Transition metal-catalyzed carbonylation reactions have emerged as one of the most relevant synthetic approaches for the preparation of carbonyl-containing molecules. The most commonly used protocol for the insertion of a carbonyl moiety is the use of carbon monoxide (CO) but, due to its toxic and explosive nature, this process is not suitable at an industrial scale. The chemistry of CO surrogates has received large attention as a way to use less expensive and more environmentally friendly methods. Among the various CO surrogates, N,N-dimethylformamide (DMF) has been paid greater attention due to its low cost and easy availability.
1. Introduction
The carbonyl functional group is very common in both natural and synthetic organic compounds: on the one hand, its chemical reactivity often plays a crucial role in several biological processes
[1]; on the other, its ability as a hydrogen-bonding acceptor allows for appealing applications in supramolecular chemistry
[2][3]. In light of these considerations, the development of efficient synthetic methods for the preparation of carbonyl-containing compounds has long attracted the attention of organic chemists all over the world.
One of the most common approach for introducing carbonyl moieties is represented by transition metal-catalyzed carbonylation, i.e., reactions involving carbon monoxide (CO) as a source of the carbonyl group. From the first pioneering study on Pd-catalyzed carbonylation reactions, reported by Heck et al. in 1974
[4], several transition metal species (in particular palladium, rhodium, iridium, nickel, ruthenium, iron and cobalt) have been successfully applied as catalysts in the synthesis of countless carbonyl-functionalized molecules by carbonylation reactions involving CO as a C
1-carbon source
[5][6].
Although some examples of carbonylative protocols have even been applied on industrial scale (e.g., in the industrial synthesis of acetic acid by rhodium-catalyzed
[7] or iridium-catalyzed
[8] carbonylation of MeOH), one of the major drawbacks of these reactions is the difficulty in the handling, storage and transport of carbon monoxide, a flammable and toxic greenhouse gas. Therefore, the development of transition metal-catalyzed carbonylation protocols involving the use of CO surrogates, rather than CO gas, is a crucial aspect to address this challenge in the context of a more sustainable chemistry.
The CO surrogates are species able of producing in-situ carbon monoxide, as a result of suitable chemical reactions; in this way, toxic CO is generated and directly consumed in a closed reaction flask, thus avoiding the requirement of high-pressure equipment and minimizing its exposure to operators
[9][10][11]. Thanks to these advantages, the application of CO surrogates in carbonylation reactions has known significant advances in the last years, becoming an attractive area of research for synthetic organic chemists. The most common CO surrogates are metal carbonyl complexes
[12], formic acid
[13], formates
[14] and formaldehyde
[15], although studies involving the use of oxalic acid
[16], carbon dioxide
[17], chloroform
[18] and so on have been also reported.
In this context,
N,
N-dimethylformamide (DMF) has recently found space as an appealing CO surrogate in transition metal-catalyzed carbonylation reactions. Compared with other CO surrogates—such as metal carbonyl complexes, whose use in stoichiometric amounts is problematic on a large scale as it may cause serious environmental pollution, or formaldehyde, whose manipulation is far from trivial—DMF is a cheap and easily accessible compound, typically used as a polar aprotic solvent in several organic reactions, easy to manipulate and also compatible for scale-up (
Scheme 1a).
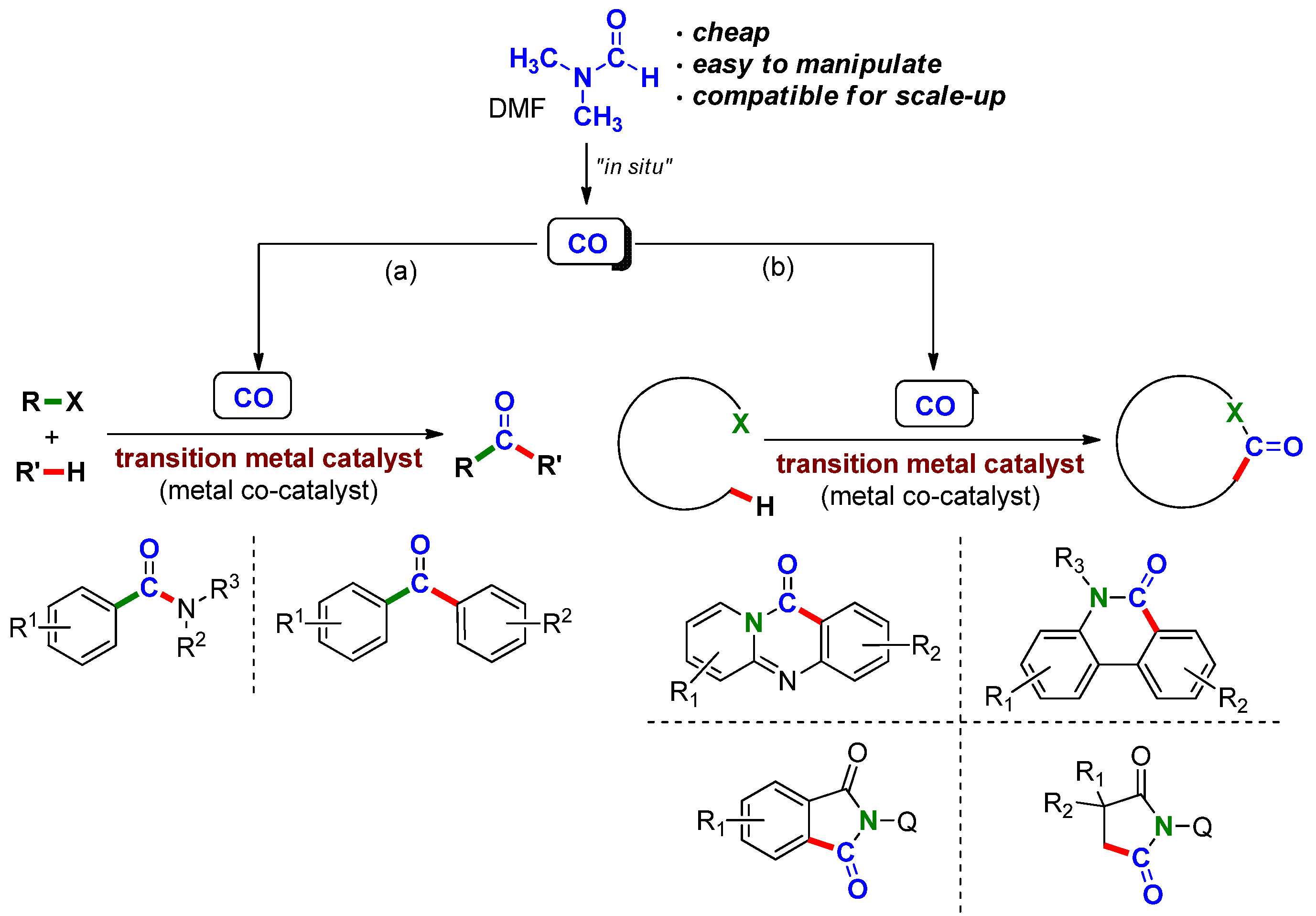
Scheme 1. Transition metal-catalyzed carbonylation reactions involving DMF as CO surrogate: (a) general scheme for the synthesis of carbonyl-containing molecules; (b) application to the synthesis of heterocycles.
Transition metal-catalyzed carbonylation reactions have acquired a prominent role in the field of the organic chemistry also for the synthesis of a multitude of useful carbonyl-containing heterocycles; in this context, we recently reported the application of cyclocarbonylative Sonogashira
[19][20][21][22] and silylcarbocyclization reactions
[23][24][25] for the synthesis of several classes of oxygen- and nitrogen-containing heterocycles. However, despite many advancements have been made in order to improve the feasibility and sustainability of these processes, the use of carbon monoxide represents a vexing problem which limits the widespread use of cyclocarbonylative reactions to some degree. The application of CO surrogates allowed to overcome this problem: in the last ten years, various carbonylative synthesis of heterocycles involving diverse CO surrogates have been successfully developed
[26]. Thanks to the above-mentioned advantages, it should not be surprising that DMF is recently also finding success for the synthesis of heterocycles (
Scheme 1b).
The application of DMF as a CO surrogate in metal-catalyzed carbonylation reactions has been partially described, to date, only as part of more general reviews, focusing on the use of DMF as a multipurpose building block in several classes of reactions
[27] in carbonylation protocols involving diverse CO surrogates
[6] or in the carbonylative synthesis of heterocycles based on several classes of CO surrogates
[26]. However, in all these reports we found a pure description of the experimental conditions without any critical discussion of both advantages and disadvantages. Therefore, a complete overview only focused on transition metal-catalyzed carbonylations involving DMF as a CO surrogate, giving not only a general state of the art of these reactions for the preparation of carbonyl-containing molecules but also an in-depth discussion of their application in the synthesis of heterocycles, is still missing.
2. Synthesis of Heterocycles by Transition Metal-Catalyzed Carbonylation Reactions Involving DMF as a CO Surrogate
2.1. Synthesis of Heterocycles by Palladium-Catalyzed Carbonylation Involving DMF as a CO Surrogate
Most of the carbonylative protocols involving DMF as a CO surrogate were carried out in the presence of a palladium catalyst. Although the aforementioned studies were only applied to the synthesis of acyclic compounds, they well showed the central role played by palladium species in promoting these processes. Therefore, it should not be surprising that most of the synthesis of heterocycles by cyclocarbonylative reactions involving DMF as a CO surrogate reported to date are based on palladium catalysts.
The first study was described in 2015 by Wu and co-workers
[28]: a palladium-catalyzed carbonylative cyclization of
N-arylpyridin-2-amines
37 by C−H activation/annulation using DMF as a CO surrogate, affording quinazolinones
38 as the final products, which are typical structural motif in many pharmaceuticals and agrochemicals. Under optimized conditions, reactions were carried out by using Pd(OAc)
2 (5–10 mol%) as the catalytic system under ligandless conditions, potassium persulfate (2–3 equiv.) as the oxidant, DMF acting as both reagent and solvent and trifluoroacetic acid as the co-solvent, at 140 °C under an O
2 atmosphere (
Scheme 2). Interestingly, some formamide analogues of DMF were also tested as an alternative source of CO, but only low yields of the resulting quinazolinones products were obtained. Concerning the substrate scope,
N-arylpyridin-2-amines
37 bearing several functional groups on the benzene ring have been tested. Electron-donating substituents (i.e., methyl, phenyl, benzyloxy, dimethylamino and methylthio), as well as electron-withdrawing groups (like trifluoromethoxy and acetyl) were well tolerated, and the corresponding products were obtained in good to excellent yields. More interestingly, substrates bearing in the
para,
meta and/or
ortho positions one or more halogen atoms, which are typically reactive in many palladium-catalyzed reactions, including the above described carbonylation reactions involving the use of DMF as a CO surrogate, were also compatible with the developed experimental conditions, affording the related quinazolinones products
38 in good yields.
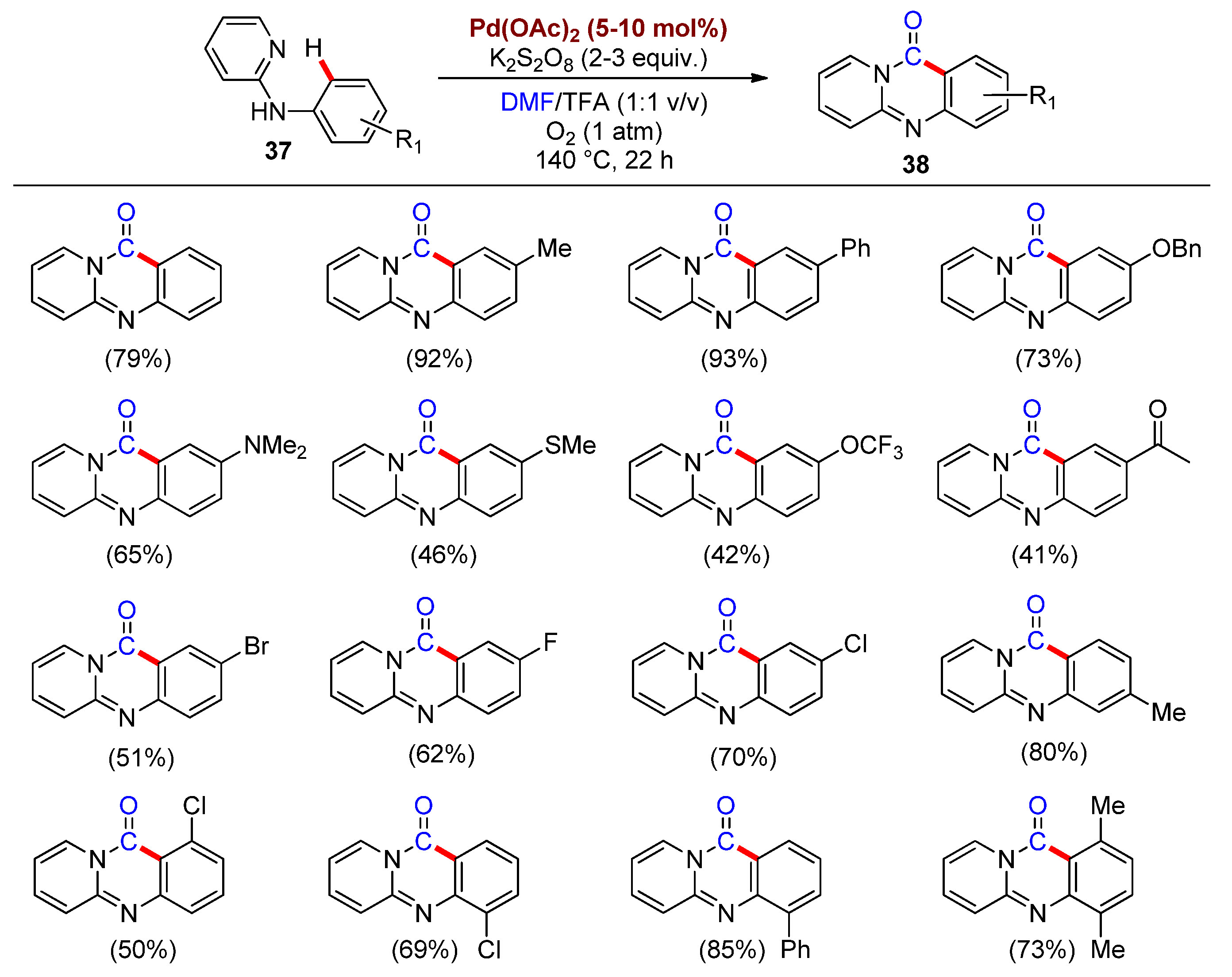
This entry is adapted from the peer-reviewed paper 10.3390/catal11121531