Minerals and trace elements are important micronutrients for normal physiological function of the body. They are abundant in natural food sources and are regularly included in dietary supplements whereas highly processed industrial food often contains reduced or altered amounts of them. In modern society, the daily intake, storage pools, and homeostasis of these micronutrients are dependent on certain dietary habits and can be thrown out of balance by malignancies. Their dietary imbalance, which is becoming more common in the diets of industrialized countries, is linked to an increased risk of cancer.
1. Introduction
After World War Two, the mean food intake increased drastically, reflected in an ongoing obesity epidemic
[1][2]. On the other hand, the quality of the food did not. Specifically, there has been a decrease in several minerals in foods over the last five decades, most notably zinc, copper, and iron
[3][4][5]. Such an occurrence is mirrored in the worldwide spread of “hidden hunger”, defined as a prolonged lack of vitamins or minerals (collectively referred to as micronutrients) intake
[6][7]. For example, it has been estimated that the daily intake of selenium is half that of the reference nutrient intake
[8].
Micronutrients are necessary for immunological functions as well as the general cellular metabolism
[9]. For instance, it has been shown that deficiency in micronutrients, including minerals, worsens the pathogenesis of COVID-19
[10]. Moreover, given the link between an abnormal immune system and oncogenesis
[11], identifying factors that affect the former can aid in predicting the latter. Quantification of biomolecules that can interfere with immune function, for example, could theoretically serve as an indicator for cancer risk. Micronutrients in general and minerals, in particular, are suitable for this purpose.
Given that cellular biochemistry requires micronutrients, the ongoing and unnoticed subclinical deficiency of micronutrients might cause significant global health issues. In particular, the hidden hunger might increase the risk of cancer development. Consequently, the measurement of micronutrients in general and minerals, in particular, might help the early identification of people at higher cancer risk.
The recommended daily intake and associated serum levels of the most common minerals are summarized in Table 1 and serve as the basis for the categorization of the minerals referred to.
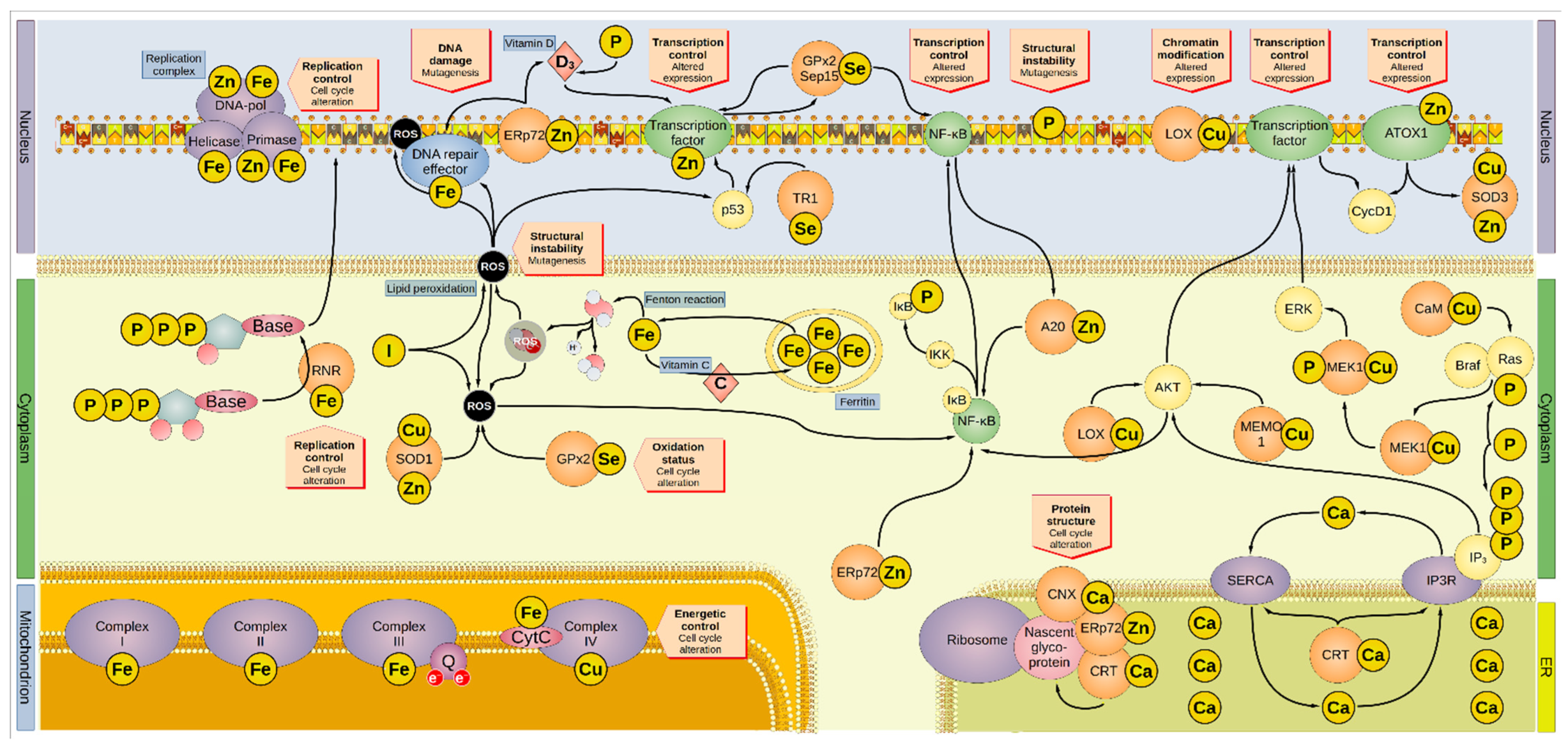
Figure 1. Overview of the involvement of minerals in oncogenesis. Minerals are involved in several and overlapping cellular pathways. Iron is sequestered by ferritin inside the cytoplasm. Iron leaking from the ferritin cages can react with water to form hydroxyl radical, one of the many ROS. Vitamin C promotes the reload of iron inside the ferritin cages. ROS can interact with lipid bilayers, generating more ROS molecules by lipid peroxidation, a process inhibited by iodide. In addition, ROS can directly damage the DNA and proteins, including the DNA-repair enzymes that are activated by ROS-induced damage. Furthermore, ROS activates p53 and NF-κB, altering the cell cycle. Iodide, SOD1, and GPx2 inactivate ROS. For instance, SOD1 removes superoxide radicals by dismutation reaction generating H2O2, O2, and GPx2 by producing two H2O from auto-reduction. The DNA-repair factors are modulated by the zinc-protein ERp72, which regulates the intake of vitamin D involved in the regulation of transcriptional factors. Cellular intake of vitamin D is also regulated by phosphorus. ERp72 reduces disulfide bonds in nascent proteins in the ER, in association with the calcium-proteins CNX and CRT. Moreover, ERp72 regulates NF-κB, the latter being also modulated by GPx2 and Sep15. GPx2 and Sep15 promote their own expression. A20 and AKT also activate NF-κB, which then reduces the activity of A20 in a negative feedback loop. AKT is modulated by LOX, MEMO1, and IP3, and affects the cell cycle by promoting the expression of CycD1. LOX can alter genetic expression by modifying the histones. Some transcription factors contain zinc, and a transcription regulator is p53, which TR1 modulates. ATOX1 is a zinc-containing protein that can modulate gene expression, particularly that of cyclin D1 and SOD3 (which regulate the oxidative environment outside the cell). Genetic transcription and cell cycle are regulated by ERK, which MEK1 activates after being phosphorylated by the complex Ras/Braf. One of the modulators of Ras is CaM, but also phosphorus can directly boost its activation. Similarly, phosphorus is also part of active IP3 that, aside from its direct modulation of AKT, regulates the release of calcium (effectively a second messenger on its own right) from the ER via the Ca2+ channel IP3R. The P-type ATPase SERCA mediates the transport of cytosolic calcium back into the ER. CRT regulates both IP3R and SERCA. Moreover, minerals are involved in DNA replication since they are embedded in several subunits of the DNA replication complex (namely: iron in the helicase, primase, and DNA-polymerase α, the latter two also containing zinc). Iron is present in the first three mitochondrial complexes and CytC, whereas copper is present in complex IV. Thus, minerals are essential to the energetic balance of the cell and its oxidative state. AKT, Ak strain transforming; A20, zinc finger protein A20; ATOX1, antioxidant-1; Braf, rapidly accelerated fibrosarcoma isoform B; CaM, calmodulin; CNX, calnexin; CRT, calreticulin; CycD1, cyclin D1; CytC, cytochrome c; DNA-pol, DNA polymerase; ER, endoplasmic reticulum; Erp72, endoplasmic reticulum resident protein 72; ERK, extracellular signal-regulated kinase; GPx2, glutathione peroxidase 2; IκB, NF-κB inhibitor; IKK, IκB kinase; IP3, inositol 1,4,5-trisphosphate; IP3R, inositol trisphosphate receptor; LOX, lysyl oxidase; MAPK, mitogen-activated protein kinase; MEK1, MAPK/ERK kinase1; MEMO1, mediator of cell motility 1; NF-κB, nuclear factor kappa-light-chain-enhancer of activated B cells; Q, coenzyme Q; Ras, rat sarcoma virus; RNR, ribonucleotide reductase; ROS, reactive oxygen species; Sep15, selenoprotein of 15-kDa; SERCA, sarco-/endoplasmic reticulum calcium ATPase; SOD, superoxide dismutase; TR1, thioredoxin reductase 1.
Table 1. Recommended daily intakes and related serum levels of the minerals reported her *.
Mineral |
RDA/PRI (µg/Day) |
EAR/AR (µg/Day) |
UL (µg/Day) |
Serum Levels |
Source |
References |
Iron |
8000 |
6000 |
45,000 |
30 µg/L † |
Meat, fish, cereals, beans, nuts. |
[12][13][14] |
Zinc |
8000–11,000 |
9400 |
25,000 |
≥800 µg/L ‡ |
Meat, legumes, eggs, fish, grains. |
[12][15][16] |
Selenium |
30–70 |
70 |
300 |
47–145 µg/L |
Meat, fish. |
[12][17] |
Phosphorus |
700,000 |
580,000 |
n.d. |
0.8–1.5 × 103 µmol/L |
Meat, fish. |
[12][18] |
Calcium |
1,000,000 |
750,000 |
2,500,000 |
2500 µmol/L |
Milk, fish, legumes. |
[12][14][19] |
Copper |
900 |
1600 |
5000 |
1200 µg/L |
Milk, fish, eggs, vegetables. |
[12][20] |
Iodine |
150 |
95 |
600 |
40–80 µg/L |
Marine products, eggs, milk, iodized salt. |
[12][21] |
* Depicted data refers to male adults. AR: average requirement; EAR: estimated average requirement; n.d.: not determined by EFSA; PRI: population reference intake; RDA: recommended daily allowance; UL: tolerable upper intake level. † as ferritin. ‡ not reliable indicator for zinc status.
2. Minerals and Risk of Cancer
Nutrition’s role in oncogenesis is increasingly being researched and supported by new scientific data. As a result, scientists are becoming more and more interested in determining the role of micronutrients in DNA stability, epigenetic regulation, immunological response, and in assessing their role as biomarkers.
Tumorigenesis is extremely complex and varies greatly between tumor entities due to the involvement of multiple molecular processes. The present analysis revealed some intriguing tendencies, even though there is no unified trend relating mineral intake and cancer risk. Specifically, higher levels of copper, iron, and iodine were linked to higher cancer risk, but zinc, selenium, calcium, and phosphorus had a more mixed relationship. A higher mineral intake was linked to an increased risk of oral cancer (iron, selenium, phosphorus, and zinc). Higher levels of zinc and copper, but lower levels of selenium, were more associated with liver cancer. Prostate cancer, on the other hand, was associated with higher phosphorus intake. The association between minerals and risk of cancer in observational and intervention studies is summarized in Table 2, Table 3 and Table 4, respectively.
Table 2. Summary of the association between minerals and risk of cancer in observational studies.
Mineral |
Organ |
Sample |
Association * |
Measure † |
Reference |
Zinc |
Breast |
Tissue |
Direct |
Qt |
[22] |
|
Brain |
Tissue |
Inverse |
Qt |
[23] |
|
|
Intake |
Inverse |
Qt |
[24] |
|
Mouth |
Intake |
None |
Qt |
[25] |
|
|
Serum |
Inverse |
Qt |
[26] |
|
Liver |
Serum |
None |
HR |
[27] |
|
|
Serum |
Direct |
Qt |
[28] |
|
Colon |
Tissue |
Inverse |
OR |
[29] |
Copper |
Liver |
Serum |
Direct |
HR |
[27] |
|
|
Serum |
Direct |
Qt |
[28] |
|
Mouth |
Serum |
Direct |
Qt |
[26] |
|
Colon |
Tissue |
Direct |
OR |
[29] |
|
Brain |
Tissue |
Inverse |
Qt |
[23] |
|
|
Serum |
Direct |
Qt |
[30] |
|
|
Intake |
Inverse |
Qt |
[24] |
|
Pancreas |
Serum |
Direct |
Qt |
[31] |
Selenium |
Esophagus |
Tissue |
Direct |
Qt |
[32] |
|
Prostate |
Tissue |
None |
Qt |
[33] |
|
|
Serum |
None ║ |
Qt |
[34] |
|
Any |
Serum |
None |
Qt |
[35] |
|
|
Serum |
Inverse |
Qt |
[36] |
|
Liver |
Serum |
Inverse |
Qt |
[37] |
|
|
Serum |
Inverse |
Qt |
[38] |
|
Colon |
Serum |
None ¶ |
IR |
[39] |
|
Pancreas |
Serum |
Inverse |
OR |
[31] |
|
Breast |
Serum |
Inverse |
HR |
[40] |
|
|
Serum |
Inverse |
HR |
[41] |
|
Lung |
Serum |
None |
Qt |
[42] |
|
Lung |
Serum |
Direct |
HR |
[43] |
|
Kidney |
Serum |
Inverse |
OR |
[44] |
|
Mouth |
Intake |
Direct |
Qt |
[25] |
Phosphorus |
Brain |
Intake |
Direct |
Qt |
[24] |
|
Prostate |
Intake |
None |
RR |
[45] |
|
|
Intake |
Direct |
Cr |
[45] |
|
|
Intake |
Direct |
OR |
[46] |
|
|
Intake |
None |
OR |
[47] |
|
Colon |
Intake |
Inverse # |
RR |
[48] |
|
Bladder |
Intake |
None |
OR |
[49] |
|
Mouth |
Intake |
Direct |
Qt |
[25] |
Calcium |
Prostate |
Tissue |
Direct |
Qt |
[33] |
|
Brain |
Intake |
Direct |
Qt |
[24] |
|
Ovary |
Serum |
Direct |
Qt |
[50] |
|
Breast |
Serum |
Inverse |
HR |
[51] |
|
Mouth |
Serum |
Direct |
Qt |
[26] |
Iron |
Any |
Serum |
Direct/Inverse |
HR |
[52] |
|
|
Intake |
Direct |
HR |
[53] |
|
Stomach |
Tissue |
Direct |
Qt |
[54] |
|
Brain |
Intake |
Direct |
Qt |
[24] |
|
Mouth |
Intake |
Direct |
Qt |
[25] |
|
|
Serum |
Direct |
Qt |
[26] |
|
Breast |
Serum |
None |
HR |
[55] |
|
Thyroid |
Urine |
Direct |
OR |
[56] |
Iodine |
Breast |
Urine |
Direct |
Qt |
[57] |
|
Rectum |
Tissue |
Direct |
Cr |
[58] |
* Direct: high micronutrient concentration is linked to an increased risk of cancer; inverse: low micronutrient concentration is linked to an increased risk of cancer; none: no significance observed. † Cr: correlation; Qt: comparison of levels between groups; HR: hazard ratio; IR: incidence rate ratio; OR: odds ratio; RR: relative risk. ║ Observed inverse trend by ethnic stratification. ¶ Observed inverse trend by sex stratification. # Observed in adenomas but not in carcinomas.
Table 3. Summary of the mineral levels in the studies retrieved here.
Mineral |
Cancer Entity |
Sample |
Case Group (Cancer) Mean Mineral Content Number of Patients |
Control Group (Healthy) Mean Mineral Content Number of Patients |
Reference |
Zinc |
Breast |
Tissue † |
3.5–19.5 ppm (n = 26) |
0.8–11.4 ppm (n = 26) |
[22] |
|
Glioblastoma |
Tissue |
0.0403 µg/cm2 (n = 11) |
0.0285 µg/cm2 (n = 11) |
[24] |
|
|
Tissue † |
0.20 g/kg (n = 6) |
0.27 g/kg (n = 6) |
[23] |
|
Colon |
Serum |
96.4 µg/dL (n = 966) |
97.1 µg/dL (n = 966) |
[29] |
Copper |
Glioblastoma |
Tissue |
0.0090 µg/cm2 (n = 11) |
0.0079 µg/cm2 (n = 11) |
[24] |
|
|
Tissue † |
0.48 g/kg (n = 6) |
1.26 g/kg (n = 6) |
[23] |
|
|
Serum |
27.5 µmol/L (n = 52) ‡ |
19.7 µmol/L (n = 52) ‡ |
[30] |
|
Colon |
Serum |
138.6 µg/dL (n = 966) |
135.8 µg/dL (n = 966) |
[29] |
|
Pancreas |
Serum |
1432 µg/L (n = 100) |
1098 µg/L (n = 100) |
[31] |
Selenium |
Any |
Serum |
58.8 µg/L * |
84.8 µg/L (n = 966) |
[36][39] |
|
Esophageal |
Tissue † |
0.73 µg/g (n = 30) ‡ |
0.59 µg/g (n = 30) ‡ |
[32] |
|
Prostate |
Tissue |
191 µg/kg (n = 49) |
168 µg/kg (n = 49) |
[33] |
|
|
Serum |
0.13 µg/g (n = 467) |
0.14 µg/g (n = 936) |
[34] |
|
Breast |
Serum |
90.5 ng/mL (n = 100) |
91.3 ng/mL (n = 1186) |
[40] |
|
Liver |
Serum |
67.47 µg/L (n = 187) |
108.38 µg/L (n = 120) |
[37] |
|
Colon |
Serum |
84.0 µg/L (n = 966) |
85.6 µg/L (n = 966) |
[39] |
|
Pancreas |
Serum |
60.0 µg/L (n = 100) |
76.0 µg/L (n = 100) |
[31] |
|
Lung |
Serum |
166.00 ng/g (n = 48) |
144.74 ng/g (n = 39) |
[42] |
|
Renal |
Serum |
161.7 µg/L (n = 401) |
288.8 µg/L (n = 774) |
[44] |
Phosphorus |
Glioblastoma |
Tissue |
1.71 µg/cm2 (n = 11) |
3.01 µg/cm2 (n = 11) |
[24] |
Iron |
Glioblastoma |
Tissue |
0.037 µg/cm2 (n = 11) |
0.118 µg/cm2 (n = 11) |
[24] |
|
Oral |
Serum |
194.6 µg/dL * |
128.6 µg/dL * |
[26] |
Calcium |
Prostate |
Tissue |
657 mg/kg (n = 50) |
1431 mg/kg (n = 49) |
[33] |
|
Oral |
Serum |
14.7 mEq/L * |
9.4 mEq/L * |
[26] |
|
Ovary |
Serum |
9.34 mg/dL (n = 170) |
9.31 mg/dL (n = 344) |
[50] |
* No number of people per group reported.
† Comparison between tumoral mass and healthy surrounding tissues.
‡ Estimated from article’s figures using
WebPlotDigitizer v. 4.5
[59].
Table 4. Summary of the daily intake of minerals in the studies retrieved here.
Mineral |
Cancer Entity |
Case Group (Cancer) Mean Mineral Intake Number of Patients |
Control Group (Healthy) Mean Mineral Intake Number of Patients |
Reference |
Zinc |
Oral |
12,851 µg/day (n = 27) |
11,788 µg/day (n = 86) |
[25] |
|
Bladder |
14.5 mg/day (n = 198) |
14.7 mg/day (n = 377) |
[49] |
Copper |
Bladder |
2.5 mg/day (n = 198) |
2.8 mg/day (n = 377) |
[49] |
Selenium |
Oral |
142.9 µg/day (n = 27) |
166.7 µg/day (n = 86) |
[25] |
Phosphorus |
Oral |
1761 mg/day (n = 27) |
1431 mg/day (n = 86) |
[25] |
|
Bladder |
1898.3 mg/day (n = 198) |
1940.4 mg/day (n = 377) |
[49] |
Iron |
Oral |
22.4 mg/day (n = 27) |
18.9 mg/day (n = 86) |
[25] |
|
Bladder |
21.3 mg/day (n = 198) |
23.1 mg/day (n = 377) |
[49] |
Calcium |
Bladder |
1127.2 mg/day (n = 198) |
1194.5 mg/day (n = 377) |
[49] |
Remarkably, the observation that cancer risk increases with both low and high serum iron levels
[52] implies that maintaining physiological levels of micronutrients is critical to avoiding an imbalance of cellular biochemistry that can promote oncogenesis. A balanced diet can ensure the intake of the Goldilocks’ quantities of micronutrients, but the depletion of minerals and vitamins in foodstuff makes this increasingly difficult. Regularly checking the mineral levels can help identify whether the physiological range has been met or if the “hidden hunger” has developed increasing the cancer risk.
Given the interdependence of many micronutrients, predicting the role of a single mineral on the oncogenic pathway is cumbersome. For example, serum phosphorus is also linked to calcium and vitamin D and the cellular bioavailability of iron depends on that of vitamin C. Such complexities suggest that minerals may not be suitable cancer biomarkers individually, but a panel of said micronutrients, including vitamins, may provide a more complete picture. Because multiple variables must be considered, it is plausible to expect that micronutrient panels would be best analyzed using machine learning approaches, providing personalized indices to assess potential increased cancer risk.
An imbalanced diet that is often involved in cancer development, is a clear and present danger, especially in industrialized countries. It is not only the high food intake that represents a problem. The vast majority of consumers are unaware of the actual composition of the food or beverages they consume, a situation exacerbated by the fact that food safety authorities do not require manufacturers to declare the actual amounts of micronutrients present in the aliments
[60]. The trend in micronutrient depletion reported in the last decades, coupled with the increasing prevalence of the “hidden hunger”, suggests that health issues associated with nutritional deficiency will become more prominent in the near future. According to the World Health Organization, two billion individuals worldwide, including those in wealthy countries, suffer from micronutrient deficiency that is largely clinically undetected
[61]. The addition of micronutrients to food (fortification) has been purposely introduced to fight the spread of nutritional deficiencies
[62]. However, the effectiveness of this precaution is still debated and does not consider its role on cancer prevention
[63][64][65][66].
While most knowledge on the medical effect of minerals is associated with high intakes, the impact of mineral deprivation on oncogenesis is still not fully understood. The most recognized medical conditions associated with minerals deficiencies, such as MD, are congenital and include stillbirth, high infant mortality, and impaired development. The role of mineral deficiency in adulthood and its involvement in oncogenesis is still unclear.
The data gathered here highlight a direct association between high intake of micronutrients (namely copper, iron, and iodine) and cancer risk. The message may point to a potential contradiction: while the average amount of micronutrients in food is decreasing, a higher cancer risk is associated with a higher amount of minerals. The solution to this paradox could be two-sided. On the one side, while most mineral concentrations in food are reduced, some minerals are augmented; this is particularly true for phosphorus and iron, particularly from heme. Mineral depletion, on the other side, may cause cells to adopt a transformed phenotype that abnormally increases the intracellular amount of micronutrients, for example, through the aberrant expression of surface importers.
Assuring a well-balanced diet is a difficult task, especially in a food market dominated by advertisements for “junk food”. Rather than relying on labels to report the amounts of selected ingredients (a process hampered by manufacturers’ use of incomprehensible chemical names for key health-associated micronutrients), it is more feasible to embrace population-wide nutritional education. If children are educated on a balanced diet beginning in primary school in a few generations, consumers will be the advisors of their nutritional intake without relying too much on labels. Nutrition deficiency will be reduced in this context, as will the biochemical risks associated with micronutrient overload.
3. Conclusions
In conclusion, herein confirms the potential of micronutrients as biomarkers, although the strong interdependence of micronutrient levels, could necessitate looking at a wide range of micronutrients to increase the significance of the findings. There was a tendency of a direct link between copper, iron, iodine, phosphorus, and zinc levels and the development of different cancer types, with the exception of colon cancer. Selenium serum levels were instead inversely related to cancer risk. However, more data are needed to assess the effectiveness of these biomarkers and if they are suitable only for specific types of cancer. Clustered micronutrient analyses could help the establishment of suitable micronutrient panels with diagnostic value to predict individual cancer risk and to facilitate predictions about the prognosis of particular types of cancer. Furthermore, the individual necessity of supplementation with food supplements, especially micronutrients, should always be critically questioned as long as there is no evident deficiency.
This entry is adapted from the peer-reviewed paper 10.3390/cancers14051256