Botryllus schlosseri, a colonial tunicate, which is the nearest invertebrate group to the vertebrates, is devoid of T- and B-cell-based adaptive immunity. It has unique characteristics that make it a valuable model system for studying innate immunity mechanisms: (i) a natural allogeneic transplantation phenomenon that results in either fusion or rejection; (ii) whole animal regeneration and noninflammatory resorption on a weekly basis; (iii) allogeneic resorption which is comparable to human chronic rejection. Recent studies in B. schlosseri have led to the recognition of a molecular and cellular framework underlying the innate immunity loss of tolerance to allogeneic tissues. Additionally, B. schlosseri was developed as a model for studying hematopoietic stem cell (HSC) transplantation, and it provides further insights into the similarities between the HSC niches of human and B. schlosseri.
The entry is from Botryllus schlosseri as a Unique Colonial Chordate Model for the Study and Modulation of Innate Immune Activity. August 2021Marine Drugs 19(8):454. DOI: 10.3390/md19080454
1. Introduction
B. schlosseri is an invasive marine colonial tunicate from the phylum Chordata, which can now be found all over the world
[1][2]. Tunicates were named after the gelatinous “tunic” structure that covers their body and were proposed by Charles Darwin as a fundamental clue in the evolution of vertebrates
[3]; indeed, molecular phylogenetics studies found them to be the vertebrates’ closest living invertebrate relatives
[4][5].
B. schlosseri has the ability to reproduce either sexually or asexually
[6][7]. Following fertilization, a series of classic embryonic developmental stages over a 6 day period results in a tadpole larva conceived in the sexual pathway
[6]. This larval stage is actually a major clue reflecting the close relationship to vertebrates, as it features characteristics such as a tail, notochord, neural tube, and striated musculature (
Figure 1A)
[8][5][6][9]. The swimming larva settles on a substrate within a few hours after hatching and metamorphoses into a sessile oozoid with an invertebrate-like body plan (
Figure 1B), which initiates a cyclical blastogenic process that results in the formation of a colony of genetically identical zooids and buds
[6][10]. The budding cycle takes 7 days and begins with secondary buds turning into primary buds which, at the end of the cycle, replace the previous generation of zooids, whose cells die through programmed cell death and are cleared through programed cell removal (
Figure 1C)
[11][6][12][13][14]. This cyclical budding process is mediated by stem cells, which sustain the organism throughout its life, and which are responsible for organogenesis in the asexual reproduction cycle and gametogenesis in the sexual reproduction pathway
[11][15][16][17][18][19][20].
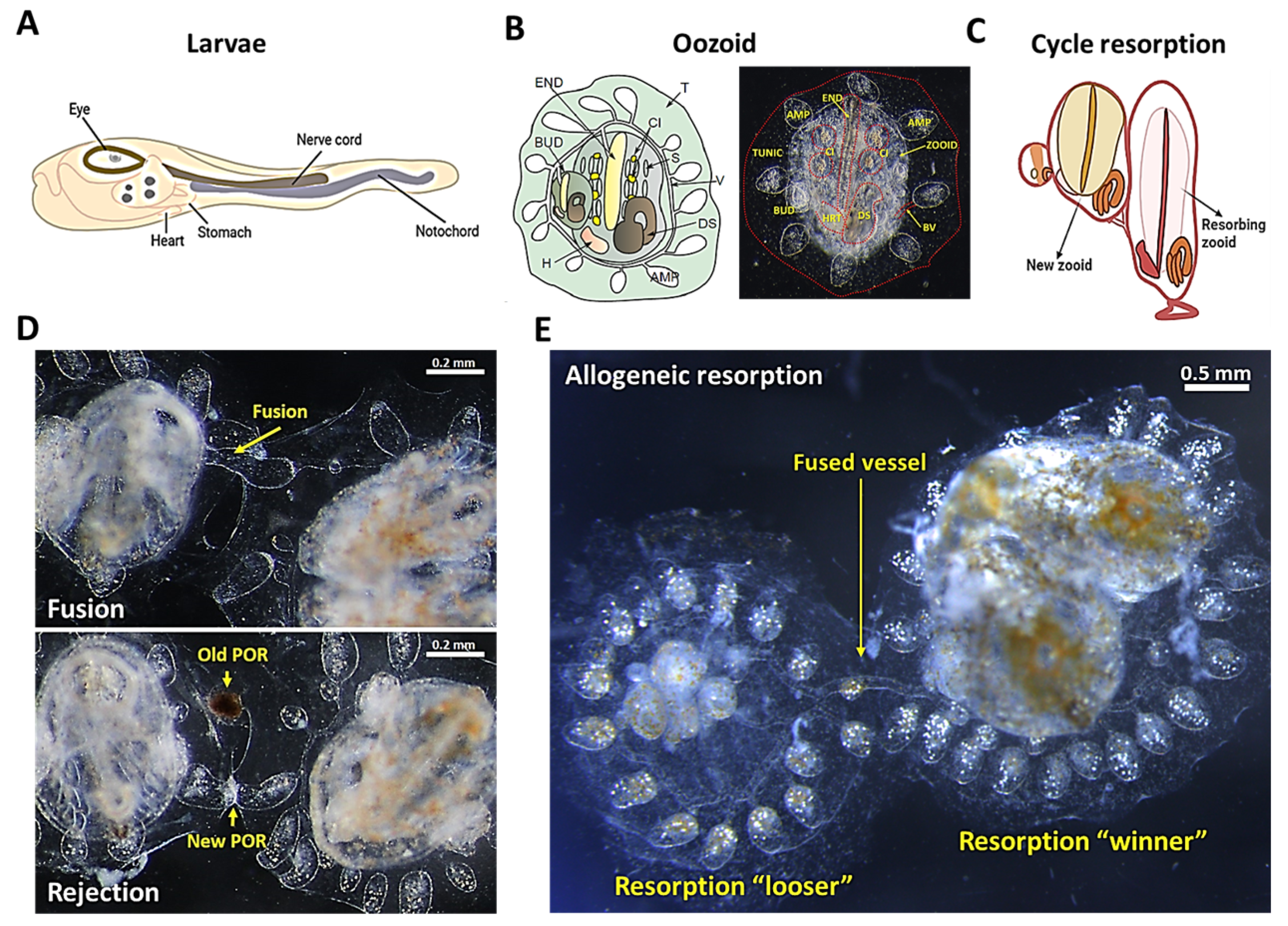
Figure 1. The anatomy of
B. schlosseri and its different levels of naturally occurring immune responses. (
A) Diagram of the tunicate larvae tadpole phase showing the nerve cord and the notochord. (
B) Diagram and live imaging of the ventral view of a zooid (Z) and primary bud (BUD), embedded within a tunic (TUN) and connected with vasculature (V), which terminates in ampullae (AMP). The zooid has a branchial sac conformed by the endostyle (END), stigmata (S), cell islands (CI), digestive system (DS), and heart (H). (
C) Diagram showing the “takeover” phase in the weekly cycle of zooid regeneration mediated by noninflammatory programmed cell removal of the resorbing old zooid. (
D) Live imaging of two
B. schlosseri colonies undergoing fusion (arrows show fused vasculature (top)) and rejection (arrows show points of rejection (POR) (bottom)). (
E) Live imaging from the allogeneic resorption process, where one colony is the “loser” (which is resorbed), while the other is the “winner”, demonstrating normal developmental stages. (
A,
C) were created using BioRender; (
B,
D) were reproduced with permission from
[11], Springer Nature Limited, Berlin, Germany, 2018; (
E) was reproduced with permission from
[21], National Academy of Sciences, 2016.
B. schlosseri has different levels of naturally occurring immune responses. When two genetically compatible colonies that share at least one allele in their
Botryllus histocompatibility factor (
BHF) gene touch, they fuse, share circulation, and form a chimera (
Figure 1D, top)
[22][23][24]. However, incompatible colonies, which do not share any allele in the
BHF gene, undergo a rejection response, creating points of rejection between the colonies with necrotic tissue (
Figure 1D, bottom)
[16][18][19][22][23][24]. Interestingly, in fused animals on a semi-compatible level, in some cases, one chimeric partner is eliminated by an inflammatory process of allogeneic resorption which is comparable to human chronic rejection (
Figure 1E)
[21][25].
Another fascinating phenomenon that takes place when two compatible colonies fuse circulation is stem-cell competition, whereby stem cells from one colony infiltrate the reproductive organs of the other colony, overtaking gametogenesis and giving rise to progeny of its own genotype; thus, the stem cells represent biological units of natural selection. Stem-cell competition is an example of a new field that evolved from studying
B. schlosseri chimeras. Cell competition in
B. schlosseri was demonstrated in an experiment that used genetic markers to track the genotype of somatic and germline tissues within a two-colony chimera, revealing the expression of only one genotype in the germline tissues of both colonies, as well as one to few genotypes in somatic cells
[16][18][19]. Follow-up studies further demonstrated that this clone takeover is mediated by stem cells
[16]. In order to translate the study of stem-cell competition to mice, tetra-chimera mice were developed by injecting three distinct colored embryonic stem cells into blastocytes, each labeled with a different fluorescent reporter
[26]. As the embryos developed, many adjacent seminiferous tubules yielded several fluorescent signals; however, only two to three different colors in each testicle remained after maturation
[27]. The interpretation suggests that, while all clones entered the genital ridges bilaterally, only one of them remained in the adult mice
[28]. In the blood-forming system, aging and disease processes have been attributed to stem-cell competition. During aging, there is an increase in the proportion of stem cells producing myeloid vs. lymphoid blood populations, which may be attributed to stem-cell competition, as observed in old versus young mice and humans
[29][30][31][32][33]. Clonal expansion and stem-cell competition were clearly shown in the stepwise progression of aberrant preleukemic clones toward leukemias
[34][35][36][37][38][39], as well as in the clonal expansion of smooth muscle cells in atherosclerosis in mice and humans
[40][41]. These are examples of how studies in
B. schlosseri have led to discoveries in mammalian development, aging, cancer, and atherosclerosis
[28].
2. Botryllus schlosseri as a Model to Study the Innate Immunity
B. schlosseri has an efficient immune system that not only fights and prevents infections, but also orchestrates histocompatibility, incompatibility, rejection, and zooid resorption processes. Interestingly, when its genome was annotated and compared with invertebrate and vertebrate genomes, it was found that numerous genes associated with immune system and hematopoiesis, including
ZBTB1,
MEFV,
DSG3,
NQ01,
NQO2, and
BHLHE40, which are involved in leukocyte development, as well as an additional set of genes that could attributed to precursors of human hematopoietic lineages, could be detected in
B. schlosseri but not in other invertebrate species or solitary tunicate species
[8].
Immune responses in
B. schlosseri are mediated by homeostatic cell turnover and the licensing of innate cytotoxic cells, which collaborate with activated phagocytes. These immune effector cells are circulating blood cells that currently exhibit phagocytic and cytotoxic cell activity. Among phagocytic cells,
B. schlosseri has a myeloid lineage that shares a large set of genes with mammalian myeloid lineages
[11]; it also has amoebocytes and large phagocytes which are morphologically more related to hemocytes of arthropods and echinoderms
[42]. These phagocytes engulf microorganisms and damaged self-cells, contain phagosomes with hydrolytic enzymes, lipids, and lipofuscins
[43], and can be subdivided into static (in the circulatory system epithelia) and mobile (circulating through the colony) populations
[44].
MCs of
B. schlosseri have been characterized as cytotoxic cells; they are the most abundant circulatory cell type and have large cytoplasmic granules containing an inactive form of phenoloxidase (PO)
[43]. Gene expression analysis has shown that they express a tunicate-specific gene repertoire and a set of genes (15%) sharing homology with vertebrate lymphocytes
[11][42].
At the molecular level, BsTLR1 is expressed in both phagocytes and MCs of
B. schlosseri as a member of the TLR receptor family, which is actively involved in self/nonself recognition
[45]. Blood circulating cells also express a gene of a type II transmembrane protein that is related to CD94 and NKR-p1 receptors of human NK cells and T lymphocytes. This protein has been found to be upregulated during the allorecognition process
[46]. Rhamnose-binding lectin (BsRBL) has been identified as an effector molecule that activates phagocytes, thus inducing the release of cytokine-like molecules that are recognized by the anti-IL1α and anti-TNFɣ antibodies
[47]. Furthermore, activated phagocytes signal through Ras-like small GTPases, MAPKs, and NF-κB networks to trigger the recognition response of foreign cells
[48].
The work in B. schlosseri has guided us to hypothesize that innate immune mechanisms during tissue maintenance, allorecognition, and regeneration are conserved and highly important for the initiation of the adaptive immune response in mammals. Therefore, this model allows the study of the orchestrating cellular and molecular processes around these immune responses, focusing on the innate immune responses. This information can then be translated to human immunity, with a particular impact on the improvement of therapeutic strategies for stem cells, tissue, and organ transplantation.
Moreover, the immune defenses of tunicates have made them a potential source of various natural drug resources with great potential for pharmacological applications. For example, the hemagglutinating activity of lectins in ascidian hemolymph has an important immune role
[49]; marine lectins have been investigated as potential antimicrobial and antiviral agents, as well as compounds with immunomodulatory and cytotoxic effects on tumor cells
[50]. Five homologous transcripts of rhamnose-binging lectins (RBLs) have been identified to enhance phagocytosis in
B. schlosseri [49]. Furthermore, components of the complement alternative pathway (C3 and Bf orthologs)
[51][52] and components of the lectin pathway (mannose-binding lectin, ficolin, and mannose-associated serine protease 1) are transcribed by MCs and associated with nonself recognition, opsonization, and clearance of microbes and apoptotic cells
[53].
The previously mentioned PO enzyme, which is degranulated and released by
B. schlosseri MCs, is a bioactive molecule with cytotoxic activity against microbial infections (i.e., yeast cells and bacterial spores), as well as nonself cells (i.e., incompatible blood)
[49][54]. MCs are also the main source of the soluble cytokine-like proinflammatory molecules IL-1-α and TNF-α, which are suggested to be released in the presence of incompatible cells and microbes
[49]. Further studies are needed to explore the drug potential of these
B. schlosseri molecules and their possible pharmacological applications.
Several other compounds with antifungal, antidiabetic, antioxidant, and antitumor potential have been identified in tunicates. More in-depth information about this topic was reviewed in
[55][56].
3. Conclusions
The advancements in research and scientific tools in the
B. schlosseri model have promoted
B. schlosseri as an interesting model to study innate immune system responses, more specifically in transplantations. One of the more prominent research tools, the
Botryllus genome project
[8], enabled analysis on the genetic and molecular levels, thus identifying the
BHF [24] on the fusion histocompatibility locus
[57]. Moreover, the genome project confirmed the location of the tunicates as the closest invertebrate group to vertebrates, whereby many immune genes are shared with mammals and their hematopoietic system
[8].
Furthermore, as mentioned above, B. schlosseri has natural occurring phenomena which resemble many basic immunological processes, such as (I) rejection as acute rejection in transplantation, (II) fusion as natural parabionts that share stem cells, (III) stem-cell competition and chimerism, (IV) natural weekly cycle of zooid resorption and new bud development, working through classical programmed cell removal and regeneration mechanisms comparable to vertebrates, and (V) allogeneic resorption as a chronic rejection process.
Moreover, there were several gene expression sets obtained during the research of the above-described processes, which enabled the search of candidate genes and pathways that affect those immune-associated processes, for example, gene sets of allogeneic resorption
[21], fusion and histocompatibility-associated genes
[24], 23 different cellular populations and endostyle
[11], and zooid regeneration and developmental processes
[6].
Taken together, the tools and advancements in B. schlosseri, along with the ability of in vivo and ex vivo cellular immune profiles, with cellular and molecular manipulations (from morpholinos to recombinant proteins), represent the foundation for future discoveries on immune activation mechanisms in a simple model, which is relevant for human immune research.
This entry is adapted from the peer-reviewed paper 10.3390/md19080454