Particulate Bound Hg (PBM) consists of all airborne particulate containing Hg, including both stable condensed and gaseous forms adsorbed on atmospheric particulate matter (PM); it is operationally sampled and quantified by pulling air through a glass fiber or a quartz filter[4]. PBM usually includes all those particles with a diameter <2.5 μm, even if its characterization depends on the pore size of the filter used for its collection[5]. The accurate dimensional characterization is then essential to estimate the dry deposition of PBM, as well as any other particulate pollutant; the particles diameters directly influence gravitational sedimentation and the PBM residence time in the atmosphere[6]. In addition, PBM chemical speciation, as well as for the other Hg forms, is fundamental to understand PBM bioavailability and therefore the effects on human .
1. Overview
Mercury (Hg) is ubiquitously distributed in the environment, naturally occurring in the Earth’s crust at average concentrations of about 0.05 mg kg−1[1]. Hg in the atmosphere is present both as gaseous forms, i.e., GEM and reactive gaseous Hg (RGM, Hg2+), and as particulate bound Hg (PBM). GEM represents the most abundant (>95%) form of Hg in the atmosphere, with high stability and long lifetime (from 0.5 to 2 years) thanks to chemical inertness[2]. Due to this long persistence, Hg is considered one of the major global environmental pollutants[3]. PBM consists of all airborne particulate containing Hg, including both stable condensed and gaseous forms adsorbed on atmospheric particulate matter (PM); it is operationally sampled and quantified by pulling air through a glass fiber or a quartz filter[4]. PBM usually includes all those particles with a diameter <2.5 μm, even if its characterization depends on the pore size of the filter used for its collection[5]. The accurate dimensional characterization is then essential to estimate the dry deposition of PBM, as well as any other particulate pollutant; the particles diameters directly influence gravitational sedimentation and the PBM residence time in the atmosphere[6]. In addition, PBM chemical speciation, as well as for the other Hg forms, is fundamental to understand PBM bioavailability and therefore the effects on human .
2. Method
PBM can be analyzed using scanning electron microscopy coupled to energy dispersive spectroscopy (SEM-EDS). The samples are metallized with a few nanometers of graphite and analyzed at a 15-kV acceleration voltage, 700 pA electron current, and at a 9–10 mm working distance. The analysis protocol is developed to standardize the measurements and quantify the particles composed of heavy metals in a faster and accurate way. Samples are previously investigated using an automatic procedure that allowed to select the standard surface area of each sample to be investigated and a suitable magnification to identify the particles. Selecting backscattered electrons images with an appropriate black and white image threshold, the program is able to identify all particles comprising the selected thresholding levels, to morphologically measure them, to perform a rapid EDS (10-s of live-time) analysis, and thus chemically classify all particles. Heavy metal particles are recognized as light grey areas using backscattered electrons images with a contrast of 60%, brightness of 5–10%, and a threshold shade of grey (between 80 and 250) able to include all particles with an average atomic number (Z), heavier than Ba (reference standard BaSO
4) (Figure 1). These methods allow the isolation of particles characterized by a high average Z, comprising (but not exclusively) those containing Hg. It has to be stressed that, on the basis of backscattered electrons (BSE) thresholder images, the dimension (equivalent circular diameter, ECD) of the identified particles is smaller than the X-ray generation volume (i.e., the volume from which X-rays are produced). We can estimate an interaction volume with a diameter of about 0.8–1.1 µm (respectively at 10–20 kV acceleration voltages) for heavy elements/compounds (density > 8 g/cm
3, i.e., Cu), and even greater (some microns) for light elements. To restrict SEM-EDS microanalyses to the volume of Hg particles, minimizing the contribution of the surrounding phases, a more detailed microanalysis (30-s of live-time) can be selectively conducted on the largest Hg-particles (i.e., ECD > 1 µm or the largest for each sample). All elements are re-calculated using a microanalysis software, which used the XPP matrix correction scheme developed by Pouchou and Pichoir
[7]. This is a Phi-Rho-Z approach which uses exponentials to describe the shape of a φ(pz) curve. The procedure is a “standard-less” semi-quantitative analysis employing pre-acquired standard materials. The monitoring of the analytical conditions (i.e., filament emission) is conducted with repeated analyses of a Co metallic standard.
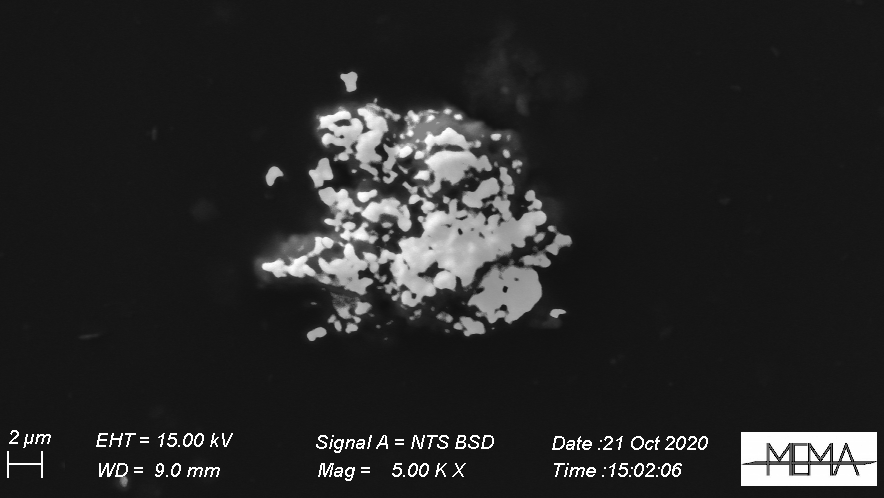
3. Conclusions
PBM characterization is of fundamental importance for human health, especially in those contexts, like workplaces, where gaseous forms are not the only airborne Hg species. In addition, it should be noted that the legislative workplace exposure limits often refer to all inorganic Hg compounds; PBM is, therefore, included in this definition and should be quantified
[8].
This entry is adapted from the peer-reviewed paper 10.3390/toxics9060141