D.; Joles, J.A.; Vernooij, R.W.M.; van Balkom, B.W.M. A systematic review and meta-analysis of COVID-19 in kidney transplant recipients: Lessons to be learned. J. Transplant. 2021, 21, 3936–3945. https://doi.org/10.1111/ajt.16742.
- Fan, Y.; Pedersen, O. Gut microbiota in human metabolic health and disease. Rev. Microbiol. 2021, 19, 55–71. https://doi.org/10.1038/s41579-020-0433-9.
- Vandeputte, D.; Joossens, M. Effects of low and high FODMAP diets on human gastrointestinal microbiota composition in adults with intestinal diseases: A systematic review. Microorganisms 2020, 8, 1638. https://doi.org/10.3390/microorganisms8111638.
- Pirouz, F.; Najafpour, G.; Jahanshahi, M.; Sharifzadeh Baei, M. Biodistribution of calcium fructoborate as a targeting agent for boron neutron capture therapy in an experimental model of MDA-MB-231 breast cancer cells. Agric. Biotechnol. 2019, 22, 101389. https://doi.org/10.1016/j.bcab.2019.101389.
- Pirouz, F.; Najafpour, G.; Jahanshahi, M.; Sharifzadeh Baei, M. Plant-based calcium fructoborate as boron-carrying nanoparticles for neutron cancer therapy. J. Eng. Trans. A Basics 2019, 32, 460–466. https://doi.org/10.5829/IJE.2019.32.04A.01.
- Thibeault, S.A.; Fay, C.C.; Sauti, G.; Kang, J.H.; Park, C. Radiation shielding materials containing hydrogen, boron and nitrogen. World Intellectual Properties Organization (WIPO), International Publication No. WO 2013/074134 A1. 2013. Available online: https://patentimages.storage.googleapis.com/20/21/9c/973fbd372c482a/WO2013074134A1.pdf (accessed on April 20, 2022).
- Evcin, A.; Çelen, Y.Y.; Çiçek Bezir, N.; Ersoy, B. Use of ilmenite and boron waste as a radiation shielding panel. AIP Conf. Proc. 2018, 2042, 020001. https://doi.org/10.1063/1.5078873.
- Dong, M.; Zhou, S.; Xue, X.; Feng, X.; Sayyed, M.I.; Khandaker, M.U.; Bradley, D.A. The potential use of boron containing resources for protection against nuclear radiation. Phys. Chem. 2021, 188, 109601. https://doi.org/10.1016/j.radphyschem.2021.109601.
- Guo, H.; Chou, W.C.; Lai, Y.; Liang, K.; Tam, J.W.; Brickey, W.J.; Chen, L.; Montgomery, N.D.; Li, X.; Bohannon, L.M.; et al. Multi-omics analyses of radiation survivors identify radioprotective microbes and metabolites. Science 2020, 370, eaay9097. https://doi.org/10.1126/science.aay9097.
- Ali, F.; Hosmane, N.S.; Zhu, Y. Boron chemistry for medical applications. Molecules 2020, 25, 828. https://doi.org/10.3390/molecules25040828.
- Coghi, P.S.; Zhu, Y.; Xie, H.; Hosmane, N.S.; Zhang, Y. Organoboron compounds: Effective antibacterial and antiparasitic agents. Molecules 2021, 26, 3309. https://doi.org/10.3390/molecules26113309.
- Zhu, Y.; Cai, J.; Hosmane, N.S.; Zhang, Y. Chapter 1—Introduction: Basic concept of boron and its physical and chemical properties. In Fundamentals and Applications of Boron Chemistry, 1st ed.; Zhu, Y., Hosmane, N.S., Eds.; Elsevier: Amsterdam, The Netherlands, 2022; Volume 2, pp. 1–57. https://doi.org/10.1016/B978-0-12-822127-3.00003-X.
- Arora, S.; Chahal, D.S. Effect of soil properties on boron adsorption and release in arid and semi-arid benchmark soils. Soil Sci. Plant Anal. 2010, 41, 2532–2544. https://doi.org/10.1080/00103624.2010.514372.
- Akkurt, İ.; Çanakciı, H.; Mavi, B.; Günoğlu, K. Natural radioactivity of boron added clay samples. AIP Conf. Proc. 2011, 1400, 512–517. https://doi.org/10.1063/1.3663173.
- Moraetis, D.; Al Lamki, M.; Muhammad, D.; Yaroubi, S.; Al Batashi, H.; Pracejus, B. Boron content and sources in Tertiary aquifers in the Sultanate of Oman. . Available online: https://ui.adsabs.harvard.edu/abs/2017EGUGA..19.3180M/abstract (accessed on April 20, 2022).
- Bilgici Cengiz, G.; Öztanriöver, E. Analysis of natural radioactivity levels in soil samples and dose assessment for Digor district, Kars, Turkey. Caucasian J. Sci. 2018, 5, 30–39. Available online: https://dergipark.org.tr/en/pub/cjo/issue/38939/424149 (accessed on April 20, 2022).