Note:All the information in this draft can be edited by authors. And the entry will be online only after authors edit and submit it.
1. Introduction
Depression is currently ranked fourth among the causes of disability worldwide [1]. Every fifth person in developed countries has been subject to a depressive disorder at some point, and the situation is even worse in third world countries. Depression is the most common type of psychiatric disorders today, making antidepressants some of the most commonly prescribed drugs [2,3]. According to the diagnostic and Statistical Manual of Mental disorders (DSM-5), major depressive disorder (MDD) is diagnosed sustaining the following symptoms for at least two weeks: depressed mood, excessive guilt, anhedonia, suicidal ideation, changes in appetite and sleep, psychomotor retardation, poor concentration, and fatigue. MDD is a complex and heterogeneous psychiatric disorder and no reliable biomarkers currently exist that have contributed to objective diagnoses and clinical therapy [4].
The known mechanisms of origin, development, and maintenance of a depressive state are multifactorial and are determined by polymodal changes in the metabolic, immune, endocrine, gastrointestinal, and central nervous systems (CNS) [5]. The gastrointestinal tract with its microbiota interacting with external environmental signals, stress, nutrients, internal systems, including the brain, may be involved in the development of depressive states too [6]. There is ample evidence for an association between the GM and the pathophysiology of depression [7]. Different stress (social or emotional, chemical, physical, a poor diet, etc.) have the potential to alter the taxonomic composition of bacterial communities in the gut, and, as a result, leads to changes in various metabolic pathways [8]. This leads to a systematic inflammatory process, which covers the other systems and organs of humans. Over the last decade, many literature reviews have described the relationship between the gut microbiome and depression, each from a slightly different perspective [9–12]. At the current level of research, the most important point is to identify various metabolite biomarkers that correlate with depressive states. Metabolic syndrome is well-documented in MDD patients and 1.5 times higher than in the non-depressed population [13]. Isolating those that can be determined directly or indirectly by the microbiome is of great importance, both for creating diagnostic systems for identifying depression as a disease, and for choosing strategies aimed at restoring the normal functioning of the microbiota and, through it, restoring human mental health.
2. Role of the Gut Microbiome in the Gut-Brain Axis
The gut microbiota is conceptualized as a virtual organ playing a key role in maintaining homeostasis and health in humans. The GM consists predominantly of bacteria which outnumber archaea, fungi, and viruses inhabiting the intestines by a ratio of 10:1 [14]. The collective genetic makeup of the GM contains 150 times as many genes as the human genome, thereby expanding the natural capacity of humans [15]. Numerous current studies of the GM revealed its active involvement in the bidirectional communication between the gut and the brain. The discovery of the microbiota-gut-brain axis (MGBA) induced scientists to study the role of the GM to neurological health [16–18]. Clinical and experimental findings are evident of the interactions occurring locally between the GM and the intestinal cells or the enteric nervous system (ENS), and also between this microbial community and the brain via neuroendocrine and metabolic pathways. The human GM is capable of producing hundreds of metabolites that directly affect most systems and organs, including the intestinal epithelium and the enteric nervous system, and also the brain [19].
The role of the intestinal symbionts in the development and function of the brain was studied in different animal models [20]. The obtained data demonstrate the enormous effect the commensals have on a wide range of behavioral aspects, including social behavior, mood, and anxiety. MGBA covers a wide range of immune and endocrine functions [21,22] and is a key player in the initial stage of central nervous system (CNS) development in humans [23]. Disturbances in the harmonious interactions between the microbiota and its host during critical stages of child’s development can cause profound damage to gut-brain signaling pathways and put an individual at risk for psychiatric disorders later in life, including depression [16,24]. For instance, among the factors contributing to brain diseases is early life exposure to harmful food derivatives and small molecules derived from microbial metabolism, which first permeate into the bloodstream and then to the brain [25]. Thus, the identification of damaging early life events can help establish the causes of depression while diagnosing a patient. These include the exposure to antibiotics, unbalanced nutrition, stress, and other factors [26].
So far, a few major mechanisms mediating the effects of the GM on the brain have been identified. Among these are the activation of the vagus nerve [27–29] and the immune system [30,31], production of metabolites, and compounds with neuroactive properties [32,33]. Bacterial products participate in stimulation of central receptors, peripheral stimulation of neural, endocrine, and immune mediators, and epigenetic regulation of histone acetylation and DNA methylation, which are implicated in depression. (Figure 1).
The mechanisms found to be linked to depression also include a dysfunctional hypothalamic–pituitary–adrenal (HPA) axis [34]; immune-inflammatory, oxidative pathways [35]; altered vagus nerve tone [36]; region-specific changes in brain-derived neurotrophic factor concentrations [37]; and an imbalance between neural excitation and inhibitory signaling [38].
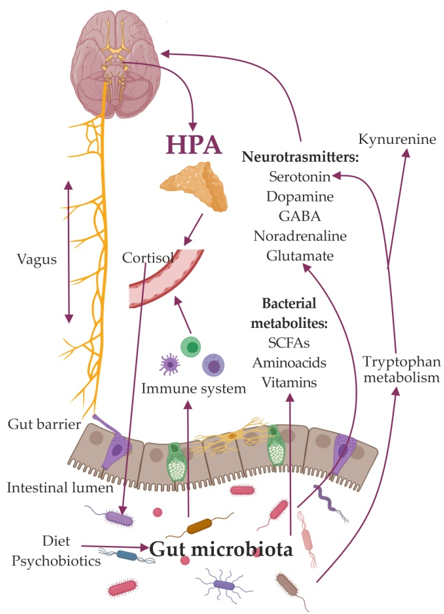
Figure 1. Schematic image of the role of the gut microbiome in the gut-brain axis.
Gut microbes may trigger neurotransmitter release via Toll-Like Receptor (TLR) signaling on epithelial, immune, and neuronal cells [39,40]. Bacteria can synthesize neuromodulators, stimulated specific epithelial cells of the intestines, to secrete molecules responsible for signal transmission through the enteric nervous system [31,32]. Using their own signaling pathways, they regulate the release of neurotransmitters from enterochromaffin cells [41–44]. Bacteria are called vectors because they can deliver neuroactive compounds to the receptors of epithelial cells. These bacteria-derived neuroactive compounds can play a significant role in modulating the signals of the GM caused by changes in concentration of ions (K+, N+, Ca2+, Cl−, etc.), as well as exogenous agonists and antagonists coming from food. Thus, bacterial neuroactive substances can control depolarization in the synaptic area of neurons containing neurotransmitters. Bacteria can produce the neurotransmitters GABA, serotonin, dopamine, and acetylcholine [44,45], which can affect the emotional state by binding to specific receptors on nerve and immune cells in the central and peripheral nervous systems [8].
Elucidating these mechanisms is crucial for understanding the etiology of depression and developing new strategies aimed at harnessing the beneficial psychotropic effects of these molecules. It is also important to note that not all bacterial metabolites are beneficial. Some metabolites are rather harmful and conducive to depression.
References
- Friedrich, J. Depression is the leading cause of disability around the world. JAMA 2017, 317, 1517.
- Alduhishy, The overprescription of antidepressants and its impact on the elderly in Australia. Trends Psychiatry Psychother. 2018, 40, 241–243.
- Olfson, ; Marcus, S.C. National patterns in antidepressant medication treatment. Arch. Gen. Psychiatry 2009, 66, 848–856.
- Otte, ; Gold, S.M.; Penninx, B.W.; Pariante, C.M.; Etkin, A.; Fava, M.; Mohr, D.C.; Schatzberg, A.F. Major depressive disorder. Nat. Rev. Dis. Primers 2016, 2, 1–20.
- Stefano, ; Pilonis, N.; Ptacek, R.; Raboch, J.; Vnukova, M.; Kream, R. Gut, microbiome, and brain regulatory axis: Relevance to neurodegenerative and psychiatric disorders. Cell. Mol. Neurobiol. 2018, 38, 1197–1206.
- Makris, A.P.; Karianaki, M.; Tsamis, K. I. The role of the gut-brain axis in depression: endocrine, neural, and immune pathways. Hormones 2020, 1-12.
- Karl, P.; Hatch, A.M.; Arcidiacono, S.M.; Pearce, S.C.; Pantoja-Feliciano, I.G.; Doherty, L.A.; Soares, J.W. Effects of psychological, environmental and physical stressors on the gut microbiota. Front. Microbiol. 2018, 9, 2013.
- Caspani, ; Kennedy, S.; Foster, J.A.; Swann, J. Gut microbial metabolites in depression: Understanding the biochemical mechanisms. Microb. Cell 2019, 6, 454.
- Therese, L.; Khan, F.; Eskander, N. Gut Microbiome and Depression: How Microbes Affect the Way We Think. Cureus 2020, 12, 8.
- Capuco, ; Urits, I.; Hasoon, J.; Chun, R.; Gerald, B.; Wang, J.K.; Kassem, H.; Ngo, A.L.; Abd-Elsayed, A.; Simopoulos, T. Current perspectives on gut microbiome dysbiosis and depression. Adv. Ther. 2020, 37, 1328–1346.
- Bear, L.; Dalziel, J.E.; Coad, J.; Roy, N.C.; Butts, C.A.; Gopal, P.K. The Role of the Gut Microbiota in Dietary Interventions for Depression and Anxiety. Adv. Nutr. 2020, 11, 890–907.
- Bastiaanssen, F.; Cussotto, S.; Claesson, M.J.; Clarke, G.; Dinan, T.G.; Cryan, J.F. Gutted! Unraveling the role of the microbiome in major depressive disorder. Harv. Rev. Psychiatry 2020, 28, 26–39.
- Gheshlagh, G.; Parizad, N.; Sayehmiri, K. The relationship between depression and metabolic syndrome: Systematic review and meta-analysis study. Iran. Red Crescent Med. J. 2016, 18, e26523.
- Marchesi, R. Prokaryotic and eukaryotic diversity of the human gut. In Advances in Applied Microbiology; Elsevier: Amsterdam, The Netherlands, 2010; Volume 72, pp. 43–62.
- Qin, ; Li, R.; Raes, J.; Arumugam, M.; Burgdorf, K.S.; Manichanh, C.; Nielsen, T.; Pons, N.; Levenez, F.; Yamada, T. A human gut microbial gene catalogue established by metagenomic sequencing. Nature 2010, 464, 59–65.
- Borre, E.; Moloney, R.D.; Clarke, G.; Dinan, T.G.; Cryan, J.F. The impact of microbiota on brain and behavior: Mechanisms & therapeutic potential. In Microbial Endocrinology: The Microbiota-Gut-Brain Axis in Health and Disease; Springer: Berlin/Heidelberg, Germany, 2014; pp. 373–403.
- Wang, ; Kasper, L.H. The role of microbiome in central nervous system disorders. BrainBehav. Immun. 2014, 38, 1–12.
- Fetissov, O.; Averina, O.V.; Danilenko, V.N. Neuropeptides in the microbiota-brain axis and feeding behavior in autism spectrum disorder. Nutrition 2019, 61, 43–48.
- Brown, M.; Hazen, S.L. The gut microbial endocrine organ: Bacterially derived signals driving cardiometabolic diseases. Annu. Rev. Med. 2015, 66, 343–359.
- Luczynski, ; Whelan, S.O.; O’Sullivan, C.; Clarke, G.; Shanahan, F.; Dinan, T.G.; Cryan, J.F. Adult microbiota-deficient mice have distinct dendritic morphological changes: Differential effects in the amygdala and hippocampus. Eur. J. Neurosci. 2016, 44, 2654–2666.
- Bravo, A.; Julio-Pieper, M.; Forsythe, P.; Kunze, W.; Dinan, T.G.; Bienenstock, J.; Cryan, J.F. Communication between gastrointestinal bacteria and the nervous system. Curr. Opin. Pharmacol. 2012, 12, 667–672.
- Stilling, ; Dinan, T.; Cryan, J. Microbes do have a significant impact on epigenetic regulation in the host’s gut epithelium and immune system, microbial genes, brain and behaviour-epigenetic regulation of the gut-brain axis. Genes Brain Behav. 2014, 13, 69–86
- Sampson, R.; Mazmanian, S.K. Control of brain development, function, and behavior by the microbiome. Cell Host Microbe 2015, 17, 565–576.
- Averina, V.; Kovtun, A.S.; Polyakova, S.I.; Savilova, A.M.; Rebrikov, D.V.; Danilenko, V.N. The bacterial neurometabolic signature of the gut microbiota of young children with autism spectrum disorders. J. Med. Microbiol. 2020, 69, 558–571.
- Forgie, J.; Drall, K.M.; Bourque, S.L.; Field, C.J.; Kozyrskyj, A.L.; Willing, B.P. The impact of maternal and early life malnutrition on health: A diet-microbe perspective. BMC Med. 2020, 18, 1–15.
- Kovtun, S.; Averina, O.V.; Alekseeva, M.G.; Danilenko, V.N. Antibiotic resistance genes in the gut microbiota of children with autistic spectrum disorder as possible predictors of the disease. Microb. Drug Resist. 2020, 26, 1307–1320.
- Wang, ; Wang, B.-R.; Zhang, X.-J.; Xu, Z.; Ding, Y.-Q.; Ju, G. Evidences for vagus nerve in maintenance of immune balance and transmission of immune information from gut to brain in STM-infected rats. World J. Gastroenterol. 2002, 8, 540.
- Wang, ; Yu, M.; Ochani, M.; Amella, C.A.; Tanovic, M.; Susarla, S.; Li, J.H.; Wang, H.; Yang, H.; Ulloa, L. Nicotinic acetylcholine receptor α7 subunit is an essential regulator of inflammation. Nature 2003, 421, 384–388.
- De Lartigue, ; de La Serre, C.B.; Raybould, H.E. Vagal afferent neurons in high fat diet-induced obesity; intestinal microflora, gut inflammation and cholecystokinin. Physiol. Behav. 2011, 105, 100–105.
- Sternberg, M. Neural regulation of innate immunity: A coordinated nonspecific host response to pathogens. Nat. Rev. Immunol. 2006, 6, 318–328.
- Dantzer, ; O’Connor, J.C.; Freund, G.G.; Johnson, R.W.; Kelley, K.W. From inflammation to sickness and depression: When the immune system subjugates the brain. Nat. Rev. Neurosci. 2008, 9, 46–56.
- MacFabe, F.; Cain, N.E.; Boon, F.; Ossenkopp, K.-P.; Cain, D.P. Effects of the enteric bacterial metabolic product propionic acid on object-directed behavior, social behavior, cognition, and neuroinflammation in adolescent rats: Relevance to autism spectrum disorder. Behav. Brain Res. 2011, 217, 47–54.
- Thomas, M.; Hong, T.; Van Pijkeren, J.P.; Hemarajata, P.; Trinh, D.V.; Hu, W.; Britton, R.A.; Kalkum, M.; Versalovic, J. Histamine derived from probiotic Lactobacillus reuteri suppresses TNF via modulation of PKA and ERK signaling. PLoS ONE 2012, 7, e31951.
- Burke, M.; Davis, M.C.; Otte, C.; Mohr, D.C. Depression and cortisol responses to psychological stress: A meta-analysis. Psychoneuroendocrinology 2005, 30, 846–856.
- Köhler, ; Freitas, T.; Maes, M.D.; De Andrade, N.; Liu, C.; Fernandes, B.; Stubbs, B.; Solmi, M.; Veronese, N.; Herrmann, N. Peripheral cytokine and chemokine alterations in depression: A meta-analysis of 82 studies. Acta Psychiatr. Scand. 2017, 135, 373–387.
- Chambers, S.; Allen, J.J. Vagal tone as an indicator of treatment response in major depression. Psychophysiology 2002, 39, 861–864.
- Krishnan, ; Nestler, E.J. The molecular neurobiology of depression. Nature 2008, 455, 894–902.
- Ghosal, ; Hare, B.D.; Duman, R.S. Prefrontal cortex GABAergic deficits and circuit dysfunction in the pathophysiology and treatment of chronic stress and depression. Curr. Opin. Behav. Sci. 2017, 14, 1–8.
- Barajon, ; Serrao, G.; Arnaboldi, F.; Opizzi, E.; Ripamonti, G.; Balsari, A.; Rumio, C. Toll-like receptors 3, 4, and 7 are expressed in the enteric nervous system and dorsal root ganglia. J. Histochem. Cytochem. 2009, 57, 1013–1023.
- Brun, ; Giron, M.C.; Qesari, M.; Porzionato, A.; Caputi, V.; Zoppellaro, C.; Banzato, S.; Grillo, A.R.; Spagnol, L.; De Caro, R. Toll-like receptor 2 regulates intestinal inflammation by controlling integrity of the enteric nervous system. Gastroenterology 2013, 145, 1323–1333.
- Grenham, ; Clarke, G.; Cryan, J.F.; Dinan, T.G. Brain–gut–microbe communication in health and disease. Front. Physiol. 2011, 2, 94.
- Khanna, ; Tosh, P.K. A clinician’s primer on the role of the microbiome in human health and disease. In Mayo Clinic Proceedings; Elsevier: Amsterdam, The Netherlands, 2014; pp. 107–114.
- Yano, M.; Yu, K.; Donaldson, G.P.; Shastri, G.G.; Ann, P.; Ma, L.; Nagler, C.R.; Ismagilov, R.F.; Mazmanian, S.K.; Hsiao, E.Y. Indigenous bacteria from the gut microbiota regulate host serotonin biosynthesis. Cell 2015, 161, 264–276.
- Lyte, ; Cryan, J.F. Microbial Endocrinology: The Microbiota-Gut-Brain Axis in Health and Disease; Springer: Berlin/Heidelberg, Germany, 2014; Volume 817.
- Averina, V.; Danilenko, V.N. Human intestinal microbiota: Role in development and functioning of the nervous system. Microbiology 2017, 86, 1–18.
This entry is adapted from the peer-reviewed paper 10.3390/ijms21239234