Transition fibers, which are present in unicellular organisms, or distal and subdistal appendages, which are present in mammals, are attached to microtubules within the transition zone[19]. Transition fibers function as docking sites for intraflagellar transport (IFT) proteins[20]. IFT transports cargo in a bidirectional manner along the length of cilia and is mediated by kinesin-2 (anterograde) and cytoplasmic dynein-2 motors (retrograde) attached to multisubunit protein complexes known as IFT particles[21][22]. Y-linkers exist at the distal end of the transition zone and secure the doublet microtubules to the ciliary membrane in most organisms[19].
2.2 Multicilia
In vertebrates, MCCs are present in a wide variety of different tissue types. In mammals, ependymal MCCs line brain ventricles and the airway epithelium. Multicilia are produced by specialized cells for highly specialized functions. MCCs are typically defined as having more than two cilia on their surface, although this occurrence is not well documented or understood. Recently, MCCs have been observed in unicellular eukaryotes and protists, as well as many metazoans, and even in certain plant sperms [23][24][25]. MCCs result in the production of motile axonemes, with the only notable exception being mammalian olfactory cilia. These olfactory MCCs lack dynein arms and are considered immotile despite having a 9 + 2 structure. This occurrence is indicative of MCCs being a solution to the need for local fluid flow, possibly due to their ability for hydrodynamic coupling [6][26][27].
Multicilia carry out their functions by beating, and the basic machinery and organization of cilia beating seems well conserved between eukaryotes, as well as between single motile cilium and multicilia. Some parameters, such as beat frequency, are under cellular control and are varied among cell types. In addition, only motile cilia and sperm flagella contain the dynein machinery needed to power axonemal beating during ATP hydrolysis
[5][28]. The ciliary beat cycle has two phases: the effective stroke, and the recovery stroke. The effective stroke is the initially bending from its upright position, while the recovery stroke sees it return to its original, unbent position. Ciliary motility is controlled by outer and inner axonemal dynein arms, which slide adjacent doublets in respect to one another. The sliding is mediated by protein bridges between doublets, and by the basal anchoring of the axoneme. As a result, cilia bend
[6][29]. The phenomenon metachrony occurs when cilia are organized in such a way that each cilium, in a two-dimensional array, will beat at the same frequency, but in a phase shifted manner. As a result, a traveling wave of ciliary action moves across the array, which propels fluids in a current. Even if each cilium in an array starts off in synch, hydrodynamic forces between each cilium will nudge them back towards metachrony, possibly because in a metachronal array, the work each cilium must do is reduced, and more fluid is displaced. Because of this, multiciliation is thought to be a more evolutionarily efficient way to generate fluid flow
[6][30][31][32].
2.3 Primary cilia
Nearly all human cells house a single nonmotile cilium on their surface, and these primary cilia serve sensory and signaling purposes. The role of primary cilia function and formation on animal health and pathophysiology has only recently been brought to researcher’s attentions and is even now not fully understood. A wealth of new information about the primary cilia has been discovered within the last few decades, shedding some light on the function of the previously thought
vestigial organelle
[33].
Primary cilia are part of various signaling pathways in vertebrates, and usually lack a microtubule central pair (9 + 0 axoneme), as well as the structures associated with the central doublet pair, such as the inner and outer dynein arms required for ciliary movement
[34][35]. Primary cilia can be found in, but are not limited to, endothelia, epithelia, and neurons. Although found in nearly every mammalian cell, notable exceptions include the intercalated cells of the kidney collecting ducts, red blood cells, hepatocytes, and MCCs
[34].
Primary cilia function mainly as sensory hubs and are host to many different groups of mechanosensory proteins, chemosensory receptors, and ion channels. These translate extracellular stimuli into an intracellular biochemical signal, which cause different cellular responses. There are two current models that attempt to explain how primary cilia function: the compartment model, and the scaffold model. The compartment model states that the ciliary structure is essential for proper signaling, while the scaffold model states that IFT molecules must play a part in either signaling itself, or the acquisition of outside transduction intermediates
[36][37]. As stated in the ciliogenesis section, primary cilia form through IFT, which transports proteins along the microtubules of the axoneme. The axoneme acts like a scaffold for certain protein complexes, including kinesins and dyneins, which facilitate back-and-forth trafficking of cargo proteins along the length of the cilium. Along with their creation, IFT is also required for maintenance of the primary cilium, and possibly even their core functions
[10].
The ciliary membrane is an extension of the cellular membrane where a host of proteins and receptors are housed due to the ciliary transition zone, which provides docking sites for molecular transport into and out of the cilioplasm (
Figure 1)
[38][39][40]. While there is no confirmed mechanism by which molecules enter and exit the cilia, several mechanisms have been proposed. One such mechanism is the active transport of vesicles from the Golgi apparatus to docking sites in the transition zone
[41]. The vesicles are thought to interact with exocyst complexes, where they experience soluble N-ethylmaleimide-sensitive factor (NSF) attachment protein receptor (SNARE) mediated diffusion across the cilioplasm/cytoplasm barrier
[37]. The BBSome, which resides in the basal body and is an octameric protein complex, is involved in the movement of transmembrane proteins to the ciliary membrane. BBSomes are known to recognize ciliary targeting sequences and will readily interact with molecules that are upstream of Rab8 activation. BBSomes are not thought to be required for any aspect of ciliogenesis; nevertheless, if BBSomes fail to deliver certain vital proteins to the cilia, the cilia may lose functionality
[42][43][44][45]. Another proposed mechanism of molecule trafficking is the action of transmembrane proteins, of which some are associated with specific protein sequences that target cilia localization, such as the N-terminal RVxP sequence on polycystin-2 (PC-2)
[42][46].
Primary cilia serve many mechanosensory functions within the body. For example, proper kidney function depends on regulated fluid flow through the nephrons and collecting ducts, which controls the glomerular filtration rate
[47]. This flow is sensed by the epithelial primary cilia present in the kidney. Fluid redirection by the primary cilia causes an increase in intracellular calcium. This calcium influx is also mediated by the PC1/2 ion channel complex, and both the mechanosensitive polycystin-1 (PC-1) membrane protein and the PC-2 channel localize to the primary cilia
[48][49][50]. In renal cells, defects in this ion channel complex, along with complete disruption of cilia formation, is known to result in PKD
[51].
3. Nitric oxide
Nitric oxide is a signaling molecule involved in a wide array of cellular pathways; mainly, NO contributes to the normal functions of a variety of organ systems
[52]. NO is highly reactive, and readily diffuses across cellular membranes. As a result, NO is found in many paracrine signaling pathways. NO is mainly synthesized from l-arginine, oxygen, and NADPH in a redox reaction, catalyzed by nitric oxide synthase (NOS)
[53]. NOS has three isoforms, but only endothelial NOS (eNOS) and neuronal NOS (nNOS) are constantly and consistently expressed in cells. Both eNOS and nNOS are calcium dependent, while the other NOS isoform, cytokine inducible NOS (iNOS), is expressed by pro-inflammatory cytokines on an as needed basis. iNOS and nNOS are both soluble enzymes that exist within the cytosol. eNOS, however, is found to localize to the plasma or Golgi body membranes. Because of its unique and wide cellular and subcellular distribution, NOS has many diverse functions throughout the entirety of the body
[54][55].
4. Cilia and nitric oxide interplay
Although primary cilia and NO have various independent roles within the body, especially in the vasculature, their functions often intersect and cooperate with each other. Most research on the interaction between endothelial primary cilia and nitric oxide focuses on vascular homeostasis, but their interactions extend into other areas
[1]. However, this entry will focus on signaling cascades that lead to NO biosynthesis or increased NO bioavailability. The following discussion of the interactions between primary cilia and nitric oxide will focus on vasodilation, wound healing, dopamine signaling, and cellular proliferation.
4.1 Vasodilation
Primary cilia and NO independently effect vasculature in different ways, but recent studies suggest a direct relationship may exist between the two. Vascular endothelial cells are present in the blood vessel wall and are in continuous contact with blood flow-generated fluid shear stress. Endothelial cells are known mechanotransducers of fluid shear stress, which causes the biosynthesis of NO. This helps regulate vascular tone; NO will diffuse into the surrounding smooth muscle, producing vasorelaxation
[56].
Evidence supports primary cilia as the main sensor in this mechanosensitive pathway. As stated previously, PC-1, a mechanosensory protein that malfunctions in polycystic kidney disease, localizes to vascular endothelial primary cilia. In an
in vitro study performed by Nauli
et al., which investigated PC-1’s fluid shear mechanosensory properties, it was found that in contrast to wildtype endothelial cells, the PC-1 knockout cells failed to produce an increase in cytosolic calcium and the corresponding NO flux in response to fluid shear stress. The authors, to demonstrate that calcium and NO signals are induced in response to ciliary PC-1 activation, used
Tg737orpk/orpk endothelial cells that lack ciliary ultrastructure but have functional PC-1. The results showed that neither calcium nor NO signals were present at flow rates up to 50 dyne/cm
2[3] These results suggest that PC-1 is responsible for proper cilia mechanosensory function, and that ciliary PC-1 specifically elicits NO production.
Follow-up studies by AbouAlaiwi
et al. Showed that PC-2, which, as stated previously, is a calcium permeable cation channel that forms a complex with PC-1, is also important for mechanotransduction. Studies using a PC-2 knockdown line of cells showed a reduction in calcium and NO flux under shear stress when compared to control cells. This was further validated in
ex vivo studies, where endothelial cells isolated from
pkd2−/− mice arteries failed to respond to fluid shear stress
[4]. These results indicate both PC-1 and PC-2 are needed for cilia mechanosensation, and further suggest that activation of the PC-1/PC-2 complex will start the signaling cascade needed for calcium-dependent NO biosynthesis. In addition, the results show that the increase in intracellular calcium is caused by an increase in intra-ciliary calcium. However, other researchers have proposed that calcium moves bidirectionally between the cilia and the cytosol
[57][58][59]. Regardless, the increase in intracellular calcium triggers the calcium/calmodulin complex, which activates constitutive NOS, such as eNOS, by binding to its target site on the enzyme
[60].
The calcium/calmodulin complex can also indirectly activate eNOS through activation of the AKT/PKB (protein kinase B/phosphatidylinositol 3-kinase) pathway, which stimulates AMPK (adenosine 5′-monophosphate-activated protein kinase) (
Figure 2)
[61]. eNOS activation is mainly calcium dependent, but some studies have shown that a calcium independent pathway exists, via heat shock protein 90 (HSP90). HSP90 is also known to localize to cilia axonemes and may act as a signal transductor that interacts with eNOS in the vasculature
[62][63]. HSP90 activation can lead to an increase in eNOS activity while calcium levels are high and can also lead to more eNOS activity at low calcium levels due to its ability to directly bind to eNOS and increase the binding affinity for calmodulin
[64][65].
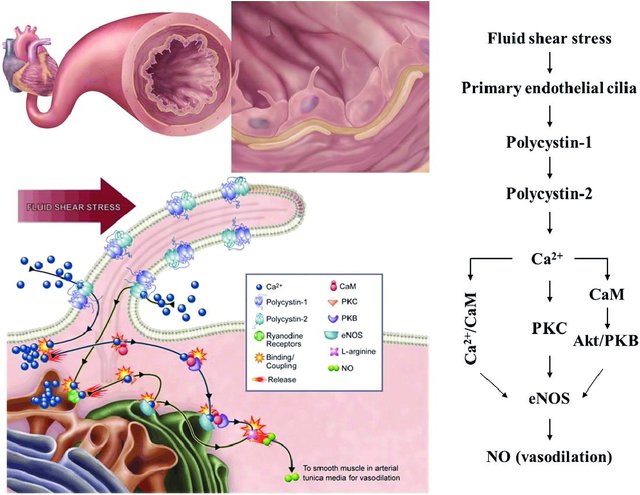
Figure 2. Primary cilia activation via fluid shear stress and NO signaling in the vascular endothelia. The left panel shows primary cilia bending while under fluid shear stress, with the resultant production and release of nitric oxide (NO). The production and release of NO is dependent on the activation of endothelial primary cilia within the vasculature. The bending of cilia via fluid-shear stress activates the mechanosensory polycystin complex, which initiates the synthesis and the release of NO. This biochemical cascade, shown in the right panel, involves an extracellular calcium influx (Ca2+), followed by the activation of multiple calcium-dependent proteins, including calmodulin (CaM), protein kinase C (PKC) and Akt/PKB. Figure is adopted from Ref. [1].
4.2 Wound healing
An under researched aspect of NO and primary cilia is their interaction in the vascular smooth muscle cell (VSMC) layer. Depending on blood vessel type, all three isoforms of NOS may exist within the VSMC layers
[66]. During normal function, the cilia on the VSMC layer extend towards the extracellular matrix. Under abnormal conditions, such as a scratch wound, VSMC primary cilia will migrate to the wound edge. A recent study showed that VSMC cilia express polycystins, as well as α3-and β1-integrins. When the researchers blocked integrin function, the percent of cilia migrating to the wound edge dropped from about 88% to around 30%
[67]. This drop suggests that VSMC primary cilia may be involved in integrin-mediated wound healing.
In further support of the purported role primary cilia play in wound healing, results from experiments using VSMC that lacked cilia showed a slower scratch wound healing time than the ciliated control cells displayed
[67]. While the cilia in the wounded area are directly exposed to constant fluid shear stress from blood flow, activation of the mechanosensory ciliary polycystin complex could occur, resulting in an increase in intracellular calcium
[1]. This could potentially trigger vasoconstriction in VSMC, resulting in the isolation of the wounded area to allow the platelets to begin clot formation. As soon as the calcium amount reached a certain level, the calcium/calmodulin complex would form and activate eNOS and nNOS, leading to vasodilation and the next step in the wound healing process.
Further studies by Schneider
et al. reveled that, once cellular growth was halted, platelet derived growth factor receptor alpha (PDGFRα), a tyrosine kinase with a prominent function in cellular proliferation, was found to localize to fibroblast primary cilia. Furthermore, ligand activation of PDGFRα will lead to the activation of the AKT and MEK/ERK (mitogen-activated protein/extracellular signal-regulated kinase) proliferative pathways. Because AKT and ERK1/2 regulate eNOS activity in endothelial cells, PDGFRα activation could indirectly lead to eNOS activation
[68]. Moreover, data from recent studies on endothelial progenitor cells indicate that PDGF-AA (platelet-derived growth factor AA (PDGF-AA) might contribute a vital role in wound healing, possibly by its effects on angiogenesis through the PI3K/Akt/eNOS signaling pathway
[69]. Bone morphological protein (BMP) receptor II (BMPRII), which is highly expressed on endothelial cells in lung vasculature, as well as moderately expressed in smooth muscle, is also involved in cell wound migration. In this pathway, migration is trigged by the ligands BMP2 and BMP4, which result in eNOS being phosphorylated
[70]. While not conclusive, this evidence, when taken all together, suggests that primary cilia may have a significant part to play in the wound healing process.
When tissues begin to repair themselves after a wound, clots must be dissolved to maintain proper blood flow. This is known as clot retraction and platelet inhibition. NO is known to inhibit platelet aggregation, secretion, adhesion, and fibrinogen binding; all through activation of guanylyl cyclase and cGMP, alongside the inhibition of thromboxane A2. By this mechanism, platelet aggregation and accumulation are reduced, enabling the clot to dissolve, and the wound to heal fully
[71][72][73]. Given the evidence, it is possible that an interaction between primary cilia and NO could be important in the wound healing and repair processes.
4.3 Dopamine signaling
Hypertension present in PKD patients in the later stages of the disease is made worse by increased kidney volume. However, hypertension can also be seen in children, as well as the early stages of PKD, long before renal function starts to deteriorate. Some evidence suggests that an increase in sympathetic activation occurs in these patients, independently of their kidney function. Dopamine, an endogenous neuronal hormone that acts within the sympathetic nervous system, is confirmed to be involved in the regulation of blood pressure. Abnormal dopamine signaling can lead to hypertensive states in humans. Dopamine receptor 1 (D1) and 5 (D5) have been found to localize to primary cilia
[39][74][75][76]. While there are no current therapies that target D1 or D5, some studies using D1-like receptor subtypes showed vasodilatory effects in peripheral arteries
[77].
The D5 receptor is thought to have both a chemosensory and mechanosensory role within primary cilia. Subjecting endothelial ciliary knockout cells
pkd1−/− (lacking PC-1), and
Tg737orpk/orpk cells that have no cilia, to dopamine under static conditions revealed a significantly subdued calcium influx when compared to the control cells. The researchers contributed this to the presence of underdeveloped cilia in the knockout cells, which would have less D5 receptors on them due to their smaller size. Under flow conditions with added dopamine, the mechanosensory function of the cilia knockout cells was restored, in comparison to the untreated knockout cells. Because calcium influx in these cell lines is associated with eNOS activation, the results of this study suggest a potential restoration of lost vasodilatory responses caused by a failed ciliary induction of NO biosynthesis
[74]. There is additional evidence that suggests dopamine receptor 2 (D2) may also localize, or possibly get transported to, the primary cilia
[78]. In one study, cerebral vasospasms were
reversed with dopamine treatment; but when haloperidol, a D2 selective antagonist drug, was administered, the vasorelaxation failed to occur. It was also reported that, after administration of dopamine, a large increase in eNOS and iNOS expression was seen, and administration of haloperidol also blocked this effect
[79].
D2 is also possibly transported to the primary cilia under specific conditions to mediate NOS activity within cells. Evidence supporting the role of ciliary dopamine receptors in the mediation of NO can be found in autosomal dominant polycystic kidney disease (ADPKD) patient clinical trials. ADPKD patients experience extrarenal maladies that mainly affect their cardiovascular system, such as hypertension. The hypertensive state could be brought on, in part, by the inability of primary endothelial cilia to respond to alterations in blood pressure. This would cause a failure to synthesize NO. In a study conducted by Lorthioir
et al., flow-mediated dilation of normotensive ADPKD patients was compared to that of adults without ADPKD. It was shown that ADPKD patients had significantly less vasodilation during sustained flow increases, as well as a total loss of NO release when compared to those without ADPKD. When ADPKD patients were administered brachial infusions of 0.25–0.5 μg/kg/min of dopamine, there was an increase in flow-mediated dilation, and a statistically significant increase in dilatory response at the highest dose
[80]. According to these results, dopamine receptors may facilitate a connection between primary cilia, NO, and blood pressure regulation in ADPKD patients.
4.4 Cell proliferation
Primary cilia also help regulate cell proliferation. As stated in the ciliogenesis section, the cilia extend from the basal body, which is composed of mother and daughter centrioles, and cilia are reabsorbed after cell cycle re-entry
[12]. In cancerous cell clusters, cilia are missing from the more prolific dividers, which suggests that despite not playing a major role in cell division, primary cilia are important for starting and stopping cell mitosis
[81][82][83][84].
NO possibly plays a role in cell proliferation as well, in conjunction with primary cilia. NO has been proven to halt the cell cycle by preventing the transition from G1 to S phase, in a dose dependent manner. The spike in NO is caused by an increase in free l-arginine, which is mediated by various cytokines. PC-1 is a known mediator of the JAK/STAT (Janus kinases/signal transducer and activator of transcription proteins) pathway by activating STAT3; when the cytosolic tail of PC-1 is cleaved upon once luminal flow halts, it can coactivate STAT-1, −3, and −6, along with JAK2. The PC-1 tail causes the cells to sensitize to cytokines and growth factor signaling, which then causes an exaggerated cellular response, which could potentially lead to an increase in l-arginine
[85][86]. Through this mechanism, overly prolific cell division would be arrested.
The superfamily of TGF-β (transforming growth factor beta) signaling provides a fascinating system of cellular crosstalk, in which the effects of the same ligand can be unique depending on the cell type and the physiological conditions. This family is composed of more than 30 different ligand types of the TGF-β-activin-Nodal BMP subfamilies that can activate receptor serine/threonine kinases of types I and II (TGFβRI/II and BMP-RI/II, respectively). Ciliopathies widely overlap with phenotypes associated with aberrant TGF-β/BMP signaling. Prominent examples include structural heart defects associated with congenital heart disease
[87], suggesting that cardiac primary cilia may contribute to cellular events regulated by TGF-β/BMP signaling events during heart development. Moreover, different components of the TGF-β signalosome, including TGF-βRI, TGF-βRII, SMAD2/3, SMAD4, and SMAD7 are present at the cilia-centrosome axis. In a recent study, Feng
et al. concluded that high salt-induced endothelial dysfunction and the development of salt-dependent increases in blood pressure were related to endothelial TGF-β signaling. Specifically, TGF-β-dependent ALK5 signaling increases endothelial NOX4 (NADPH oxidase-4), an enzyme that produces hydrogen peroxide, which limits NO bioavailability and ultimately promotes increased blood pressure
[88]. BMPRII contributes to cell proliferation through its interactions with primary cilia, eNOS, and NO. Using pulmonary artery endothelial cells, studies have shown that stimulation of BMPRII results in eNOS activation. BMPRII ligands BMP2 and BMP4 stimulate eNOS phosphorylation at a regulatory site via activation by protein kinase A. This eNOS stimulation results in increased NO bioavailability; loss of BMPRII function, therefore, is proposed to contribute to endothelial dysfunction
[70].
5. Conclusion
Primary cilia and nitric oxide are both essential for normal tissue functions. While they can operate independently of one another, often their roles and cellular pathways are complementary. While this entry only touched on a small portion of their possible connections, scattered research suggests a more complex linkage between the two in many more organ systems and cellular pathways. However, current research on the direct links between primary cilia and NO are scarce. The aim of this entry was to discuss the more well-known links between primary cilia and NO, and to initiate discussion leading to further examination of the topics covered. Revealing the connections between cilia and NO would provide insight into various ciliopathies and could reveal new targets for therapies.