Thermochromic smart windows technology can intelligently regulate indoor solar radiation by changing indoor light transmittance in response to thermal stimulation, thus reducing energy consumption of the building. In recent years, with the development of new energy-saving materials and the combination with practical technology, energy-saving smart windows technology has received more and more attention from scientific research.
1. Introduction
The energy crisis is a major problem threatening human society. In recent years, heating, ventilation and refrigeration account for 43% of the global primary energy consumption
[1], and the problem of building energy consumption
[2] has attracted widespread attention
[3]. In the global total energy consumption, building energy consumption accounts for more than one-third, and nearly half of it is lost by building windows.
At present, to solve the problem of building energy consumption, usually adopt the method of dynamic adjustment
[3], changing building roof and metope adornment to achieve the goal of energy saving. For example, the use of highly reflective cooling coatings
[4], wet roofing
[5] and porous materials
[6] not only have certain effects on maintaining indoor temperature stability but also have non-negligible defects. When the weather is cold, the coating will not work, and it is difficult to reduce the building heating energy consumption. The coating is not suitable for year-round use
[7]. In addition, the wet roof is not suitable used in the winter
[5]. In the complex natural environment, a system that can spontaneously respond to temperature changes to meet the needs of practical applications, in order to better solve the problem of building energy consumption
[8].
Compared with the development of an intelligent regulation system on the top of the wall, window renovation is the most effective and simple way to realize the intelligent heat transfer controlling and effectively reducing the total energy consumption of the buildings
[9]. Therefore, it is necessary to improve the glass window
[10][11]. Generally ideal smart windows usually meet the following conditions: (1) In the use of the process does not increase energy consumption; (2) wide range of regulation and control; (3) strong self-regulation ability of the system.
To solve the above problems, thermochromic smart windows
[12] have been researched. Under heating or cooling conditions, the optical transmittance, reflectivity and color of thermochromic materials will change significantly. They are the best materials for making smart windows. Compared with electrochromic smart windows and gas smart windows
[13], thermochromic smart windows have the advantages of no external control device, no need to consume additional energy and simple structure
[14].
Thermochromic material-produced smart windows commonly exhibit an inorganic phase change material and an organic polymer material. Inorganic phase change material mainly focuses on vanadium dioxide (VO
2), which undergoes a metal–insulator transition at the critical temperature (
Tc) of 68 °C, accompanied with huge transmittance contrast in the IR range and negligible change in the visible range. Vanadium dioxide (VO
2) used as the thermochromic material has attracted more attention in recent years
[15]. When the external temperature (
T) is lower than the phase transition temperature (
Tc), vanadium dioxide will undergo a reversible phase transition. VO
2 is a monoclinal phase with low reflectance in the near-infrared band
[16]. When the external temperature (
T) is greater than the phase transition temperature (
Tc), the phase of VO
2 could change from a monocline state to a rutile state (
Figure 1a). In this state, VO
2 has a large number of free electrons, the transmittance of the near-infrared band drops and the reflectivity increases
[16]. The reversible transformation of vanadium dioxide from monoclinic crystal phase to rutile crystal phase increases its absorbance in the near-infrared spectrum. The optical throughput curve is shown in
Figure 1b.
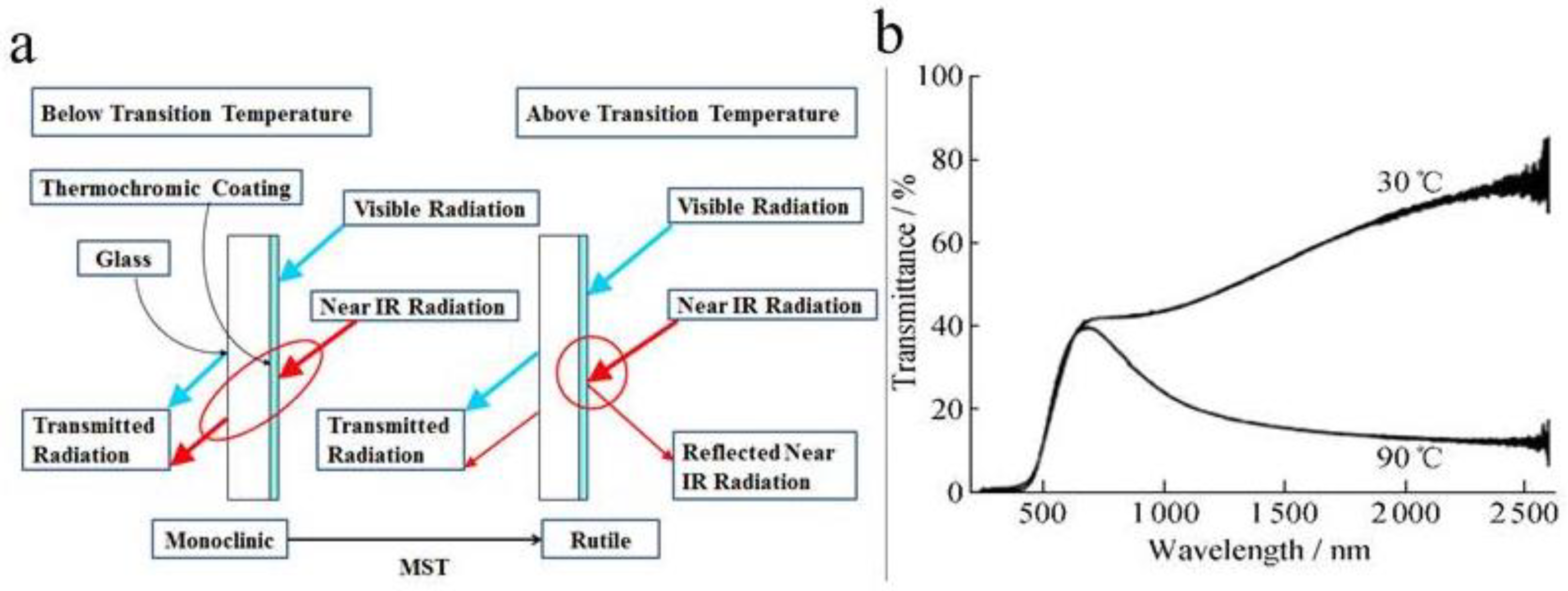
Figure 1. (
a) Schematic diagram of VO
2 thermochromic smart window dimming
[17]. (
b) Optical transmittance curves at VO
2 in high temperature (90 °C) and low temperature (30 °C) states
[18].
The phase transition temperature can be effectively reduced by doping W
6+ Nb
5+ Cr
3+ plasma or nanostructure VO
2 [19]. In addition, the visible light transmittance of VO
2 thin films is low (usually between 40~50%), resulting in the solar modulation ability being unsatisfactory
[18].
Due to the above disadvantages of smart windows prepared by inorganic phase change material VO
2 [20], polymer thermochromic smart windows have received extensive attention in recent years
[19]. Compared with traditional inorganic thermochromic materials, organic polymer thermochromic materials possess low preparation costs and excellent sunlight regulation ability
[10]. Moreover, most organic polymer materials are environmentally friendly and suitable to use in thermochromic smart windows
[21].
Hydrogels-based smart windows, including thermochromic ionic liquid and liquid crystal smart windows, are summarized and discussed (Figure 2). Firstly, the categories and strategies for the performance improvement of these three hydrogels-based smart windows are outlined. Secondly, the integration of the hydrogel with the multifunction devices was discussed. The last section summarizes the prospects of smart windows for future development and applications.
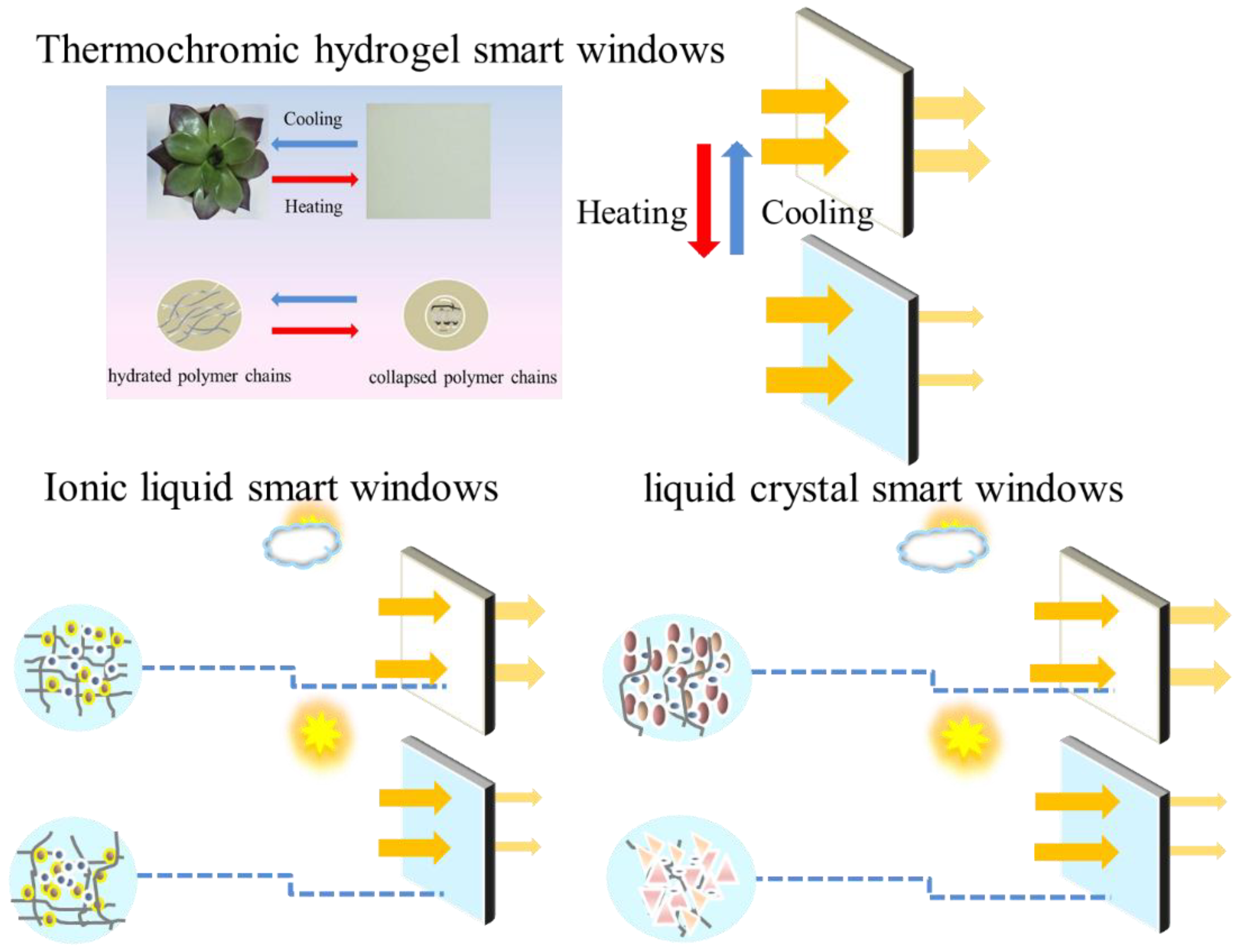
Figure 2. Illustration of thermochromic, Ionic liquid and liquid crystal smart windows.
2. Thermochromic Hydrogel Smart Windows
The thermochromic smart windows use the characteristic that the light transmittance of thermochromic materials changes with temperature to dynamically adjust sunlight without consuming additional energy
[22]. Another advantage of thermochromic smart windows is that it does not need an additional control system
[23]. Thermally responsive organic materials, such as hydroxypropyl cellulose
[24] and polyacrylamide
[21], play an important role in thermochromic smart windows
[25].
In addition, thermochromic functions can be combined with other functions, such as electrothermy-based active control and thermosensitive/electrochromic
[26], for better light modulation and energy utilization.
2.1. PNIPAm-Based Thermochromic Materials Smart Windows
2.1.1. PNIPAm Temperature-Responsive Hydrogel
A novel organic thermochromic material PNIPAm was used to replace the traditional inorganic phase change material VO
2 [27]. With appropriate thickness, PNIPAm temperature-responsive hydrogel was completely transparent at room temperature, and the thickness of the thin layer reached 87.9%. Translucent at 40 °C
[28], the thickness of the thin layer can reach 59.9%. Compared with the currently reported best VO
2 thermochromic film (Δ
Tsol(20–90 °C)22.3%,
Tlum(20 °C) 45.6%
[13], and
Tlum(90 °C) 40.0%)
[29], they can provide both high modulation in the visible and infrared range, which lead overall to enhanced Δ
Tsol(20–40 °C) of 20.4% or 25.5% for Δ
Tsol(20–60 °C) [30].
According to the above discussion, the application of PNIPAm hydrogel film in smart windows is mainly related to the thickness of hydrogel
[31]. The thickness effect of “thin” hydrogels with thickness less than 100 μm was further studied. When the thickness increases from 26 μm to 78 μm and the temperature decreases from 40 °C to 20 °C
[32], the water absorption strength at 1930 nm and 1430 nm increases significantly (
Figure 3a). It can be seen from
Table 1 that the
Tlum(20 °C) of the three samples remained unchanged
[7], while the
Tlum(40 °C) decreased monotonically from 80% (26 μm) to 20% (78 μm)
[33]. While the 78 μm thick hydrogel showed impressive Δ
Tsol values of nearly 50%, its low
Tlum(40 °C) of less than 20% is less satisfactory for an ideal smart window
[34].
Tlum at high temperatures and Δ
Tsol are also suitable for hydrogels of 26 μm thickness
[31]. At temperatures above lower critical solution temperature (LCST), extremely low thickness accompanied by almost constant brightness transmittance and poor solar regulation ability occur
[35]. Averaged
Tlum(40 °C) decreases with thickness, Δ
Tlum, Δ
TIR and Δ
Tsol increases with the thickness (
Figure 3b)
[36].
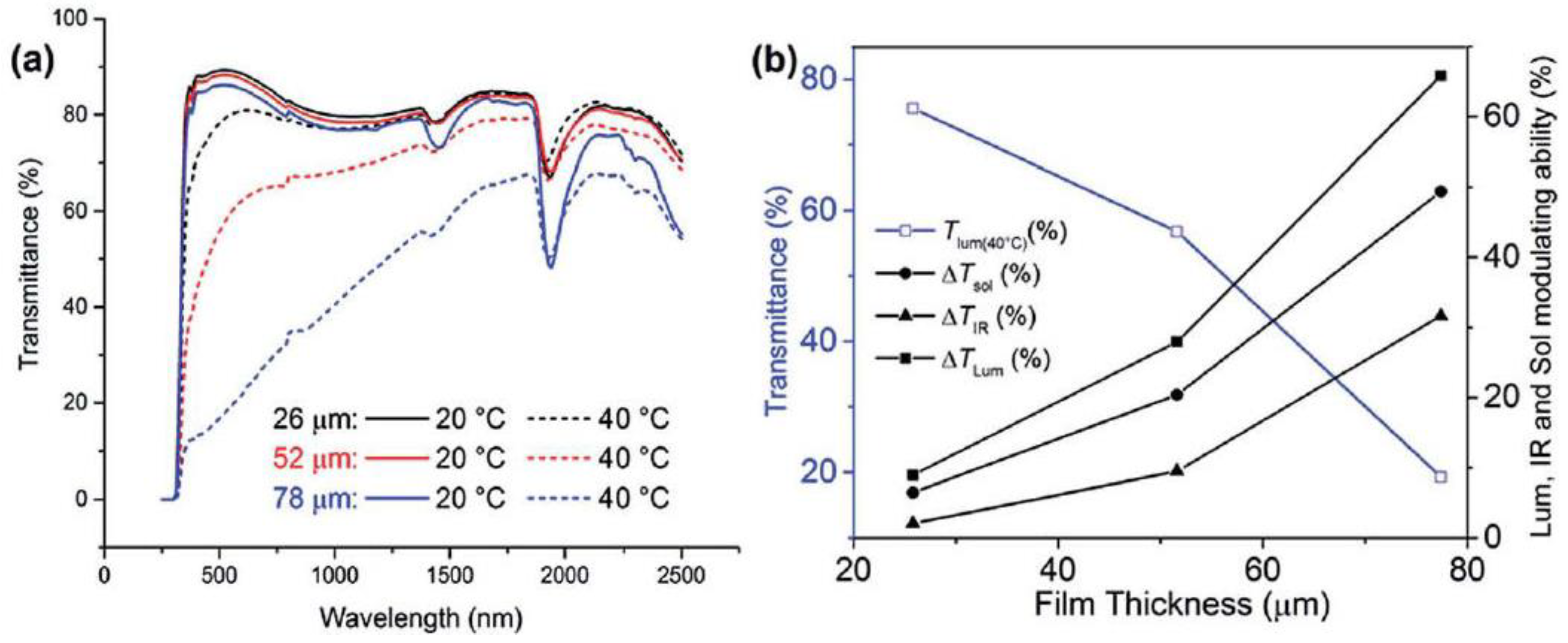
Figure 3. (
a) Optical transmission spectra of three samples with different thicknesses at 20~40 °C. (
b) The variation trend of Δ
Tsol, Δ
Tlum, Δ
TIR and Δ
Tlum at 40 °C with different sample thicknesses
[21][36].
Table 1. Thermochromic properties of 52 μm thick PNIPAm hydrogel films
[21].
|
20 °C |
30 °C |
35 °C |
40 °C |
50 °C |
60 °C |
Tlum (%) |
87.9 |
63.3 |
62.6 |
59.9 |
56.5 |
53.6 |
TIR (%) |
80.0 |
72.6 |
72.7 |
70.5 |
68.4 |
66.1 |
Tsol (%) |
83.0 |
65.5 |
65.0 |
62.3 |
59.8 |
57.5 |
This high-performance temperature-responsive hydrogel can facilitate the development of thermochromic smart windows based on organic materials
[35].
2.1.2. PNIPAm Thermally Responsive Liquid Hydrogel
Conventional smart windows can only adjust solar energy transmission
[33]. Researchers extracted the hydrogel-derived liquid in glass
[37] and developed the high thermal energy storage thermoresponsive smart window (HTEST smart windows)
[15]. Excellent thermal response optical properties, coupled with high liquid specific heat capacity, enable HTEST smart windows to have excellent energy-saving performance. Simulation results show that, compared with ordinary glass in Singapore
[38], the HTEST windows can reduce the energy consumption of heating, ventilation and air conditioning by 44.6%. In the outdoor demonstration, HTEST smart windows showed good energy-saving performance during the summer day
[39].
Optical photos of samples of different thicknesses at 20 °C and 60 °C. The optical image is consistent with spectrum: at low temperatures, all samples are transparent light transmittance free from thickness
[13]. Moreover, when the temperature is higher than LCST
[36], transmittance of 0.1 mm sample
[40] does not change significantly. In contrast, the 1 mm sample becomes translucent
[41], the 1 cm sample becomes opaque, and flowers under the 1 cm sample become invisible (
Figure 4a)
[42]. Therefore, thermal response optical characteristics of thermoresponsive liquid (TRL) can be adjusted by changing temperature as well as thickness of TRL
[43] significant difference in optical transmittance (
Figure 4b). By adjusting the hydrophilic and hydrophobic balance of thermochromic hydrogels through copolymerization, the phase transition process can be flattened, while the transmittance near the phase transition temperature changes slowly with the change of ambient temperature (
Figure 4c). Transmittance spectra of an ion gel at different temperatures of 15~60 °C. The spectral range of 300~2200 nm is affected, with temperature increase of ion gel. when the ion gels were heated above LCST, up to 80% luminous modulation (Δ
Tlum) was to be achieved by changing the observable color to white (
Figure 4d), as reported by Lee et al.
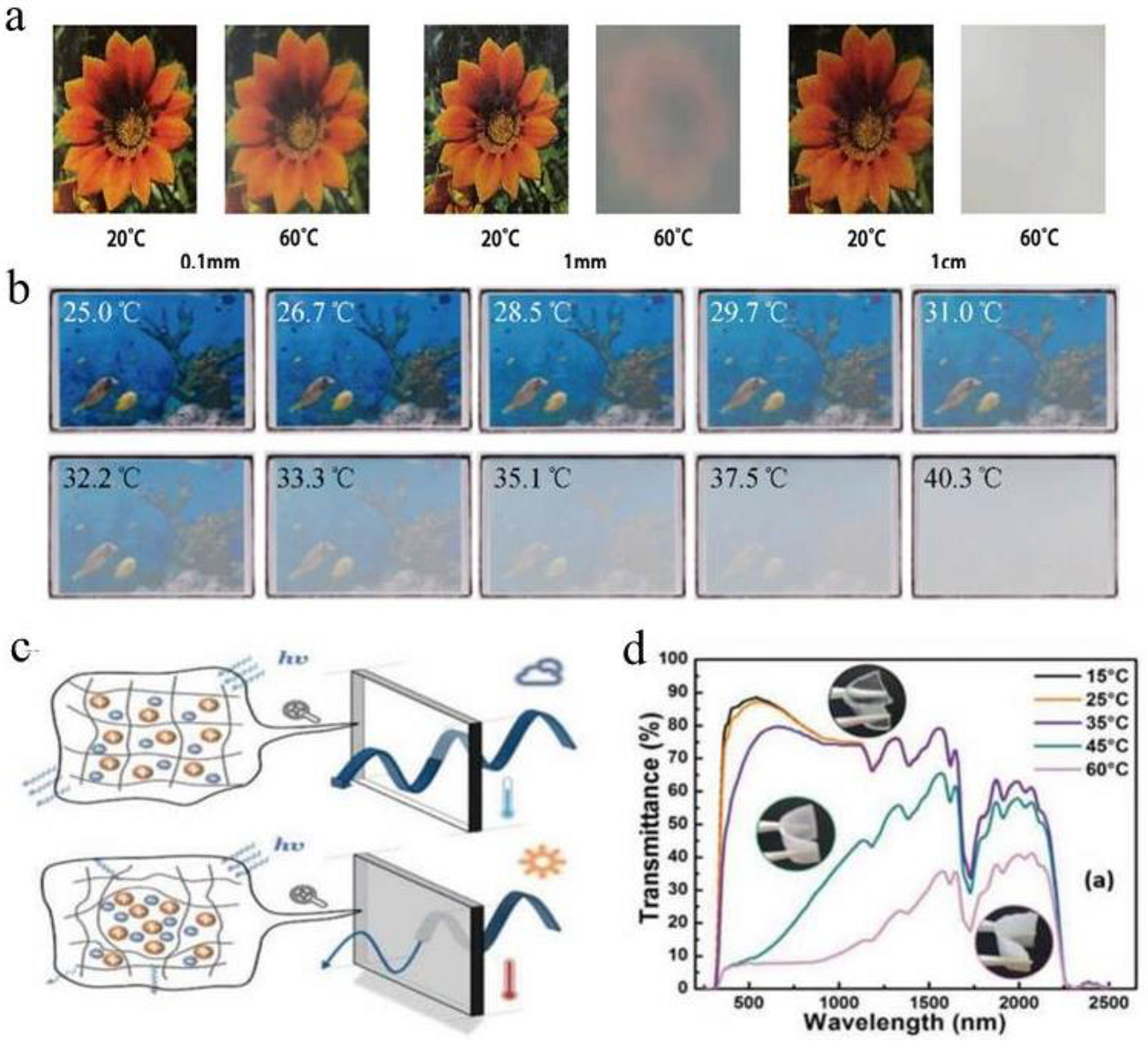
Figure 4. (
a) Optical photographs of 0.1 mm, 1 mm and 1 cm samples at 20 °C and 60 °C. (
b) Schematic diagram of thermochromic optical transmittance as a function of temperature. (
c) Schematic diagram of the change of thermochromic light transmittance. The reduced transparency of the ionic liquid above the LCST is due to light scattering at the interface between the ionic liquid and the polymer chain. (
d) Transmission spectra of the UV-Vis-NIR regions at different temperatures of 15 to 60 °C
[5][42].
Compared with traditional energy-saving smart windows
[44], which require expensive equipment, the thermally responsive liquid structure is easy to manufacture, has good uniformity and expansibility and has the function of sound insulation, which opens a new path for energy-saving buildings and greenhouses
[45].
2.1.3. PNIPAm/Antimony-Doped Tin Oxide (ATO) Nanocomposite Hydrogel
Photothermal and temperature-sensitive nanocomposite composed of hybrid poly PNIPAm hydrogel and antimony-doped tin oxide (ATO) were reported by the Li group
[44]. In this photothermal system, near-infrared (NIR) absorption of ATO can be used as an optical switch to induce hydrogel induced by nano heater
[46]. This new type of passive smart windows has excellent near-infrared shielding performance. The photothermal activation switch mechanism enhances the thermal response speed and solar modulation capability.
[47] ATO doped with 0, 5, 10 and 15 at% Sb separately in PNIPAm was studied, it was found that PNIPAm/ATO nanocomposites could be photothermal activated
[48]. The PNIPAm /ATO doped with 10 at% Sb showed the best thermal response speed and solar modulation ability. Different film thicknesses and ATO content will affect the response rate and solar modulation ability. By increasing the content of Sb dopant in SnO
2 to 10 at%, the concentration of free electrons increases, leading to the improvement of near-infrared absorption efficiency. This means ATO can convert absorbed solar radiation into heat, also known as the photothermal effect. For structural stability, the performance stability of such a photothermal system
[49] can be proved by 15 consecutive periods of irradiation under solar intensity (
Figure 5c).
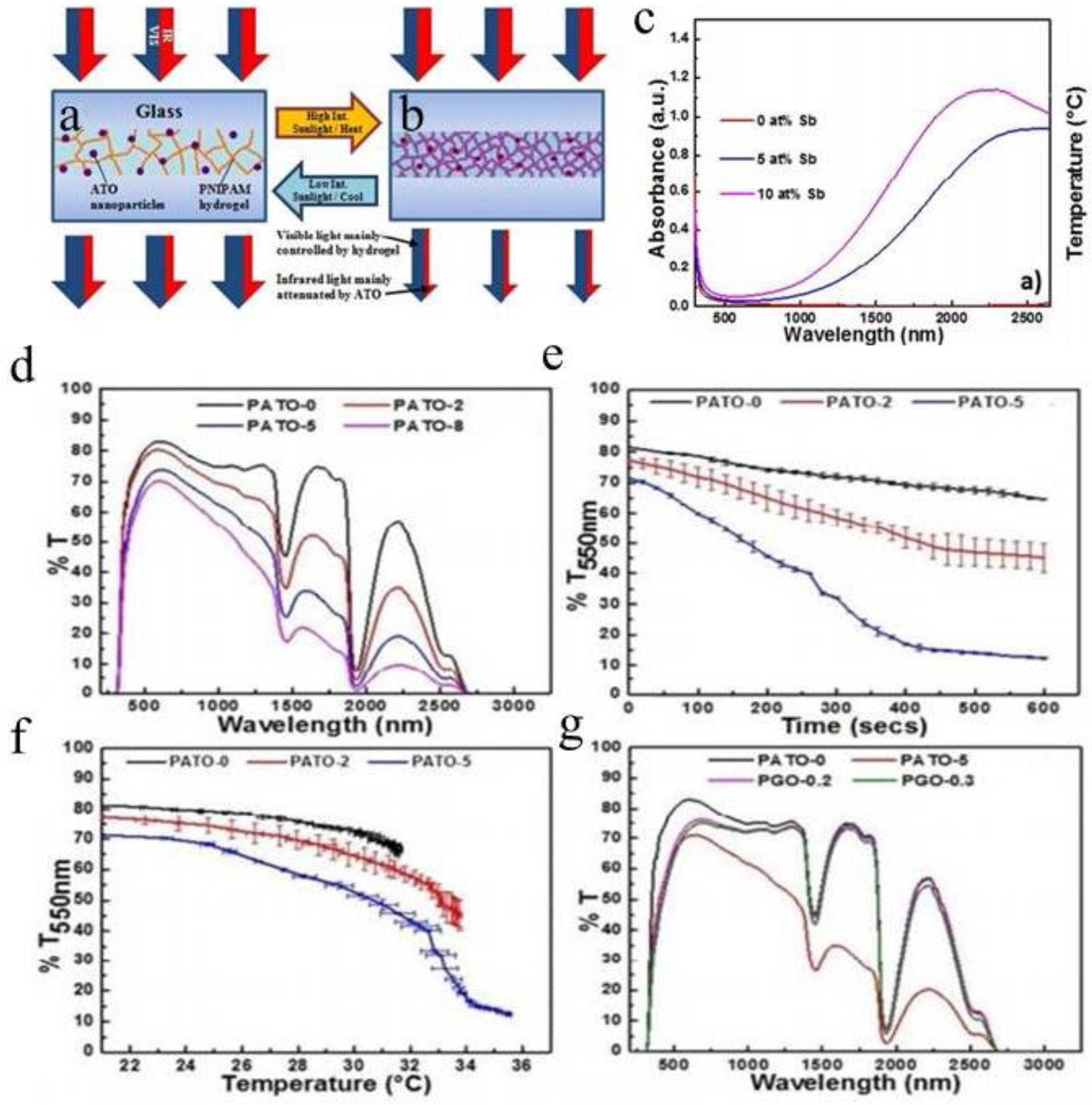
Figure 5. Optical sensitive smart windows (
a) transparent state and (
b) translucent state structure diagram. (
c) UV-Vis-NIR absorption spectra of 0.025 wt% SnO
2 dispersed in perchloroethylene solvents with Sb doping of 0, 5 and 10 at%
[49]. (
d) UV-Vis-NIR spectra of nanocomposite hydrogel films with different filling contents at 25 °C. Single-wavelength transmittance rate (
T550%) nanocomposite hydrogel as a function of € time and (
f) temperature from solar irradiation at 100 mW cm
−2 for 10 min. The figure above shows the optical properties of the 180 nm hydrogel film incorporated with various content of 10 at% Sb doped ATO. (
g) UV-Vis-NIR spectra of PATO Compared with different levels of PGO at 25 °C
[50].
When PNIPAm/ATO composite hydrogels (PATO) are exposed to sunlight irradiation (
Figure 5a,b)
[17], dimming control can be facilitated and accelerated by plasmonic heating of ATO under glaring sunlight even if the temperature outdoor is far below the transition point of PNIPAm hydrogel
[45].
As the content of ATO in PATO increases, the absorption of the plasma increases, resulting in a decrease in transmission in the NIR region (
Figure 5d). With the increase of ATO content, a significant increase in the response rate is observed in addition to the lower visible light transmittance in the transparent state (
Figure 5e). The optical effects of different ATO concentrations on the PATO are further observed in the transmittance temperature profile (
Figure 5f)
[45]. Use ATO as an alternative to graphene oxide (GO), as a nano heater. Their performance is compared in (
Figure 5g). Unlike the PATO showing the NIR shielding effect, the PGO shows only a decreased visible transmittance as the GO content increases, while the spectrum in the NIR region remains the same as the PNIPAm
[17].
In the background of the smart windows, NIR absorption is an important feature of indoor temperature regulation. Thus, not only does it sacrifice the photothermal rate of visible light, but PGO also fails to achieve significant NIR shielding and insulation. So, in this respect, PATO will be more capable than PGO.
[50].
In addition to the dimmer-controlled transparency modulation, the addition of ATO also helps in NIR shielding to reduce burden of cooling and air conditioning in tropical climates
[44]. A series of parameters including ATO doping, composite hydrogel thickness and nanoparticle content were studied and optimized. Researchers feel that the experimental results of the study will provide further research direction for development of ideal passive smart glass
[51].
They believe that this new generation of autonomous passive smart windows can be used to adapt to solar modulation.
2.1.4. PNIPAm/VO2 Nanocomposite Hydrogel
Although vanadium dioxide is a hot spot in the areas of color-changed materials
[31], it has low solar energy modulation (Δ
Tsol) and low luminous transmittance (
Tlum) and high phase change temperature
[50].
Traditional hydrogel smart windows have higher modulation efficiency in visible light wavelengths, while light modulation efficiency in near-infrared wavelengths is lower. To improve the solar modulation efficiency of smart hydrogel windows, researchers combined organic and inorganic thermochromic materials to prepare organic/inorganic composite thermochromic hydrogels
[52]. Hydrophilic and hydrophobic phase transition of PNIPAm at 30 °C mainly controls the light emission modulation
[53], while the monoclinic phase transition of VO
2 nanoparticles at 68 °C mainly controls infrared modulation
[54]. The formation mechanism of the layered VO
2/hydrogel hybrid film is shown in
Figure 6a. In 2015, Long Yi’s research group dispersed VO
2 in PNIPAm hydrogel and prepared an organic/inorganic hybrid smart window with high visible light transmittance when the temperature was lower than phase transition temperature
[51]. Organic/inorganic hybrid smart windows also have a high solar modulation efficiency, which is more than twice that of the traditional VO
2 thermochromic smart windows
[55].
Figure 6b shows the transmittance of the hybrid smart window before and after the phase change.
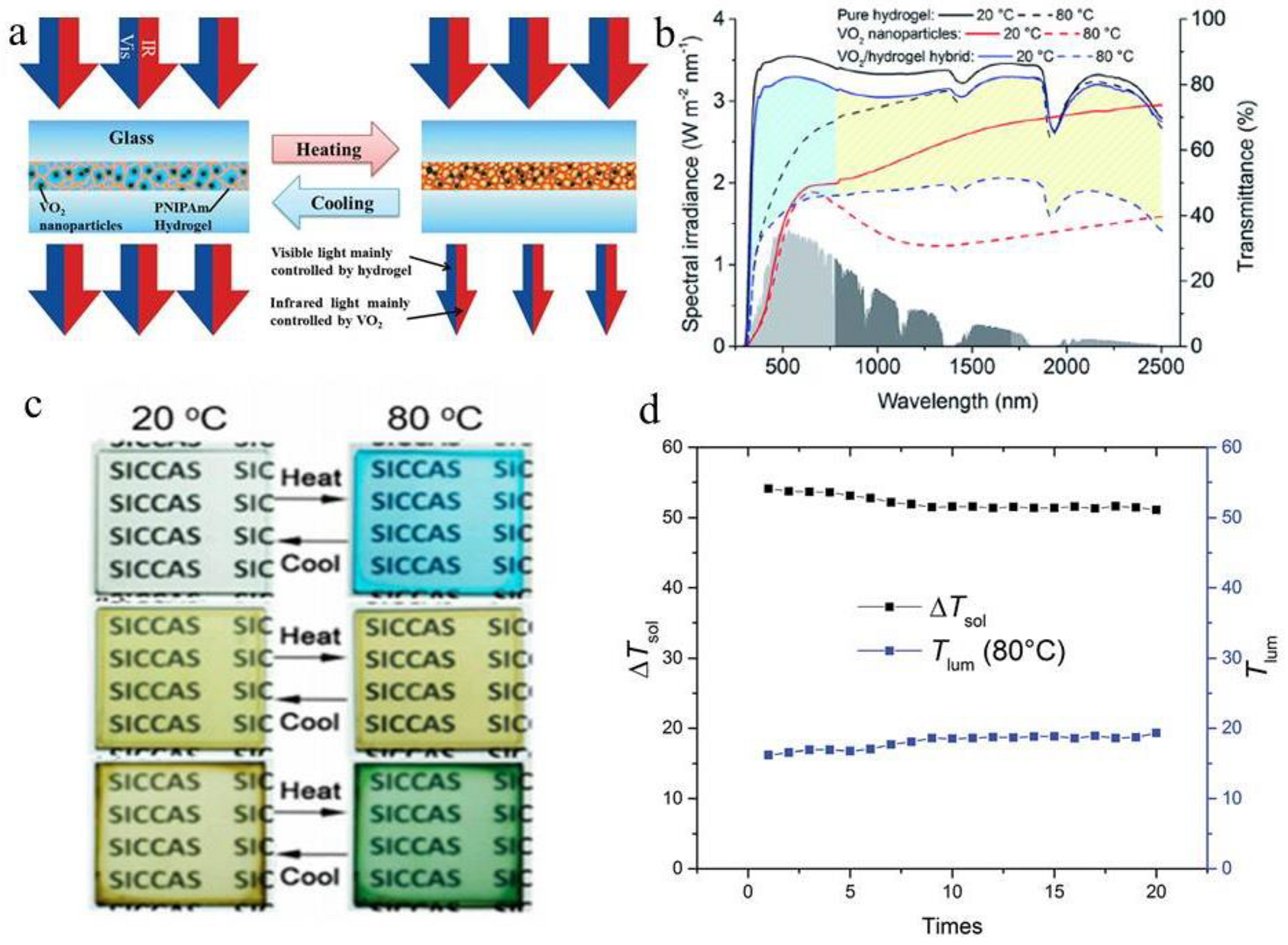
Figure 6. (
a) Sunlight regulation mechanism of VO
2/ hydrogel composites. (
b) UV-Vis spectra of pure hydrogels, pure VO
2 and VO
2/ hydrogel complexes
[51]. (
c) Films Photographs based on the pure IL-Ni-Cl complex (above row), VO
2 nanoparticles (intermediate row) and VO
2/IL-Ni-Cl composite (bottom row) at 20 °C (left column) and 80 °C (right column), respectively. The IL-Ni-Cl complex and VO
2/IL-Ni-Cl composite have significant temperature-responsive color changes. (
d) Durability test of composite hydrogels with the thickness of 100 μm from 20 °C to 80 °C.
[52].
In another work, the Jin׳s team combined VO
2 nanoparticles with thermochromic ionic liquids, which usually increase the absorbance at 650–750 nm when heated, instead of increasing the absorbance over the entire visible light range like hydrogels. The VO
2/IL-Ni-Cl composite has excellent optical regulatory properties (Δ
Tsol of 26.5%) and maintains good transparency (
Tlum of 50%). More interestingly, these films present significant color changes from brown (20 °C) to green (80 °C), which are considered synergistic of color variations in pure VO
2 and pure IL-Ni-Cl films (
Figure 6c). The proposed method provides an alternative for simultaneously improving the unfavorable brown-yellow color of VO
2 and achieving good solar modulation. Then they were applied to a ligand exchange thermochromic system based on cobalt (II) and nickel (II) (Co-based ligand exchange thermochromic system and Ni-based ligand exchange thermochromic system) and obtained similar results
[51].
The VO
2/hydrogel composite maintains good reversibility during the durability test.
Tlum and Δ
Tsol are relatively stable at 80 °C, and there is almost no change in 20 cycles of measurement. This shows that in actual smart window applications, as long as good sealing is achieved and moisture evaporation is prevented, the performance stability should be reliable (
Figure 6d)
[55].
The composite material proposed by the researchers has unprecedented excellent thermochromic properties, opening a new research direction for the field of thermochromic smart windows in the future.
2.1.5. Graphene Oxide (GO)/PNIPAm Nanocomposite Hydrogel
Regarding the bilayer structure of the hydrogel, mechanical properties of poly (N,N-dimethylacetamide) PDMAA and PNIPAm are studied respectively. Figure 7a illustrates the composition and polymerization process of a nanocomposite (NC) hydrogel based on graphene oxide (GO).
The monomers (DMAA and NIPAM), dissolved in GO dispersion separately, cannot only react with a crosslinking agent to form a covalent bond but also has a noncovalent bond with the clay due to rich amide groups. Thus, these monomers tend to polymerize next to the clay plate to form noncovalent bonds, as a result, enhancing the mechanical properties of the hydrogel
[55]. There are active groups on GO sheets such as –COOH and –OH to easily disperse in water, which facilitates the consistency between polymers and GO sheets in the hydrogel system. The internal morphology of the individual PDMAA and PNIPAm hydrogels was shown by SEM (
Figure 7b,c)
[55]. Both hydrogel networks have a nearly uniform aperture, while the GO is well dispersed in the hydrogel without any aggregation, suggesting that the gel isotropic structure has uniform mechanical properties
[56].
In 2014
[57], Chou prepared GO/PNIPAm thermochromic material using GO dispersion as photothermal conversion material. Subsequent tests showed that at 30 °C (<LCST), PNIPAm with GO dispersion could still undergo a phase transition in a relatively short period
[56]; however, pure PNIPAm hydrogel smart windows could not complete phase transition (
Figure 7d,e). The addition of GO is beneficial to improve the practical application of PNIPAm hydrogel in the field of smart windows
[58].
Figure 7. (
a) Schematic illustration of GO-based NC hydrogel components. (
b) SEM image of GO-based NC PDMAA hydrogel. (
c) SEM image of GO-based NC PNIPAm hydrogel. The scale bars are 10 μm. 30 °C Changes of light transmittance between PNIPAm/GO composite hydrogel (left) and PNIPAm hydrogel (right) (
d) before thermal radiation; (
e) after thermal radiation
[59].
Recent strategies of the fabrication of thermochromic hydrogel smart windows, including their name, function, thermochromic properties and characteristics are summarized in Table 2. Noncovalent interactions responding to temperatures, such as precise intermolecular hydrogen bonding control between polymer chains and water molecules, could optimize the performance of hydrogel smart windows. The pure hydrogel smart windows system is easy to fabricate with excellent solar modulating ability. With the addition of IR regulating/blocking materials, the solar modulating ability of thermochromic hydrogel smart windows was further enhanced. By adding photothermal nanoparticles (ATO, GO), the response speed of smart windows was further improved. At the same time, thermochromic host–guest interactions of hybrid hydrogels were fabricated to improve mechanical stability.
Table 2. Thermochromic performance of various hydrogel smart windows.
Category |
Materials |
Tlum (%) |
∆Tsol (%) |
τc (°C) |
Characteristic |
Ref. |
Single function hydrogel |
PNIPAm |
70.7 |
25. |
32 |
Conventional thermochromic hydrogel with excellent Tlum, ∆Tsol and suitable τc. |
Zhou et al., 2014 [21] |
Regular the solar modulation ability |
PNIPAm-VO2 PNIPAm/VO2@ SiO2 |
62.6 |
34.7 |
– |
By enhancing the IR regulating ability, the ∆Tsol was increased. |
Zhou et al., 2015 [50] |
38.4 |
62.7 |
40 |
Wang et al., 2018 [23] |
Photo-thermochromic |
ATO/PNIPAm GO/PNIPAm |
62.7 |
35.7 |
– |
High absorbance materials were added to increase the response speed of thermochromic hydrogels. |
Lee et al., 2017 [54] |
– |
58.2 |
– |
Chou et al., 2017 [57] |
“–” means data not available.
2.2. Hydroxypropyl Cellulose (HPC) Based Thermochromic Materials Smart Windows
Thermochromic materials are the most cost-effective smart windows. Compared with inorganic VO
2 materials, organic smart materials have higher solar energy modulation (Δ
Tsol) and luminous transmittance (
Tlum)
[60].
In 1998, Watanabe et al. dispersed hydroxypropyl cellulose in 5% sodium chloride aqueous solution
[59], obtained an intelligent window with the area of 1 m
2 and found that its cyclic stability and strong optical properties were superior
[59]. In 2001, Schneider and See both blended hydroxypropyl cellulose with hydroxyethyl cellulose and dispersed it to gum
[44][60], which not only inhibited water volatilization but also improved optical properties
[44].
In 2016, Long reported green and new organic thermochromic materials hydroxypropyl cellulose
[61]. With addition of sodium chloride (NaCl) from 0.5% (wt.%) to 5% (wt.%), (lower critical solution temperature) LCST decreased from 42 °C to 30 °C
[21]. From morphology changes of LCST and freeze-dried samples, it can be seen that the phase transformation of hydroxypropyl cellulose hydrogels is caused by the different solubility of polymers in water
[62]. The fine-tuned recipe could afford an outstanding solar modulating ability (Δ
Tsol) of 25.7% and a high averaged
Tlum of 67.4% with an LCST of 38 °C
[63].
When temperature is heated from 20 °C to 44 °C, the visible light transmittance of HPC decreases significantly, while the change of IR
[64] can be ignored (
Figure 8a). This reflects change of solution from transparent at 20 °C to translucent at 42 °C (
Figure 8b). It is worth noting that the transmissivity decreases around 1430 nm and 1930 nm at both high and low temperatures, as a result of absorption of water at these two wavelengths
[65]. When temperature rises to 50 °C, the visible and infrared transmittance of the solution decreases significantly, and the opacity of the solution also increases
[26]. When temperature rises to 60 °C and 70 °C, it becomes completely opaque while all transmissions at 250~2500 nm are blocked. Transparency is restored. within 1 min after the heater is removed.
The difference of the HPC structure at 20 °C and 80 °C is shown in Figure 8c. At room temperature, the elongation and homogeneity of polymer particles are stronger. However, at 80 °C, the length of polymer fibers decreases sharply to about 4–5 μm, with a large shrinkage pore size (Figure 8d).
This entry is adapted from the peer-reviewed paper 10.3390/molecules27051638