Barnase is an extracellular ribonuclease secreted by Bacillus amyloliquefaciens that was originally studied as a small stable enzyme with robust folding. The identification of barnase intracellular inhibitor barstar led to the discovery of an incredibly strong protein-protein interaction. Together, barnase and barstar provide a fully genetically encoded toxin-antitoxin pair having an extremely low dissociation constant. Moreover, compared to other dimerization systems, the barnase-barstar module provides the exact one-to-one ratio of the complex components and possesses high stability of each component in a complex and high solubility in aqueous solutions without self-aggregation. The unique properties of barnase and barstar allow the application of this pair for the engineering of different variants of targeted anticancer compounds and cytotoxic supramolecular complexes. Using barnase in suicide gene therapy has also found its niche in anticancer therapy.
1. Introduction
Barnase is an extracellular ribonuclease (RNase) produced by
Bacillus amyloliquefaciens as an active proenzyme, processed by the removal of the amino-terminal signal peptide and secreted into the extracellular space as a single-chain 110 amino acid protein. In this bacterial species, barstar, a specific intracellular inhibitor of barnase, is produced. Barstar tightly binds to barnase and thereby inhibits its intracellular enzymatic activity and protects host cells from the damaging effect of this RNase
[1]. Barnase is a small single-chain protein that is known for its high stability and favorable biochemical features, namely the lack of disulfide bonds, post-translational modifications, divalent cations, or other non-peptide components required for its function
[1].
The extracellular ribonuclease produced by
Bacillus amyloliquefaciens (
Bacillus subtilis strain H) was first reported in 1958 by Nishimura and Nomura
[2]. The barnase activity was estimated in 1966 to be 2.0 × 10
6 units/mg, and in 1967 the barnase cytoplasmic inhibitor, barstar, was purified and its interaction with barnase was shown to be resistant to urea, salt, or concentration change or heating of sulfhydryl reagents
[3].
Both barnase and barstar were purified on a large scale as homogenous products from
Bacillus amyloliquefaciens in 1972
[4][5], and before the establishment of molecular cloning, it required complex culturing conditions optimization, the use of a 1100-L custom-made fermenter and management of 2200 L of sorbent-medium mixtures. As a result of this laborious work, the barnase amino acid composition and sequence were estimated using hydrolysis and peptide analysis
[6]. What is more interesting, the authors could further use barnase for affinity chromatography to purify barstar
[5] and study the stoichiometry of the complex. It was shown that barnase and barstar form a one-to-one complex which dissociates only in the presence of strongly dissociating agents such as 5 M of guanidine HCl or 0.1% sodium dodecyl sulfate
[7], suggesting that barnase-barstar interaction is extremely stable, but it is not based on covalent bonds formation. It was later shown that barstar covers the barnase active site, thus protecting the bacterial cell from ribonuclease that can accidentally be synthesized on free ribosomes in the cytoplasm, and the dissociation constant of the barnase-barstar complex is estimated to be 10
−14–10
−13 M
[7][8]. In a series of works, it was estimated that the barnase-barstar pair is highly soluble in aqueous solutions, possesses high stability both in a complex and separately, and the members of the pair have no tendency to self-aggregation
[9][10].
The cloning of the barnase gene was quite challenging, as in the absence of barstar the expression of barnase from a plasmid kills a cell, so the pioneer works were made with inactivated barnase, the gene of which was mutated in
Bacillus amyloliquefaciens before any other manipulations
[11][12]. The cloning of barstar and encoding of both barstar and barnase on a single plasmid enabled the production of active barnase in
Escherichia coli [13]. From that time the barnase and its molecular twin Binase were used in numerous applications including folding studies
[14][15], enzymatic activity investigation
[16], protein-protein interactions investigation
[17][18], positive selection of cloned inserts
[19][20], providing male and female sterility in plants
[21][22], crop defense system construction
[23], specific cell depletion
[24], virus elimination
[25][26][27], and cancer cell killing
[28][29][30][31].
Barnase is capable of nonspecific RNA cleavage and, being produced in the cytoplasm or being delivered into it, can decrease cell viability. The cleavage of mRNA is a straightforward toxic mechanism that differs from the mechanisms that are implemented in conventional chemotherapy
[32], so an obtained resistance to chemotherapeutic agents is not likely to affect the effectiveness of barnase. Unlike RNase A, RNase 1, RNase 2, and angiogenin, barnase is insusceptible to the ubiquitous cytoplasmic ribonuclease inhibitor
[33]. The extremely high affinity of the barnase-barstar complex can be used in the design of self-assembling complex therapeutic and theranostic agents. The N- and C-terminals of both barnase and barstar are located far from their interaction interface (
Figure 1)
[34][35], so both proteins can be fused with other functional modules without loss of function or affinity of barnase and barstar. These properties are successfully used in anticancer agent design.
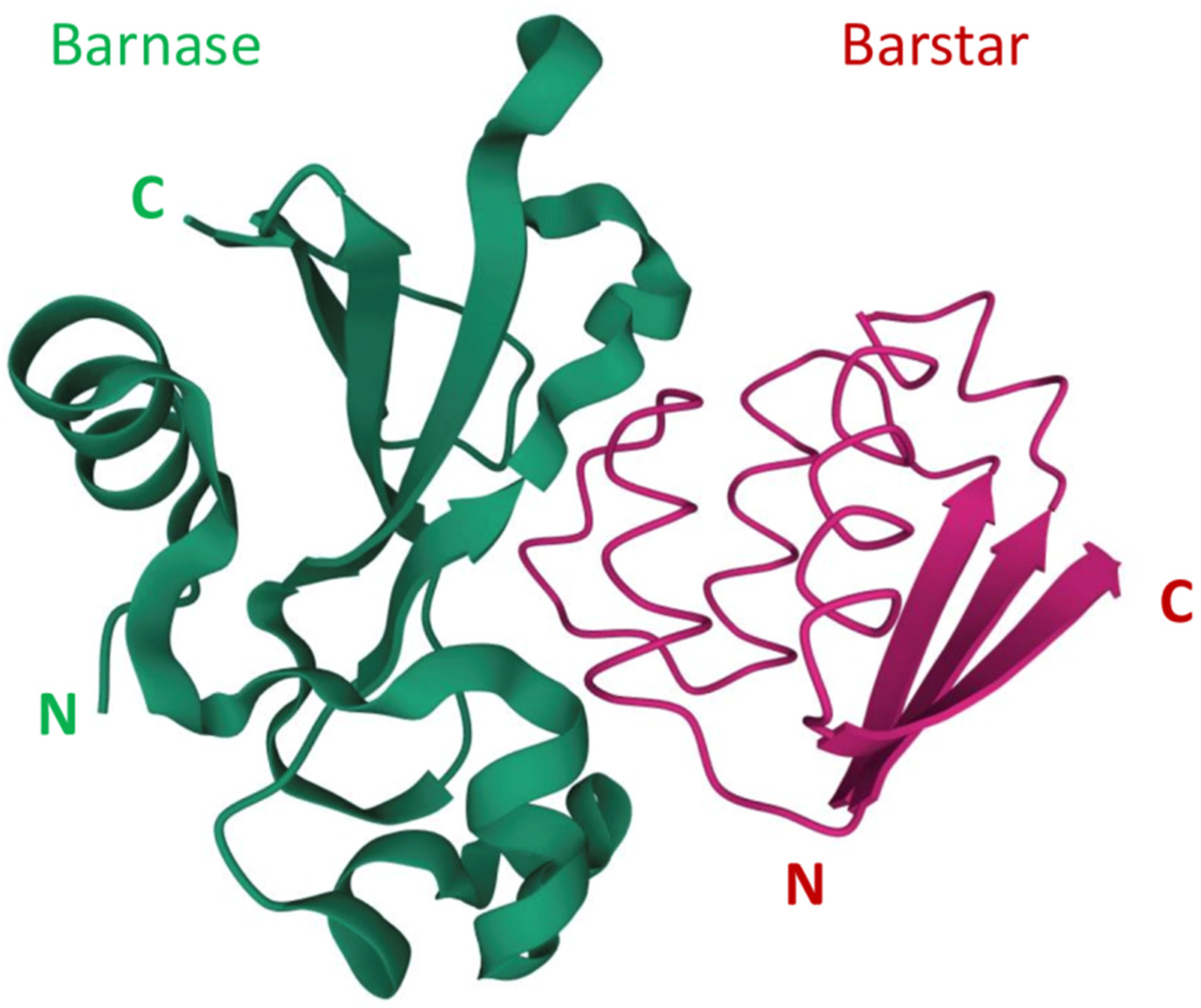
Figure 1. The barnase and barstar complex structure
[34][35]. N and C refer to N-terminus and C-terminus respectively.
2. The Use of Barnase-Barstar Interaction for Supramolecular Complexes Assembly
Besides the regulation of ribonuclease activity, the strong interaction of barnase and barstar can be used for the assembly of supramolecular complexes consisting of proteins, and nano- and microparticles. The extremely high dissociation constant of barnase-barstar complex (10
−14–10
−13 M)
[7][8] provides the formation of stable complexes that remain associated under severe protein denaturing conditions including high temperature, low pH as well as high salt and chaotropic agent (urea and guanidinium hydrochloride) concentrations
[36]. In the same study, the stability of barnase-barstar complex was compared to the commonly used assembly systems, namely, streptavidin-biotin, antibody-antigen, and protein A-immunoglobulin, and barnase-barstar was proven to be comparable with these systems or to surpass them due to the combination of its high resistance to severe chemical perturbation and unique advantages offered by genetic engineering of this entirely protein-based system.
The use of barnase and barstar interaction enables the assembly of multimeric targeted proteins. The use of barnase-barstar modules fused to 4D5scFv made it possible to assemble di- and trimeric complexes with increased avidity and molecular weight (81 kDa and 132 kDa versus 30 kDa of 4D5scFv monomer)
[9]. Barnase and barstar were used as heterodimerising modules in a novel anticancer DNA vaccine
[37][38], bringing together two units composed of an antigen presenting a cell targeting motif and an immunogenic motif. In combination with the easy cloning strategy, the heterodimeric barnase-barstar vaccine molecule provided a flexible platform for development of novel DNA vaccines
[38].
The same strategy can be used to couple targeting and effector modules for cancer therapy. 4D5-dibarnase served as a targeting moiety to deliver heat shock protein Hsp70 to cancer cells through fused barstar, the resulting bifunctional complex efficiently binding to HER2-overexpressing cells and recruiting NK cells to them
[39]. In recent work barnase and barstar provided the tumor targeting and regulation of CAR-T (chimeric antigen receptors) cells in anticancer therapy
[40]. The designed chimeric receptor containing barstar could bind to tumor-targeted barnase instead of a tumor antigen itself. The intermediate molecule enabled the precise control of CAR-T cells activity towards cancer cells and provided the basis of a universal CAR-T cells system design.
The benefits of barnase-barstar self-assembly are most obvious for recombinant proteins, as these modules are fully genetically encoded. However, to date, the most diverse and sophisticated methods involving these modules have been developed in nanoparticles design. Convenient methods for nanoparticles modification based on physical adsorption and chemical conjugation have several disadvantages including the uncontrollable orientation and steric availability of biomolecules, the loss of components activity, and poor reproducibility. The use of complementary proteins allows for sufficient and controllable supramolecular complexes assembly. The process of assembly does not require complex equipment and reagents, so it can be useful both for diagnostic and clinical applications. The functional modules can be mixed in a test tube or perform self-assembly within an organism after consecutive injections.
Barnase and barstar can be used as “a molecular glue” in supramolecular complexes assembly. Usually, one of the named proteins is linked to a nanoparticle’s surface with the help of chemical conjugation, and its partner is genetically fused to a functional protein, for example, a single-chain antibody. The final step of nanoparticle modification is usually done via simple mixing of components in a test tube. The barnase-barstar linker provides the addition of a single molecule of a functional protein to each linker molecule on the surface of a nanoparticle (Figure 2).
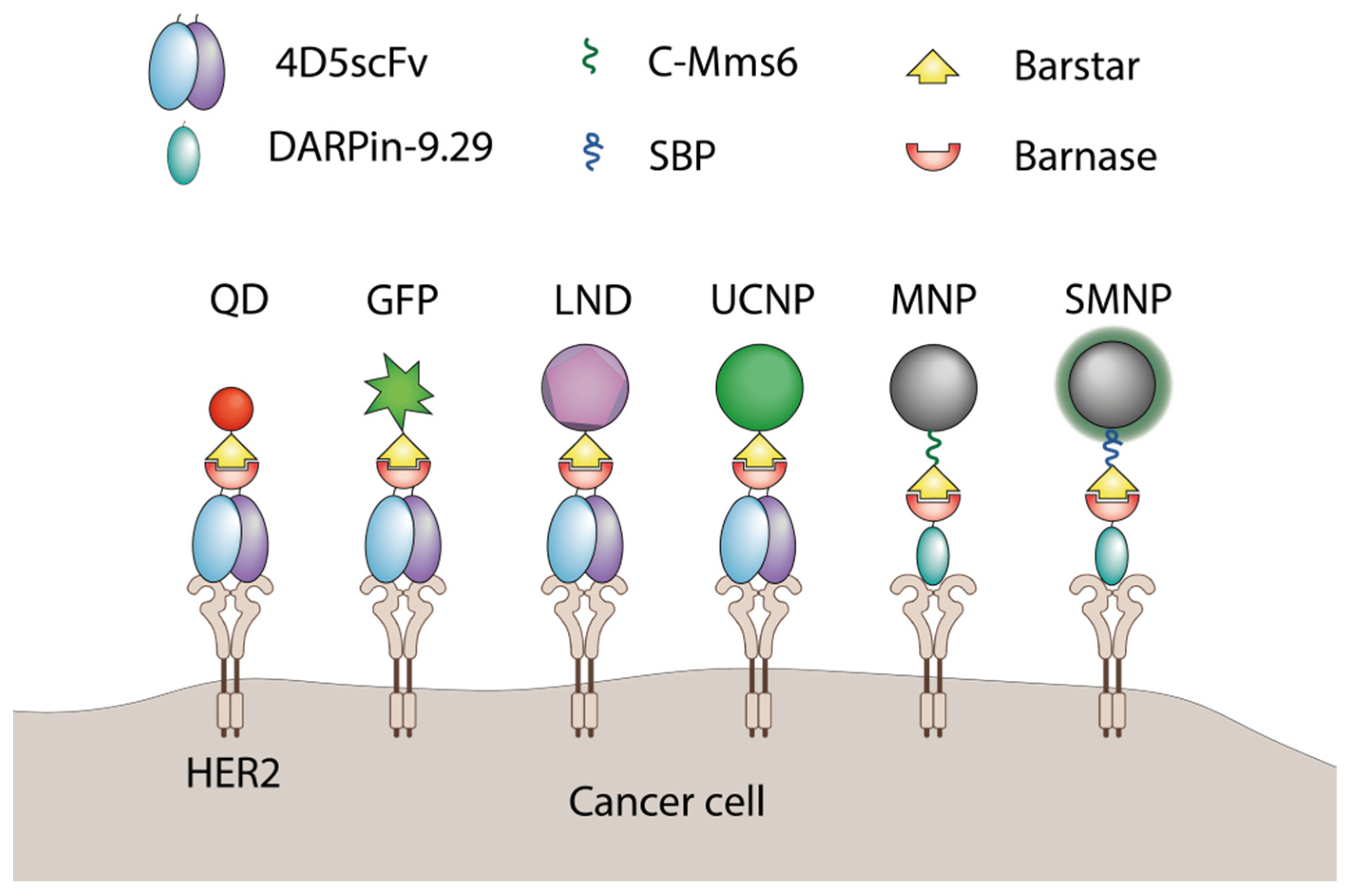
Figure 2. The application of barnase and barstar interaction in supramolecular complexes assembly. QD—quantum dots
[41], GFP—green fluorescent protein
[42], LND—luminescent nanodiamond
[43], UCNP—upconversion nanoparticle
[44], MNP—magnetic nanoparticle
[45], SMNP—SiO
2-coated nanoparticles
[46], C-Mms6—magnetite-binding peptide
[45], SBP—SiO
2-binding peptide
[46].
This strategy was used for various types of nanoagents. The luminescent upconversion nanoparticles were designed for breast cancer diagnostics. The particles were stabilized with amphiphilic polymer and modified with barstar, which provided the attachment of a 4D5 single-chain antibody through genetically fused barnase. The resulting UCNP-Barsar:Barnase-scFv4D5 complexes were tested in an optical phantom consisting of HER2-positive SK-BR-3 cells covered by mammary gland tissue. The modified UCNP-exposed SK-BR-3 cells could be detected at a depth of 4 mm with the optical contrast as high as 10:1 benchmarked against a negative control cell line
[44].
Quantum dots were also successfully modified using this system. Nanoparticles were chemically linked to either barstar or barnase and tumor targeting was achieved due to fuse proteins of a coupling partner with scFv specific for HER1 or HER2
[41]. The resulting self-assembling nanoparticles specifically bound to cancer cells expressing respective surface markers in vitro and HER2-specific nanoparticles accumulated in xenograft tumors significantly better than the untargeted nanoparticles
[47].
In addition to coupling therapeutic proteins to nanoparticles, barnase and barstar can facilitate the assembly of various objects referring to the nano- and microscale themselves. This was demonstrated for fluorescent nanoparticles bound to the surface of magnetic nanoparticles. The interaction of barnase and barstar was strong enough to provide a sustainable complex formation resistant to extreme conditions including heating, low pH, and high concentrations of chaotropic agents
[36]. This interface was accompanied with a HER2-targeting 4D5 module through the same barnase-barstar interaction resulting in trifunctional nanostructures including targeting a single-chain antibody, magnetic nanoparticle, and fluorescent nanoparticles. All modules retained their features in the resulting construct, providing efficient magnetic cell sorting and visualization
[48].
A similar modification method was used for luminescent nanodiamonds (LND). These particles were chemically conjugated with barstar, which provided LND colloid stability in aqueous solutions. These particles were further coupled with either barnase-GFP fuse protein or gold nanoparticles linked to barnase
[43]. In all cases the barnase-barstar “molecular glue” technique provided stable complexes retaining their functional features that could be obtained by simply mixing the components in a test tube.
The culmination of barnase-barstar nanoparticles modification technology development is the use of solid phase-binding peptides that help to avoid chemical conjugation at all. Either barnase or barstar can be genetically fused to a surface-binding peptide, which will help to modify a nanoparticle without chemical conjugation, and the partner protein will provide a coupling of targeting or other functional components to the particle. This approach allows one both to achieve the desired orientation of the binding module and to assemble the targeting modules according to the principle of a construction kit. It was first demonstrated for silicon nanoparticles, modified with barstar fused to SiO
2-binding peptide (SBP-barstar). As HER2-targeting modules, the fuses of barnase with single-chain 4D5 antibody or scaffold protein DARPin-9.29 were used. These proteins provided the assembly of the outer layer of nanoparticles in a solution without using conjugation or on the surface of cancer cells (the pretargeting strategy). In the last case, the targeted proteins were first delivered to the cells and then the nanoparticles were added subsequently
[46].
By replacing SBP with magnetite-binding peptide C-Mms6, the system was tailored to magnetic nanoparticles modification. C-Mms6 is a fragment of the protein which is used by magnetotactic bacteria for magnetosomes building. Magnetotactic bacteria can feel the Earth’s magnetic field due to special membrane organelles containing magnetic nanoparticles. The biomineralization of these particles is controlled by a complex of special proteins including Mms6 protein.
3. Conclusions
Barnase and barstar are notable both for biological activity and their physicochemical features. Their tight and strong interaction provides experimental biology with one more bio-compatible type of interaction in addition to antigen-antibody, antibody Fc-protein A/G, and streptavidin/avidin-biotin. The barnase-barstar pair represents a versatile bioconjugation platform for the design, production, and characterization of various supramolecular complexes. High-affinity interaction of barnase-barstar has already been used in the construction of innovative cancer therapy for multivalency introduction, binding of variable targeting and effector modules, and nanoparticles functionalization providing proper orientation of the targeting module. The use of self-assembling modules provides an opportunity to easily change the specificity or the effector mechanism of a complex, which provides a flexible platform for new anticancer agents design. Using barnase-barstar in pretargeting drug delivery also has great potential: first, in contrast to existing pretargeting systems based on noncovalent interaction, barnase and barstar have no endogenous inhibitors or nonspecific targets in mammals, and second, this approach is highly modular since barnase or barstar can be easily fused at the gene level with any artificial scaffold recognizing any antigens, making this approach a versatile pretargeting platform.
The ribonuclease activity of barnase is a promising source of anti-tumor agents. Cleavage of messenger RNA is a universal mechanism of cell killing, as any human cell depends on protein synthesis. The experimental data demonstrate that barnase in the form of a targeted recombinant protein that binds to the surface HER2 receptor enters the cell via receptor-mediated endocytosis and can induce apoptosis in cancer cells. Due to ribonuclease activity and the protection of virus-producing cells by barstar, barnase can also become a component of suicide gene therapy, but this potential is yet to be utilized.
One of the challenges associated with barnase-barstar-based anticancer therapy is the possible immunogenicity of these modules. Since barnase and barstar are bacterial proteins, the question about their immunogenicity arises. For some bacterial RNases (binase, RNase Sa) low immunogenicity has been shown
[49][50][51], on the other hand, in a series of works, barnase and barstar are used in DNA vaccines aimed at obtaining an immune response
[37][38]. Thus, the detailed study of the immunogenicity of barnase-barstar in anticancer therapy should be the subject of further research.
In conclusion, we can state that due to the unique features of the baranse-barstar pair, this complex has found its own niche in the field of cancer research and biotechnological methodology.
This entry is adapted from the peer-reviewed paper 10.3390/molecules26226785